Fig. 9.1
Representative findings of 123I-IMZ SEPCT. White-and-black images of the vehicle- (a) and bone marrow stromal cell (BMSC)-transplanted rats (b). In both vehicle- and BMSC-treated rats, a marked decrease in the uptake of 123I-IMZ is observed in the ipsilateral neocortex at 6 days postischemia. The uptake of 123I-IMZ significantly improves in the peri-infarct neocortex of the BMSC-transplanted rats at 35 days postischemia (arrow). The finding cannot be observed in the vehicle-transplanted rats [14]
9.2.2 Effects of Cell Therapy on Glucose Metabolism
Using an autoradiography technique, Mori et al. found that BMSC transplantation significantly improves glucose metabolism in the thalamus and barrel cortex in response to whisker stimulation after neocortical freezing injury [12]. The study by Dr. Wang et al. demonstrated functional recovery in a rat stroke model treated by intraventricularly administered ESCs and iPSCs along with increased 18F-FDG uptake in stroke lesions depicted by small-animal PET and autoradiography. Immunohistochemistry examination at 4 weeks after cell transplantation also indicated the presence of neuronal differentiation among injected cells [44, 45]. Du et al. reported that the BMSCs delivered via the intra-arterial route promoted angiogenesis and improved functional recovery in a rat transient MCA occlusion model, using 99mTc-ECD SPECT and 18F-FDG PET [46]. We also serially assessed local glucose metabolism in the rats subjected to permanent MCA occlusion and found that BMSC transplantation significantly enhances the recovery in the peri-infarct area, using small-animal 18F-FDG PET/CT system. As shown in Fig. 9.2, glucose utilization was markedly decreased in the ipsilateral neocortex at 6 days after ischemia. In the vehicle-treated animals, glucose utilization improved to some extent in the peri-infarct neocortex at 35 days after ischemia, that is, 28 days after transplantation. However, BMSC transplantation significantly enhanced the recovery in the peri-infarct neocortex at the same time point [5]. Considering together with 123I-IMZ study, the BMSCs may enhance the recovery of local glucose metabolism by improving neuronal integrity in the peri-infarct area, when directly transplanted into the infarct brain, because oxidative glucose metabolism is quite high in the neurons. Therefore, BMSC transplantation may possibly contribute to accelerate functional recovery by improving neuronal integrity and local metabolism in the peri-infarct brain. However, some alternative possibilities are not completely excluded. The increase in 18F-FDG uptake might indicate not only neural but also glial proliferation by cell therapy, neovascular growth facilitated by treatment, or a simple reflection of macrophage migration and microglial activation after ischemia. Although the mechanisms of 18F-FDG uptake in the stem cell-treated lesions are still not clear, the uptake and functional recovery measured by behavioral testing appear to correlate in some studies [45].


Fig. 9.2
Representative findings of 18F-FDG PET at 6 and 35 days after ischemia. Color (a) and black-and-white (c) images of vehicle-transplanted animals. Color (b) and black-and-white (d) images of BMSC-transplanted rats. There was significant increase in local glucose metabolism in peri-infarct neocortex (arrows) [5]
9.3 Conclusion
It would be essential to establish functional bio-imaging technique serially and noninvasively validating the effects of cell therapy on the host CNS in order to achieve clinical application of cell therapy for cerebral stroke. Although there are few studies that indicate the utility of imaging techniques to monitor the response of the host CNS after cell therapy and further investigation is needed, 123I-IMZ SPECT and 18F-FDG PET may be promising modalities to assess the therapeutic benefits of cell therapy for ischemic stroke without subjective bias in clinical situation.
References
1.
Savitz SI, Fisher M. Future of neuroprotection for acute stroke: in the aftermath of the SAINT trials. Ann Neurol. 2007;61(5):396–402. Epub 2007/04/11. eng.PubMed
2.
Jablonska A, Lukomska B. Stroke induced brain changes: implications for stem cell transplantation. Acta Neurobiol Exp. 2011;71(1):74–85.
3.
Hokari M, Kuroda S, Shichinohe H, Yano S, Hida K, Iwasaki Y. Bone marrow stromal cells protect and repair damaged neurons through multiple mechanisms. J Neurosci Res. 2008;86(5):1024–35.PubMed
4.
Kawabori M, Kuroda S, Ito M, Shichinohe H, Houkin K, Kuge Y, et al. Timing and cell dose determine therapeutic effects of bone marrow stromal cell transplantation in rat model of cerebral infarct. Neuropathology. 2013;33(2):140–8.PubMed
5.
Miyamoto M, Kuroda S, Zhao S, Magota K, Shichinohe H, Houkin K, et al. Bone marrow stromal cell transplantation enhances recovery of local glucose metabolism after cerebral infarction in rats: a serial 18F-FDG PET study. J Nucl Med. 2013;54(1):145–50.PubMed
6.
Shichinohe H, Kuroda S, Yano S, Ohnishi T, Tamagami H, Hida K, et al. Improved expression of gamma-aminobutyric acid receptor in mice with cerebral infarct and transplanted bone marrow stromal cells: an autoradiographic and histologic analysis. J Nucl Med. 2006;47(3):486–91.PubMed
7.
Sugiyama T, Kuroda S, Takeda Y, Nishio M, Ito M, Shichinohe H, et al. Therapeutic impact of human bone marrow stromal cells expanded by animal serum-free medium for cerebral infarct in rats. Neurosurgery. 2011;68(6):1733–42. discussion 42.PubMed
8.
Lee JS, Hong JM, Moon GJ, Lee PH, Ahn YH, Bang OY, et al. A long-term follow-up study of intravenous autologous mesenchymal stem cell transplantation in patients with ischemic stroke. Stem Cells. 2010;28(6):1099–106.PubMed
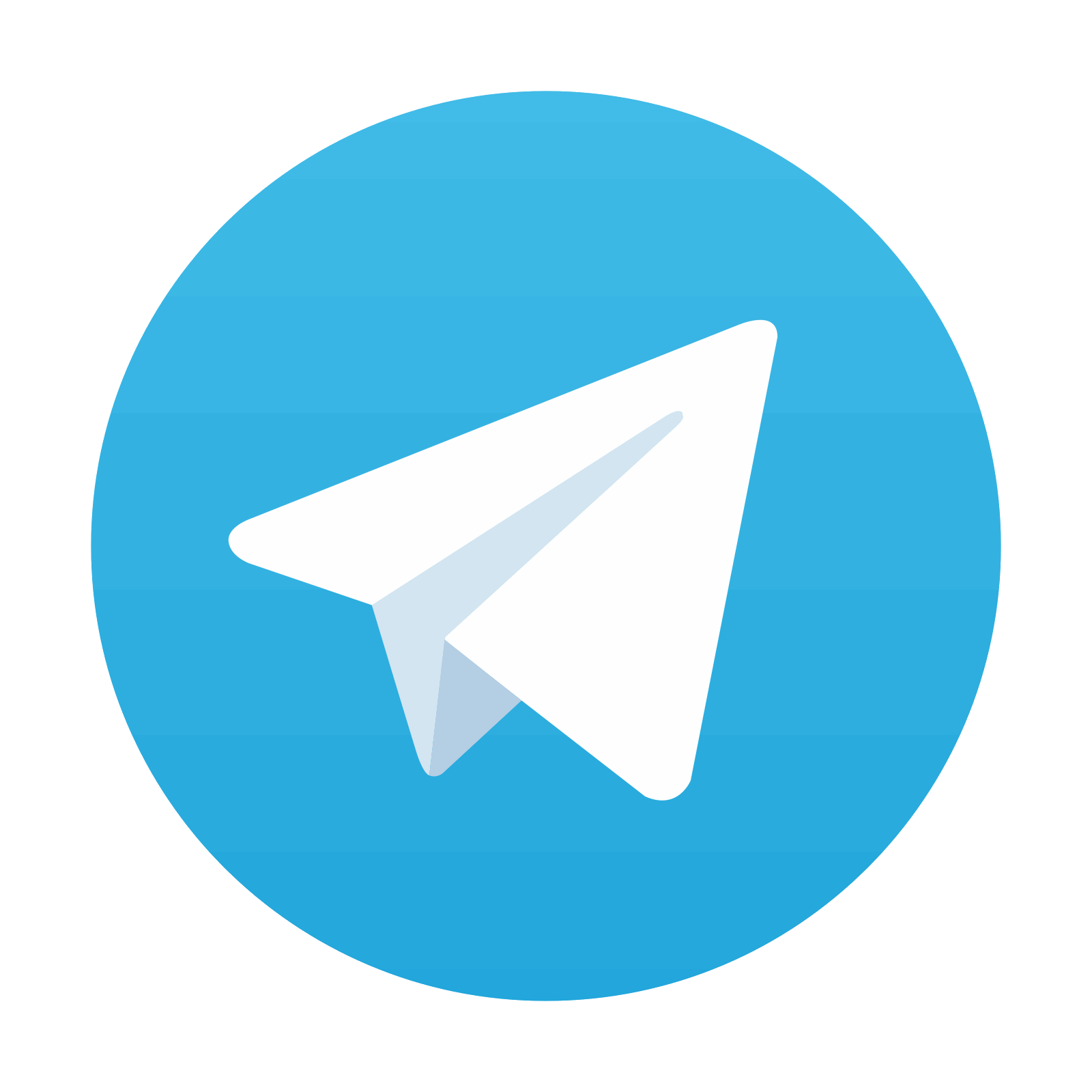
Stay updated, free articles. Join our Telegram channel

Full access? Get Clinical Tree
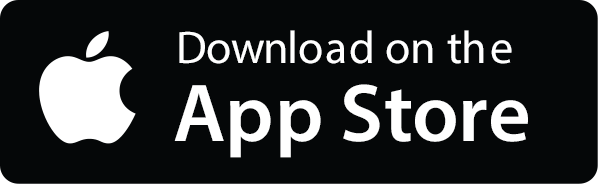
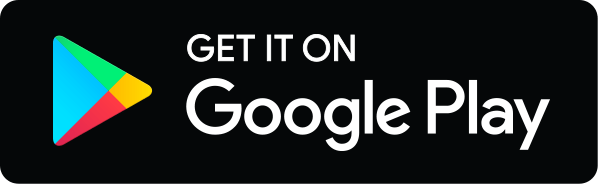