© Springer International Publishing Switzerland 2017
James M. Ecklund and Leon E. Moores (eds.)Neurotrauma Management for the Severely Injured Polytrauma Patient10.1007/978-3-319-40208-6_2828. Functional Restoration for Neurological Trauma: Current Therapies and Future Directions
(1)
Dept. of Neurosciences, Inova Fairfax Hospital, Virginia Commonwealth University School of Medicine—Inova Campus, 3300 Gallows Road North Patient Tower, 2nd Floor, Falls Church, VA 22042, USA
Keywords
NeuromodulationVagal nerve stimulatorEpilepsyResponsive neurostimulatorDeep brain stimulationSpinal cord stimulatorMemoryCognitionSpasticityIntrathecal baclofen pumpExoskeletonBrain–machine interfaceBrain injurySpinal cord injuryFunctional Restoration for Neurological Trauma: Current Therapies and Future Directions
Neurological trauma is frequently associated with disruptive or disabling neurological symptoms and deficits. The annual incidence of traumatic brain injury in the United States has been estimated at 1.5 million with approximately 300,000 hospitalizations and over 50,000 deaths [1–4]. It has been estimated that these traumatic brain injuries lead to over 120,000 people with long-term disability annually [2], resulting in 1.1 % of the US population with disability from traumatic brain injury [5]. Traumatic brain injury is associated with posttraumatic epilepsy [6–10], impaired attention, memory and concentration [11–13], disabling neurological symptoms such as headache, dizziness, sleep disturbance, tinnitus and imbalance [11], emotional problems including depression, anxiety and posttraumatic stress disorder [11, 12, 14], social impacts including difficulties with school, employment, and marriage [11, 14], and in the most extreme conditions spasticity , minimally conscious states and persistent vegetative states.
Spinal cord injury affects an estimated 273,000 people per year in the United States [15]. Spinal cord injury frequently results in loss or limits in the use of the extremities as well as spasticity and pain [16–18]. The prevalence of peripheral nerve injury in one series was 2.8 % within the trauma population [19], with a 1.64 % incidence among patients with extremity trauma [20]. Peripheral nerve injuries can result in loss of motor and/or sensory function and can also lead to development of complex regional pain syndrome [21, 22]. These effects of neurotrauma can be mitigated by modern neuromodulation surgical techniques and devices. This chapter will review the neuromodulation techniques currently part of standard practice as well as those in development and potential future technologies that may provide alleviation of the negative sequelae of neurotrauma.
Posttraumatic Epilepsy
Head trauma is associated with an incidence of posttraumatic epilepsy to a degree commensurate with the severity of the head trauma. Seizures following head injury are classified as early posttraumatic seizures (EPTS) which occur within the first week following the head injury and late posttraumatic seizures (LPTS) which occur at any time later than 1 week following head trauma [6]. Studies have shown the incidence of EPTS in adults (>16 years of age) with severe traumatic brain injury to be 8.4 %, and in children to be proportional to the severity of injury with moderate to severe traumatic brain injury resulting in 12 % EPTS [23] and severe traumatic brain injury resulting in 19–25 % EPTS [6, 24]. EPTS are associated with a higher incidence of LPTS and posttraumatic epilepsy [6]. LPTS and posttraumatic epilepsy also appear to occur more frequently in more severe traumatic brain injury. The incidence of LPTS was 2.1 % in all types of traumatic brain injuries [8]. 10 % in patients with traumatic brain injuries having positive head CT findings [7], and 25.3 % in patients with traumatic brain injuries severe enough to require rehabilitation services [6]. In one study, 77 % of patients having LPTS went on to develop posttraumatic epilepsy [8]. The incidence of posttraumatic epilepsy is the highest in penetrating head trauma with 53 % of patients having posttraumatic seizures of which 92 % went on to have posttraumatic epilepsy [9].
Posttraumatic epilepsy has significant impacts on many aspects of life. Patients with LPTS have a higher disability rating, lower life satisfaction scores, and have a higher utilization of public transportation due to inability to drive compared to traumatic brain injury patients without LPTS [25]. In a study comparing mortality rates of traumatic brain injury patients over a 8–15 year post-injury period 27 % of LPTS patients died compared to 10 % of those without LPTS [26]. Clearly, interventions to prevent the development of posttraumatic epilepsy and prevent seizures in patients who do develop posttraumatic epilepsy would positively impact clinical outcomes. Anti-seizure medications have demonstrated efficacy in reducing the incidence of EPTS [23, 27] but no medication has proven effective in preventing posttraumatic epilepsy [27–29]. A proposed trial of levetiracetam for prevention of posttraumatic epilepsy may hold promise but has not yet yielded results [30–32].
Once posttraumatic epilepsy has developed, surgical approaches to seizure control have proven effective. A review of all extratemporal lobe resections for posttraumatic epilepsy at a single institution showed a 28 % seizure free rate [33], but another study demonstrated higher seizure free rates for posttraumatic frontal lobe epilepsy surgery at 33 % and posttraumatic temporal lobe epilepsy surgery at 69 % [34]. These outcomes are similar to outcomes for epilepsy surgery of non-traumatic origin as well [35, 36]. The surgically implanted vagal nerve stimulator has also been effective in control of posttraumatic epilepsy. Although few patients are seizure free with the vagal nerve stimulator, 4.6 % in a review of studies [37], the majority of patients benefit from the therapy with 50–56 % of patients having greater than 50 % reduction in their seizures [37, 38] and an average reduction in seizures of 62–73 % at 2 years [38, 39]. Other promising surgical techniques for seizure control include the Neuropace RNS device which was recently approved by the US Food and Drug Administration (FDA). This device provided 54 % of patients greater than 50 % seizure reduction [40]. The medtronic deep brain stimulator has been effective in reducing seizures by stimulation of the anterior nucleus of the thalamus. This therapy is not yet FDA approved but is being used internationally and has demonstrated a 50 % or greater reduction in seizures in 54 % of patients [41].
In animal studies vagal nerve stimulation has demonstrated a positive impact on other aspects of trauma. In animal models of brain injury, vagal nerve stimulation was able to decrease edema and the inflammatory response [42, 43], decrease disruption of the blood–brain barrier [44], and protect GABAergic neurons [45]. Vagal nerve stimulation in animal models of brain injury has also produced improvements in behavioral outcomes with improved performance on motor tasks [43, 46–48] and a cognitive task [48]. Although these results are promising, vagal nerve stimulation is not currently being utilized in humans for traumatic brain injury therapy other than for the treatment of posttraumatic epilepsy .
Memory , Cognition and Consciousness
The detrimental effects of traumatic brain injury on memory and cognition, including the effects on concentration necessary for memory and cognition, are frequently transient but can lead to significant disability when they are persistent. In one study, 22 % of traumatic brain injury patients reported persistent memory or thinking problems [11]. The effects of traumatic brain injury on neuropsychological tests of memory and cognition seem to be dependent on severity of the brain injury. Patients with mild traumatic brain injury had impairments in pooled neuropsychological testing compared to controls. Their average results on the various tests were 13–24 % below the average results of the control subjects [49]. Mild traumatic brain injury patients appear to have greater impairments in attention where moderate to severe traumatic brain injury patients have greater deficits in memory and learning [13]. Severely brain injured patients have shown more cognitive difficulties than mild to moderate brain injured patients [12] and decreases in neuropsychological testing performance correlate with higher levels of injury severity on the Glasgow Outcome Scale in moderate to severe brain injury patients [14]. One to 55 % of patients with mild to severe brain injuries had moderate to severe cognitive impairment on a battery of neuropsychological tests [50]. A review of the neuropsychology of brain injury literature suggested a dose dependent effect of brain injury on cognitive impairment with a clear decline of cognitive functions following penetrating brain injury [51].
Brain stimulation may hold promise as a therapy for the treatment of cognitive deficits and may play a positive role in the treatment of cognitive deficits due to traumatic brain injury. Deep brain stimulation is most widely utilized for the treatment of movement disorders and has proven effective in the treatment of tremor related to traumatic brain injury [52, 53]. Several animal models of traumatic brain injury have had improvements in the resulting memory deficits with deep brain stimulation of the hippocampus [54, 55], fornix [56], and the medial septal nucleus [57]. A few cases of deep brain stimulation for the treatment of memory deficits or improvement of baseline memory have been conducted in humans, but mostly in dementia patients, not trauma patients with cognitive deficits. The oldest of these is a case report of stimulation of the basal nucleus of Meynert in a patient with Alzheimer’s dementia. The stimulation produced no significant clinical improvement [58]. Memory improvement from stimulation of the fornix and hypothalamic area in a person with normal cognition initially prompted enthusiasm for this target as a treatment of dementia [59]. Deep brain stimulation of the fornix and hypothalamus region in a small group of patients with dementia produced some improvement in memory or slowing of cognitive decline [60, 61], but the effects have been mild. More recently, visual-spatial memory improvements but not improvements in verbal or naming memory were demonstrated with stimulation of the fornix in patients without dementia being monitored for medically refractory epilepsy [62]. A study of nucleus accumbens deep brain stimulation in patients with treatment-resistant depression suggested a trend toward cognitive improvement [63]. However, a review of the literature concerning deep brain stimulation as a treatment of Alzheimer’s disease warned that the data is preliminary and limited [64], making it far from use in other pathologies with memory deficits. Noninvasive brain stimulation techniques have also had some limited success. Repetitive transcranial magnetic stimulation of the left dorsolateral prefrontal cortex improved outcomes in several neuropsychiatric measures and significantly decreased post-concussive symptoms in patients with mild traumatic brain injury [65]. The United States Defense Advanced Research Projects Agency has invested in an ambitious project to develop a memory prosthesis device [66, 67] so a neuromodulation device for the treatment of posttraumatic brain injury memory deficits may be available to patients in the near future.
The more significant effects of severe brain injury on consciousness are always disabling when they persist. They lead to significant health care costs as well as emotional distress for the patients and their families. In one hospital’s experience, 0.6 % of all patients admitted with head injury had prolonged unconsciousness for over two weeks [68]. In a population study of traumatic brain injury it was estimated that 0.3 % remained in a persistent vegetative state and 0.8 % remained in a minimally conscious state 1 year after the head trauma [69]. Another study of severe traumatic brain injury reported 7.2 % remained in a persistent vegetative state and 2.4 % were in a minimally conscious state [70]. The persistent vegetative state has been defined as a complete lack of awareness of self and environment with no purposeful or voluntary response to stimuli but with preserved sleep-wake cycles, hypothalamic function, and brainstem autonomic functions [71, 72]. In the minimally conscious state the patient is able to follow simple commands, respond yes or no verbally or with gestures, have intelligible verbalization and/or have purposeful behavior without achieving functional interactive communication or the use of two or more different objects [73]. The prevalence of persistent vegetative state has been estimated at 0.2–6.1 patients per 100,000 population [74], which would be approximately 640–19,500 in the United States alone. The average lifetime cost of care per patient with severe traumatic brain injury has been estimated at $600,000–$1,875,000 [4], with those in a persistent vegetative or minimally conscious state being at the higher end of these costs. The humanitarian and economic impact of any intervention that could improve the level of consciousness in these patients would be significant.
There have been reports of improved levels of consciousness in patients in a permanent vegetative state and minimally conscious state with brain stimulation. The foundation for brain stimulation is found in the results from animal model studies. Deep brain stimulation of the thalamus [55, 75, 76] and hippocampus [75] in animal models of traumatic brain injury have demonstrated increases in measures of arousal. A group of clinical investigators in Japan has produced several reports of positive outcomes with over a 10 year follow up in a group of 21 patients in a persistent vegetative state who received deep brain stimulation of the mesencephalic reticular formation or the centromedian parafasicular complex of the thalamus [77–82]. They report that 8 of the 21 patients had improved consciousness to the level of being able to follow commands. These results have not been replicated by others in the literature. The same group reports positive results from the use of deep brain stimulation and spinal cord stimulation in patients in a minimally conscious state [80, 83]. All five of the patients receiving deep brain stimulation had stimulation of the centromedian parafasicular complex of the thalamus and the ten spinal cord stimulator patients had electrodes placed in the epidural space from C2 to C4. They report that all 5 of the deep brain stimulator patients and 7 of the 10 spinal cord stimulator patients emerged from the minimally conscious state to be able to functionally use two different items and have functionally interactive communication. Deep brain stimulation of the central thalamus has been attempted by others for the treatment of patients in a minimally conscious state. In one case report [84] and a report of single-subject studies which appears to present the same data as the case report [85] a group of investigators describe increases in measures of arousal, communication, and motor function with deep brain stimulation of the central thalamus of patients in a minimally conscious state. However, with limited data it is difficult at this point to determine how successful this therapy may be.
The less invasive techniques of transcranial stimulation have also been attempted in these two patient populations. In a randomized double-blind placebo-controlled crossover study of transcranial direct current stimulation of the left dorsolateral prefrontal cortex, the group of 30 patients in a minimally conscious state showed benefit in a measure of coma, but a group of 25 patients in a persistent vegetative state did not [86]. In another study of transcranial direct current stimulation of the left dorsolateral prefrontal cortex or the left primary sensorimotor cortex none of the seven patients in a persistent vegetative state improved in a measure of coma but all three of the patients in a minimally conscious state had improvements [87]. A case report of transcranial magnetic stimulation for a patient in a minimally conscious state reported an increase in meaningful behaviors interpreted as a clinical benefit [88]. These results hold promise for a minimally invasive therapy for the minimally conscious patient population. Another less invasive surgical option has been considered for the treatment of traumatic brain injury patients with disorders of consciousness as well. Given the encouraging basic science results of vagal nerve stimulation in animal models of traumatic brain injury [42–48], a study of vagal nerve stimulation in patients with traumatic brain injury and persistently vegetative or minimally conscious states has been proposed [89].
Depression
Major depressive disorder remains a significant problem in patients with traumatic brain injury. Among all traumatic brain injury patients 22 % self-report feelings of depression [11], whereas 33–53 % of traumatic brain injury patients fulfill objective criteria for major depressive disorder within the first year after injury [90, 91]. Post-concussive disorder is highly associated with the development of depression [92]. The development of depression also seems to be relative to the severity of the traumatic brain injury with 3.6–11.6 % of mild traumatic brain injury patients developing depression at 1 year [93, 94], and 21.2 % of severe traumatic brain injury patients developing major depressive disorder after their injury [95]. Traumatic brain injury patients who developed depression had lower health-related quality of life measures [95] and poorer functional outcomes as measured by the Glasgow Outcome Scale [93, 96]. Medical therapy and psychosocial interventions are currently employed for the treatment of traumatic brain injury-related depression, but as a recent systematic review of the literature has demonstrated [97] the success of these interventions is modest. There are only two studies with class I evidence. One is a study of sertraline in which 59 % of the treatment group and 32 % of the placebo group were responders with a 50 % or greater decrease in their baseline Hamilton Depression Rating Scale (HAM-D) scores. The other is a study of multidisciplinary psychosocial intervention that resulted in no statistically significant difference in the Hospital Anxiety and Depression Scale between the intervention and the placebo groups. The other studies showed varying degrees of improvement, but without class I evidence it is difficult to determine how successful these interventions would be in the traumatic brain injury population.
Neuromodulation techniques have been applied to general major depressive disorder without specifically targeting traumatic brain injury-related depression, but the outcomes may prove to generalize to traumatic brain injury patients as well. Deep brain stimulation has been preliminarily investigated as an intervention for the treatment of major depressive disorder. The most commonly studied deep brain stimulation target for depression is the subcallosal cingulate gyrus. The original group of 20 treatment-resistant major depressive disorder patients implanted in Toronto, Canada have reported follow-up outcomes up to 3–6 years after surgery [98–100]. Utilizing the Hamilton Rating Scale for Depression and considering patients with greater than 50 % reduction from baseline responders, at 12 months this group of 20 patients went from a mean score of 24.4 to a mean score of 12.6 [98] with a responder rate of 55 % [99]. They reported a 60 % responder rate at 3 years with a 40 % remission rate [100]. Deep brain stimulation of the subcallosal cingulate gyrus has been reported in multiple studies from seven medical centers that included a total of 56 treatment-resistant depression patients [101–105]. From combined data of these studies, at 6 months after surgery there were 28/56 (50 %) responders and 8/35 (22.9 %) remitters, at 1 year after surgery there were 16/43 (37.2 %) responders and 9/22 (40.9 %) remitters, and at 2 years after surgery there were 11/12 (91.7 %) responders and 7/12 (58.3 %) remitters (one study did not report remission rates, only response rates). Another promising deep brain stimulation site for depression is the ventral capsule/ventral striatum region which was used in 15 treatment-resistant patients with response rates of 46.7 % at 3 months, 40 % at 6 months and 53.3 % at last follow-up [106]. Deep brain stimulation of the nucleus accumbens and caudate nucleus was attempted in four treatment-resistant depression patients with no responders at 6 months but three responders at 1 year with nucleus accumbens stimulation, one of whom was in remission [107].
Spasticity
Spasticity is one of the potential sequelae of severe traumatic brain injury that can lead to discomfort, disability, and difficulty with care. In a review of patients with severe traumatic brain injury requiring rehabilitation, 25.8 % had increased muscle tone consistent with some degree of spasticity [108]. Forty percent of these patients required some intervention for their spasticity. Traditional medical treatment modalities for traumatic brain injury patients with spasticity include medications, muscle stretching, orthoses, nerve blocks, phenol neurolysis, BoTox injections [109, 110], ambulation, serial casting, electrical stimulation, and robotics [111]. Medications used orally for traumatic brain injury spasticity are baclofen, tizanidine, cannabinoids, benzodiazepines, clonidine, and dantrolene [111]. Traditional surgical therapies included surgical muscle lengthening, transfers, or releases [109], as well as rhizotomies [111].
Neuromodulation for spasticity by intrathecal infusion of baclofen provides a nondestructive surgical therapy. The standard measure used to grade spasticity is the Ashworth Scale [112] which is graded as follows; 1 no increase in tone, 2 slight increase in tone with a catch that releases, 3 more marked increase in tone but able to move extremity through range of motion, 4 significant increase in muscle tone making movement difficult, 5 rigid in flexion or extension. The traditional protocol for implantation of in intrathecal catheter and pump for long-term therapy is to begin with a trial of 50 micrograms of intrathecal baclofen to evaluate the response. One paper evaluating the intrathecal baclofen trial in a spasticity population primarily containing traumatic brain injury patients (10 out of 11) reported a decrease in the average lower extremity Ashworth scale from 4.2 to 2.2 at 4 h after administration of the baclofen [113]. Two other studies of 58 total patients, 29 of which had traumatic brain injuries, found that the average lower extremity spasticity decreased from a baseline of 2.0–2.4 to 1.6 at 2 h, 1.4–1.5 at 4 h, and 1.3–1.4 at 6 h [114, 115]. Pooled data from the traumatic brain injury patients reported in five different studies of long-term intrathecal baclofen infusion by programmable pump demonstrated clear benefit of this therapy in upper and lower extremity spasticity [116–120]. Included in these five studies were 42 patients with spasticity from traumatic brain injury who were followed anywhere from 3 months to 5 years. The average lower extremity Ashworth score decreased from 3.7 to 1.6 and the average upper extremity score decreased from 3.1 to 1.8 demonstrating a benefit for the upper extremities as well as the lower extremities. Intrathecal baclofen therapy is effective if implemented early, as early as 3 months after traumatic brain injury [119], and the benefit is prolonged, reportedly lasting 14 years and longer [121].
Spinal cord injury has a high association with traumatic brain injury , especially cervical spinal cord injury [122]. It has been estimated that over 200,000 people in the United States have a spinal cord injury with persistent neurological deficits [16]. Among patients with chronic spinal cord injury, a 65–78 % incidence of spasticity has been reported [17, 18]. A survey of patients with spinal cord injury showed that arm and hand function was the most important to the quality of life of those living with quadriplegia, and both walking movement and chronic pain were ranked with middle importance to the quality of life for those with paraplegia [16], all of which could potentially be improved with better control of spasticity when spasticity is present. In three clinical series including a combined total of 174 patients having continuous intrathecal baclofen therapy for spasticity of spinal origin, 29–41 % of which were traumatic spinal cord injury patients, the average Ashworth scale results decreased from a baseline of 2.5–4.2 down to 1.3–1.9 at 3 months or last follow-up [123–125]. Intrathecal baclofen therapy has relatively low risks, with the majority of risks coming at the time of pump and catheter implantation including reported 3.3 % pseudomeningocele, 2.9 % constipation, 2.4 % headache, and 2.2 % cerebrospinal leak [126], and long-term complication rate of 0.13 complications per pump-year [127] with the most common long-term complications being catheter kink or catheter migration at 4 % and infection at 1.2 % [126]. Studies have shown that intrathecal baclofen therapy for severe spasticity is successful in improving quality of life [128] and cost savings over time [129]. These results support the clinical application of this therapy which should be considered for appropriate patients following traumatic brain or spinal cord injury.
Pain
The pain syndrome most commonly associated with traumatic brain injury is headache with an estimated 57.8 % prevalence [130]. Traumatic brain injury can also be associated with complex regional pain syndrome, mostly due to the sequelae of the brain injury neurological deficits [131]. Recommendations for medical treatment of posttraumatic headaches are limited and include opioids for a short period of time then nonprescription pain relievers [132]. For patients with posttraumatic headaches that continue for a prolonged period of time and are refractory to any medical therapy, neuromodulation techniques may provide an opportunity for relief. Two case reports of high cervical spinal cord stimulation showed effective posttraumatic headache relief, with 90 % pain relief in one patient [133, 134]. Stimulation of the great auricular nerve produced 90 % posttraumatic headache relief in a case report of a single patient [135]. Motor cortex stimulation has also been reported in one patient to be effective in relieving posttraumatic facial pain due to injury [136]. Pain from spinal cord injury can be complex including one or several pain syndromes depending on the individual patient [137]. The prevalence of spinal cord injury associated pain varies based on clinical study, being anywhere from 26–96 % [137]. A review of the literature concerning spinal cord stimulation for spinal cord injury associated chronic intractable pain showed moderate benefit, with significant long-term pain relief in only 18–40 % [138].
Treatment of complex regional pain syndrome from extremity injury may also be controlled by neuromodulation . Complex regional pain syndrome can result from injury of an extremity, especially with the presence of a peripheral nerve injury. The prevalence of peripheral nerve injury in a population of patients with multiple injuries was 2.8 % [19]. There was a high association between peripheral nerve injury and head injury, with 60 % of the peripheral nerve injury patients having a head injury as well [19]. Complex regional pain syndrome, which frequently results from extremity and peripheral nerve injury, has an incidence in the population of 5.46–26.2 per 100,000 person years [21, 22] and a prevalence of 20.57 per 100,000 [22]. Complex regional pain syndrome can be challenging to treat with only 30 % having resolved symptoms, 16 % reporting progression of their symptoms and 31 % unemployed at 2 year or more follow-up [139]. Sixty percent of patients with spinal cord stimulators implanted for complex regional pain syndrome type 1 continued to use their stimulator for effective pain relief in a follow-up to 12 years [140]. In a randomized, prospective controlled trial of spinal cord stimulation for complex regional pain syndrome, stimulator patients had an average decrease in their 10 point visual-analog scale of 2.4 points compared to controls who had an average increase of 0.2 [141]. There was no significant improvement in their functional status, but the patients who had stimulators implanted had improvement in their health-related quality of life. The benefits that the patients with implanted stimulators enjoyed persisted through a 5 year follow-up period [142]. An economic analysis of these patients showed an initial $4000 higher cost for each patient implanted with a spinal cord stimulator, but a projected $60,000 savings for each spinal cord stimulator patient over his or her lifetime [143]. Although spinal cord stimulator therapy does not completely eliminate the pain from complex regional pain syndrome, it provides clear benefit to patients.
Robotics and Brain–Machine Interface
Recent advances in robotics and technologies of brain–machine interface hold promise for devices that could provide increased function in posttrauma patients. Robotic exoskeleton systems are commercially available and have been FDA approved for work with spinal cord injury patients in the clinical environment [144–147]. Seven clinically available robotic exoskeleton systems were identified in a 2015 review article [147]. The significant limitation of these devices is that they are not approved to be utilized at home and in the community. The promise of this technology will only be fully realized when combined with brain–machine interface technologies which are in the process of development and implementation. People with tetraplegia have successfully utilized current brain– machine interface technologies to independently move and meaningfully utilize advanced robotic upper extremity prosthetics directly or in virtual reality environments [148, 149]. Brain–machine interface technology has been utilized by spinal cord injury patients in a virtual reality environment to operate a walking simulator [150] providing proof of concept that robotic exoskeleton technologies may someday be effectively operated directly by the individual’s brain activity. Once realized, this technology combination would be extremely powerful in expanding functionality for the patient with trauma related disability.
Conclusions
The long lasting detrimental effects of neurological trauma can lead to significant pain and disability, but these effects can sometimes be mitigated by neuromodulation techniques. Established therapies include resection and stimulator implantation surgeries for epilepsy , intrathecal baclofen pumps for spasticity , and spinal cord stimulators for pain. Other therapies like deep brain stimulation for depression, memory deficits and disorders of consciousness show promise but are currently in limited clinical trials. Case reports suggest that other stimulation modalities for chronic intractable headache may be helpful. Future restorative therapy involving robotics and brain–machine interface technology are in development and could have an important impact on restoring function to severely injured patients.
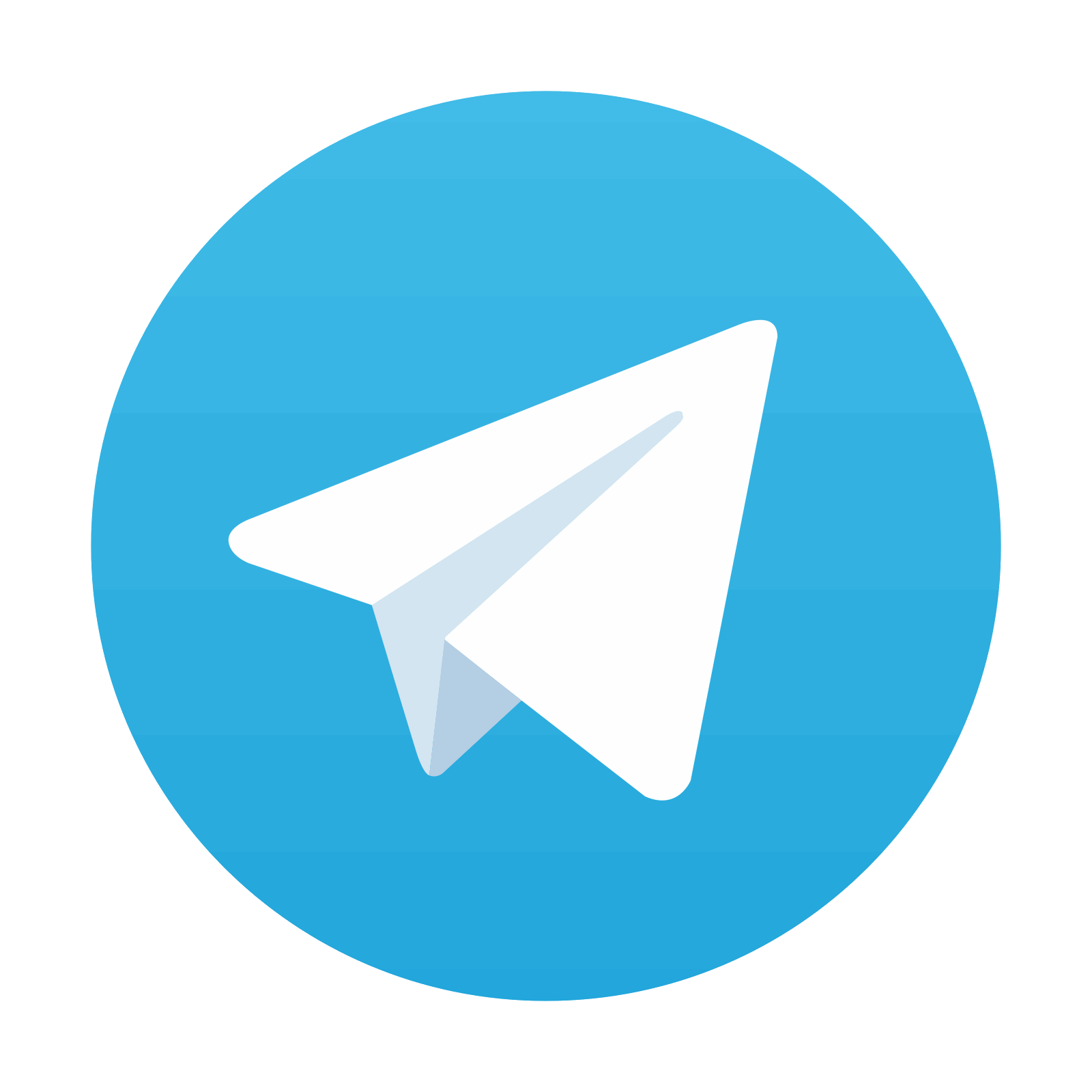
Stay updated, free articles. Join our Telegram channel

Full access? Get Clinical Tree
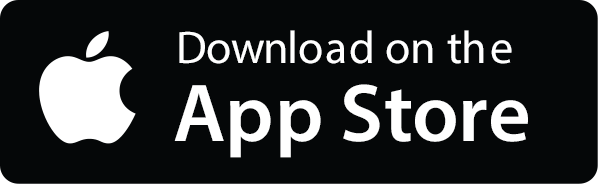
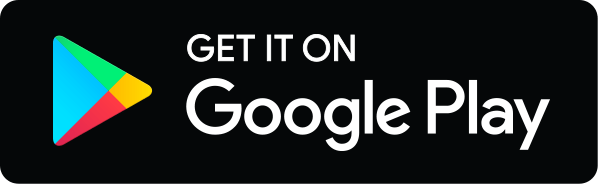