The preceding chapters presented the major structures seen at individual levels of the central nervous system (CNS) or in particular views of the brain. This chapter is complementary, using many of the same sections and views to indicate the structures and connections involved in particular neurological functions.
We have taken a “bare-bones” approach to this task and indicated only major pathways and connections. Much of the circuitry discussed in standard textbooks has been omitted in the interest of simplicity. The locations of neuronal cell bodies, the trajectories of their axons in tracts, and the locations of their synaptic endings are usually indicated by cartoon neurons like this one:

In addition, some anatomical liberties were taken to keep the diagrams relatively simple. The number of lines was minimized by indicating axons as diverging or converging:

(Axons frequently branch to innervate multiple targets, but this is not what we mean to indicate in any of these figures; moreover, axons from multiple neurons never converge to form a single axon.)
Finally, colors were used to make it easier to follow particular pathways in each figure. Their use is consistent within a given figure but not across figures: We were unable to devise a meaningful color scheme that would accommodate all the different functional systems. Hence a given color seldom has a functional implication.
Long Tracts of the Spinal Cord and Brainstem
- •
Fig. 8.1 . Touch sensation and proprioception: the posterior column–medial lemniscus system
- •
Fig. 8.2 . Pain and temperature sensation: the anterolateral system
- •
Fig. 8.3 . Voluntary movement: the corticospinal tract
Sensory Systems of the Brainstem and Cerebrum
- •
Fig. 8.4 . Connections of the trigeminal nerve
- •
Fig. 8.5 . Chemical senses: gustatory system
- •
Fig. 8.6 . Chemical senses: olfactory system
- •
Fig. 8.7 . Connections of the eighth nerve: auditory system
- •
Fig. 8.8 . Connections of the eighth nerve: vestibular system
- •
Fig. 8.9 . Visual system and visual fields
Cranial Nerve Motor Nuclei
- •
Fig. 8.10 . Cranial nerve nuclei that innervate ordinary skeletal muscle (via cranial nerves III, IV, VI, and XII)
- •
Fig. 8.11 . Cranial nerve nuclei that innervate muscles of branchial arch origin (via cranial nerves V, VII, IX, X, and XI)
- •
Fig. 8.12 . Voluntary movement of muscles of the head and neck: the corticobulbar tract
Visceral Afferents and Efferents
- •
Fig. 8.13 . Visceral and gustatory afferents, preganglionic sympathetic and parasympathetic neurons
Basal Ganglia
- •
Fig. 8.14 . The major circuit of the basal ganglia
- •
Fig. 8.15 . Connections of the striatum (caudate nucleus, putamen, nucleus accumbens)
- •
Fig. 8.16 . Connections of the globus pallidus
- •
Fig. 8.17 . Connections of the substantia nigra
- •
Fig. 8.18 . Connections of the subthalamic nucleus
Cerebellum
- •
Fig. 8.19 . Gross anatomy
- •
Fig. 8.20 . Cerebellar circuitry: afferent and efferent routes through the cerebellar peduncles
- •
Fig. 8.21 . Cerebellar circuitry: structure of cerebellar cortex
- •
Fig. 8.22 . Inputs to the cerebellum
- •
Fig. 8.23 . Outputs from the cerebellum to the forebrain
- •
Fig. 8.24 . Outputs from the cerebellum to the brainstem
Hypothalamus and Limbic System
- •
Figs. 8.28 and 8.29 . Connections of the hypothalamus
- •
Fig. 8.30 . An overview of the limbic system
- •
Figs. 8.31 and 8.32 . Major input/output pathways of the limbic system
- •
Fig. 8.33 . Inputs to the amygdala
- •
Fig. 8.34 . Outputs from the amygdala
- •
Fig. 8.35 . Inputs to the hippocampus
- •
Fig. 8.36 . Outputs from the hippocampus
Chemically Coded Neuronal Systems
- •
Fig. 8.37 . Cholinergic neurons and pathways
- •
Fig. 8.38 . Noradrenergic neurons and pathways
- •
Fig. 8.39 . Dopaminergic neurons and pathways
- •
Fig. 8.40 . Serotonergic neurons and pathways
- •
Fig. 8.41 . Modulatory outputs from the hypothalamus
Each posterior column terminates in the ipsilateral posterior column nuclei ( nuclei gracilis and cuneatus ), whose axons cross the midline and ascend to the ventral posterolateral nucleus ( VPL ) of the thalamus. VPL in turn projects to primary somatosensory cortex in the postcentral gyrus .
An analogous pathway conveying similar information from the face involves primary afferents with cell bodies in the trigeminal ganglion and the mesencephalic nucleus of the trigeminal nerve (see Fig. 8.4 ). Central processes of these afferents terminate in the main sensory nucleus of the trigeminal nerve. Their axons cross the midline, join the somatotopically appropriate region of the medial lemniscus, and ascend to the ventral posteromedial nucleus ( VPM ) of the thalamus. VPM in turn projects to the face area of the postcentral gyrus.
Tactile and proprioceptive information can also reach consciousness by way of postsynaptic fibers arising from spinal cord neurons. Some of these projections travel in the posterior columns, but others travel in pathways outside the posterior funiculus (e.g., tactile information in the anterolateral system described in Fig. 8.2 ), so posterior column damage does not cause total loss of touch and position sensation.


The analogous pathway dealing with the face involves trigeminal ganglion cells whose central processes descend through the spinal trigeminal tract to caudal parts of the spinal trigeminal nucleus (see Fig. 8.4 ). Axons of these second-order neurons cross the midline, join the somatotopically appropriate region of the spinothalamic tract, and ascend to VPM of the thalamus. VPM in turn projects to the face area of the postcentral gyrus.
Pain and temperature information is in fact more widely distributed than this simplified account would indicate and reaches the reticular formation, additional thalamic nuclei, and multiple cortical areas. Several additional pain pathways travel with or near the spinothalamic tract, and all are commonly referred to collectively as the anterolateral system .
Access to the spinothalamic tract is modulated by small neurons of the substantia gelatinosa (and at several other sites). One important pain-control pathway originates in the periaqueductal gray matter of the midbrain and involves a relay in the raphe nuclei (see Fig. 8.40 ) and nearby reticular formation of the medulla and caudal pons. The periaqueductal gray sums up anterolateral and limbic inputs to help determine when to turn this pathway on.
Commands for the initiation of voluntary movements are conveyed to the spinal cord via the corticospinal tract , which includes not only fibers originating in primary motor cortex of the precentral gyrus but also fibers originating in premotor , supplementary motor (not shown), and somatosensory cortex. Corticospinal axons descend through the internal capsule , cerebral peduncle , and basal pons in company with corticopontine and corticobulbar fibers . They then continue into the pyramids of the medulla. At the spinomedullary junction, most corticospinal fibers decussate (see inset below) to form the lateral corticospinal tract . Those that do not cross in the pyramidal decussation continue into the smaller anterior corticospinal tract and typically cross in the spinal cord before terminating. Finally, the axons originating in motor areas of the cortex terminate on motor neurons (or, more often, on nearby interneurons); those originating in somatosensory cortex terminate in the posterior column nuclei and posterior horn and presumably play a role in modulating the transmission of sensory information.
The trigeminal nerve conveys somatic sensory information from most of the head, and it is also the motor nerve for most muscles of mastication. Connections of the trigeminal motor nucleus are noted in Fig. 8.11 , and sensory connections are reviewed here. The connections of somatosensory components bear many similarities to those of spinal nerves (see Figs. 8.1 and 8.2 ), but there are important differences as well.
Large-diameter trigeminal afferents have cell bodies in the trigeminal ganglion or in the mesencephalic nucleus of the trigeminal (in effect, a bit of the trigeminal ganglion located within the CNS instead of in the periphery). Many central processes terminate in the main sensory nucleus of the trigeminal, which in turn projects through the contralateral medial lemniscus to VPM of the thalamus. (Part of the main sensory nucleus, where the mouth is represented, sends an uncrossed projection, the dorsal trigeminal tract, to the ipsilateral VPM; the functional significance of this uncrossed projection is unclear.) Other central processes project to the trigeminal motor nucleus as part of the masseter stretch reflex arc, or to trigeminocerebellar neurons in rostral parts of the spinal trigeminal nucleus .
Small-diameter trigeminal afferents, all with cell bodies in the trigeminal ganglion, travel through the spinal trigeminal tract to termination sites at various levels of the spinal trigeminal nucleus. The most caudal levels of the spinal trigeminal tract and nucleus are essentially rostral extensions of Lissauer’s tract and the posterior horn , respectively, of the upper cervical spinal cord and have an analogous function. That is, they convey information about facial pain and temperature to VPM. This seemingly odd location of the second-order neurons for facial pain and temperature ensures that, moving from the spinal cord into the brainstem, there is a smooth continuation of the somatotopic pain/temperature map. More rostral levels participate in trigeminocerebellar projections and in other reflexes, notably the bilateral blink reflex in response to an object touching either cornea.


Receptor cells in taste buds synapse on peripheral processes of fibers in the facial ( VII ), glossopharyngeal ( IX ), and vagus ( X ) nerves. Facial endings innervate taste buds on the anterior two thirds of the tongue, glossopharyngeal endings innervate those on the posterior third, and vagal endings innervate scattered taste buds of the epiglottis and esophagus. Central processes of these gustatory primary afferents travel through the solitary tract to reach second-order neurons in rostral parts of the nucleus of the solitary tract .
Second-order gustatory neurons influence feeding-related behavior and autonomic functions by projecting to the dorsal motor nucleus of the vagus , to the nearby reticular formation , and even to preganglionic sympathetic neurons in the spinal cord (not indicated in the accompanying figures). Conscious perception of taste is mediated by a largely uncrossed projection from the nucleus of the solitary tract to the thalamus ( VPM ), and from there to the gustatory cortex in the insula and the adjacent frontal operculum .
Gustatory information also reaches the hypothalamus and amygdala , where it influences metabolic regulation and feelings of hunger, satiety, and pleasantness or unpleasantness accompanying various tastes. The route utilized involves, at least partially, a projection from gustatory cortex to the amygdala. In many animals, gustatory information is also transmitted to the hypothalamus and amygdala more directly, through a projection from the parabrachial nuclei of the pontine reticular formation. Whether this pathway exists in humans is doubtful, as implied by question marks in Fig. 8.5B .
The olfactory bulb develops as an outgrowth of the cerebral hemisphere , leading to a unique arrangement of connections in the olfactory system. The axons of olfactory receptor neurons , collectively making up cranial nerve I , pass through the cribriform plate of the ethmoid bone and terminate in the olfactory bulb. Second-order olfactory neurons then project through the olfactory tract to a variety of nearby sites, all part of the ipsilateral cerebral hemisphere. Some olfactory tract fibers terminate in the anterior olfactory nucleus (which in turn projects through the anterior commissure to the contralateral olfactory bulb) or the olfactory tubercle (an inconspicuous part of the anterior perforated substance at the base of the cerebrum). The remaining fibers curve toward the temporal lobe as the lateral olfactory tract to reach olfactory cortical areas ( piriform cortex adjacent to the lateral olfactory tract, periamygdaloid cortex overlying part of the amygdala , and entorhinal cortex at the anterior end of the parahippocampal gyrus ) and a restricted part of the amygdala. This is the only known example of sensory information reaching the cerebral cortex directly, without a stop in the thalamus.
Olfactory information is subsequently distributed more widely, both by projections from these primary olfactory receiving areas and by relays in the thalamus. Some of these olfactory projections converge with gustatory and somatosensory information in an area of orbital cortex important for integrated judgments of flavor.
Auditory information reaches the brainstem via the cochlear division of cranial nerve VIII , a collection of primary afferent fibers with cell bodies in the spiral ganglion (embedded in the cochlea), peripheral processes that innervate cochlear hair cells , and central processes that terminate in the dorsal and ventral cochlear nuclei at the pontomedullary junction.
In contrast to the somatosensory system, the second-order neurons of the cochlear nuclei project bilaterally (crossing fibers in the trapezoid body ) to higher levels of the auditory system, allowing for sound localization by comparing inputs from the two ears. The first site where such binaural comparisons occur is the superior olivary nucleus . Efferents from each superior olivary nucleus, together with crossed and uncrossed projections from the cochlear nuclei, ascend to the inferior colliculus through the lateral lemniscus . The inferior colliculus then projects through the brachium of the inferior colliculus to the medial geniculate nucleus of the thalamus, which in turn projects to auditory cortex in the temporal lobe. Primary auditory cortex is located in the aptly named transverse temporal gyri (of Heschl ) on the superior surface of the superior temporal gyrus .
One consequence of this bilateral representation of each ear at all levels beyond the cochlear nuclei is that serious hearing loss restricted to one ear implies damage at the level of the cochlear nuclei or (more likely) the middle or inner ear or the eighth nerve.
Vestibular information reaches the brainstem via the vestibular division of cranial nerve VIII , a collection of primary afferent fibers with cell bodies in the vestibular ganglion , peripheral processes that innervate hair cells in the utricle , saccule , and semicircular ducts , and central processes that mostly terminate in the vestibular nuclei of the rostral medulla and caudal pons.
The vestibular nuclei then project to the spinal cord (to mediate postural responses to linear and angular acceleration, and to coordinate head movements with eye movements) and to the abducens, trochlear (not shown), and oculomotor nuclei (to mediate eye movements compensating for head movements—the vestibuloocular reflex ).
Abundant interconnections between the vestibular nuclei and the cerebellum (particularly the flocculonodular lobe and parts of the vermis ) assist in these tasks, aided by some vestibular primary afferents projecting directly to the cerebellum. Finally, there is a projection (not shown) from the vestibular nuclei to the thalamus through which changes in head position or motion reach consciousness.
The lateral and medial vestibulospinal tracts arise primarily from the lateral and medial vestibular nuclei respectively. Other vestibular connections, however, are shared among all the nuclei; the apparently exclusive connections in Fig. 8.8B are merely a mechanism for minimizing the number of lines in the figure.





Some of these axons—specifically, those for the superior oblique (CN IV) and superior rectus (CN III)—cross the midline before they exit. This is an unusual situation because motor neurons generally innervate ipsilateral muscles. It is presumed to be an adaptation to maintain certain interrelationships between head movements and eye movements (e.g., all the motor neurons needed to rotate both eyes in the same direction are located on the same side of the brainstem).
The abducens nucleus contains not only the motor neurons for the ipsilateral lateral rectus , but also a population of interneurons whose axons cross the midline and ascend through the medial longitudinal fasciculus ( MLF ) to medial rectus motor neurons. Simultaneous activation of both populations of neurons in the abducens nucleus thus results in conjugate gaze to the ipsilateral side.

The axons of these motor neurons leave the brainstem in cranial nerves V , VII , IX , X , and XI . Many of them make an odd, hairpin turn before their exit. Axons leaving the facial motor nucleus provide the most striking example, hooking around the abducens nucleus in an internal genu (accounting for the facial colliculus in the floor of the fourth ventricle —see Fig. 1.11A ).

Commands for the initiation of voluntary movements mediated by cranial nerves are conveyed to the brainstem via the corticobulbar tract , which includes not only fibers originating in primary motor cortex of the precentral gyrus but also fibers originating in premotor , supplementary motor (not shown), and somatosensory cortex. Corticobulbar axons descend through the internal capsule , cerebral peduncle , basal pons , and medullary pyramids in company with corticopontine and corticospinal fibers . At the levels of the motor nuclei of cranial nerves V , VII , IX , X , XI , and XII , contingents of these fibers peel off and terminate on motor neurons there (or, more often, on nearby interneurons). In contrast to the corticospinal tract , which mostly crosses in the pyramidal decussation , the corticobulbar fibers from each hemisphere are distributed bilaterally. This corresponds to the way in which muscles on both sides of the face and head are typically used simultaneously (e.g., chewing, swallowing, speaking). As a result of this bilateral distribution, unilateral damage to the internal capsule or corticobulbar tract typically does not cause substantial, lasting weakness of these muscles. The major exception is the muscles of the lower face, whose motor neurons receive a predominantly crossed corticobulbar input (corresponding to the way in which we can make asymmetrical lower facial expressions). Hence, weakness of one lower quadrant of the face is an important clinical sign of contralateral corticobulbar damage. (The nuclei of cranial nerves III, IV, and VI receive no direct corticobulbar inputs, because cortical projections instead reach brainstem pattern generators that in turn drive coordinated movements of the two eyes.)


a Striatum refers to the combination of caudate nucleus, putamen, and nucleus accumbens. (Nucleus accumbens and adjacent parts of the caudate, putamen, and basal forebrain are often referred to as the ventral striatum .)
globus pallidus , subthalamic nucleus , and substantia nigra , are prominently involved in motor control (and in cognitive functions as well, through parallel connections with other CNS areas). They affect movement not by projecting to motor neurons in the spinal cord or brainstem, but rather by influencing the output of the cerebral cortex. The principal anatomical circuit underlying this influence is a series of parallel loops of the type indicated in the inset below: A relatively widespread area of cerebral cortex projects to a particular region of the striatum, which by way of the globus pallidus and thalamus feeds back to the cerebral cortex (typically to a frontal or limbic area).Most parts of the cerebral cortex, and the hippocampus and amygdala as well, participate in such loops. Association areas of cortex are related most prominently to the caudate nucleus , somatosensory and motor cortex to the putamen , and limbic areas to the ventral striatum .
The substantia nigra and subthalamic nucleus form parts of additional basal ganglia circuitry, as indicated in Figs. 8.17 and 8.18 .

Striatal efferents form the next stage in the path back to cerebral cortex by projecting to both segments of the globus pallidus and to the reticular part of the substantia nigra , which in most respects may be considered an extension of the globus pallidus ( internal segment ).

The external ( GPe ) and internal ( GPi ) segments of the globus pallidus have distinct efferent projections. GPe projects to the subthalamic nucleus through the subthalamic fasciculus. (GPe also has widespread inhibitory outputs to other parts of the basal ganglia, omitted from this figure to keep it from getting too complicated.) GPi and SNr, in contrast, form the next stage in the path back to cerebral cortex, by projecting to the thalamus. Some efferents from GPi penetrate the internal capsule as a series of small fiber bundles collectively called the lenticular fasciculus ; others emerge from the inferior surface of GPi and hook around the internal capsule in the ansa lenticularis . The ansa lenticularis and lenticular fasciculus join cerebellar efferents underneath the thalamus to form the thalamic fasciculus .
Although pallidal efferents in this figure are portrayed as ending in the ventral lateral nucleus , many actually end in the ventral anterior nucleus . Others reach more widespread thalamic sites (as might be expected in view of the striatal inputs from diverse cortical areas), such as the dorsomedial nucleus .

The compact part ( SNc ) is a collection of darkly pigmented neurons (from which the substantia nigra derives its name) that receive inputs from the striatum and other sites, then project to the putamen and caudate nucleus and release dopamine there; their degeneration leads to Parkinson’s disease. A comparable set of dopaminergic neurons in the nearby ventral tegmental area projects to the ventral striatum , frontal cortex , and amygdala .

The most important of these connections from a functional standpoint may be inputs from the external segment of the globus pallidus and outputs to the internal segment of the globus pallidus (and to the reticular part of the substantia nigra ). These form part of an indirect route through the basal ganglia (see inset below). One general strategy used by the basal ganglia may be to facilitate some cortical activities by means of signals conveyed in the direct route (described in Fig. 8.14 ), while simultaneously suppressing competing cortical activities by means of signals in this indirect route. However, there is undoubtedly more to the story than this; it does not take into account, for example, the widespread inhibitory projections of GPe to sites other than the subthalamic nucleus.

Along lines roughly at right angles to the fissures, the entire cerebellum is divided into a narrow vermis that straddles the midline and a much larger hemisphere on each side.






The climbing fibers all come from one place—the contralateral inferior olivary nucleus —and wrap around the proximal dendrites of Purkinje cells, forming powerful excitatory synapses.
All other cerebellar afferents (other than a complement of the diffuse modulatory inputs indicated in Figs. 8.38 to 8.41 ) enter the cerebellum as mossy fibers and terminate on the vast numbers of tiny granule cells in the granular layer . (Granule cells account for half of the neurons in the CNS.) Granule cell axons ascend toward the cerebellar surface and bifurcate in the molecular layer to form parallel fibers , which run parallel to the long axis of a given folium. In so doing, each parallel fiber intersects the flattened, transversely oriented dendritic trees of hundreds of Purkinje cells, where they make excitatory synapses.

The cerebellum receives afferent inputs of three broad categories: afferents conveying information from the cerebral cortex, afferents conveying sensory information from a variety of subcortical sites, and climbing fibers from the contralateral inferior olivary nucleus .
Cerebral cortical input (mostly, but not entirely, from motor and somatosensory areas ) reaches the cerebellum through the middle cerebellar peduncle after a relay in the pontine nuclei . Most sensory information, arising most prominently in the spinal cord and vestibular nuclei , arrives via the inferior cerebellar peduncle (although a small amount traverses the superior peduncle ). Axons leaving each inferior olivary nucleus travel through the contralateral inferior cerebellar peduncle before ending in the cerebellum as climbing fibers.
(The connections of the cerebellum are actually more widespread than this simple account would indicate, and it seems to have correspondingly broader functions. For example, interconnections between the cerebellum and the hypothalamus have been described, and the cerebellum plays a role in coordinating autonomic functions. Cortical inputs from association areas such as prefrontal cortex indicate that the cerebellum, like the basal ganglia, is also involved in higher cognitive functions.)
Purkinje cell axons are the sole output from cerebellar cortex . Some leave the cerebellum entirely, through the inferior cerebellar peduncle , to reach the vestibular nuclei . Most, however, project to a series of deep cerebellar nuclei in the roof of the fourth ventricle, which in turn provide most of the output from the cerebellum.
There are three deep cerebellar nuclei on each side, arranged in a lateral-to-medial sequence: the dentate , interposed , b
b The interposed nucleus is itself a combination of the more lateral emboliform nucleus and more medial globose nucleus.
and fastigial nuclei . This arrangement of the nuclei corresponds to three longitudinal zones of cerebellar cortex: the large, lateral part of the hemisphere ; a smaller, medial part of the hemisphere (also called the intermediate zone ); and the vermis most medially. The pairing of deep nuclei and longitudinal zones of cortex is a reflection of functional subdivisions within the cerebellum that are also reflected in cerebellar inputs and outputs.The lateral part of each cerebellar hemisphere receives its major inputs from the cerebral cortex (motor, somatosensory, and other, more widespread areas) via pontine nuclei . Its outputs then influence the activity of motor and premotor cortex through a pathway involving the dentate nucleus and contralateral thalamus (primarily the ventral lateral nucleus ). It is thought to play a role in planning skilled movements.
The medial part of each cerebellar hemisphere receives information about the limbs from two major sources—motor cortex (via pontine nuclei) and the spinal cord (via spinocerebellar tracts ). It is thus strategically positioned to compare intended and actual movements and to assist with moment-to-moment correction of movement by way of connections with the interposed nucleus and motor cortex (via the thalamus).
The vermis receives information about axial muscles and body position from the vestibular nuclei and spinal cord and, through the fastigial nucleus, is involved in the maintenance and adjustment of posture. Most of this is accomplished at the level of the brainstem (see Fig. 8.24 ), and the connections of the fastigial nucleus with the thalamus are relatively minor.
Finally, the flocculonodular lobe (which does not fit comfortably into this longitudinal zonation scheme) is critically involved in eye movements by way of its connections with the vestibular nuclei .

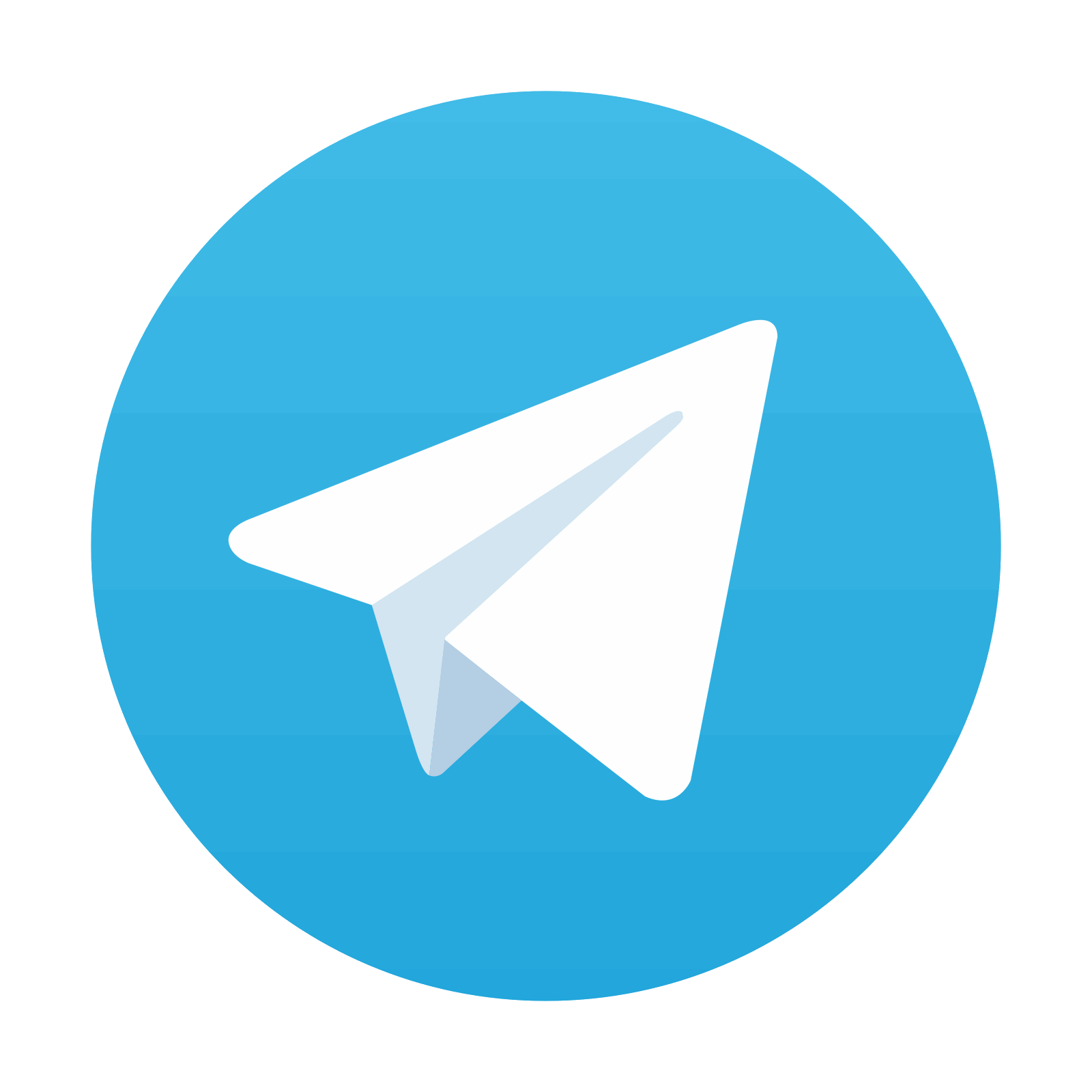
Stay updated, free articles. Join our Telegram channel

Full access? Get Clinical Tree
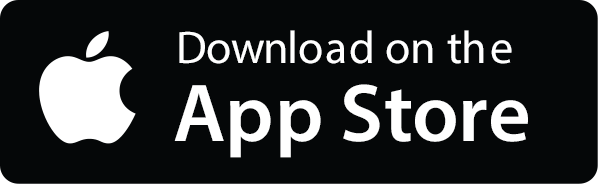
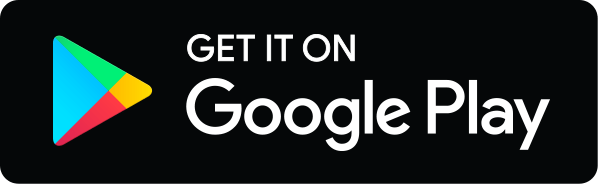
