Video-electroencephalography (EEG) studies are created by simultaneously recording the patient’s EEG and video image with sound. These are time-locked and combined for later review. The result is a powerful methodology for the clinical diagnosis and management of paroxysmal disorders. This chapter will focus on clinical motivation and technology underlying the combining of audio and video information with the EEG.
One of the most difficult symptoms to evaluate, whether in the emergency department or the neurologist’s office, is an episode of loss of consciousness. This complaint accounts for up to 3% of visits to emergency rooms and 1% of admissions to hospitals.1 The differential diagnosis of an episode of loss of consciousness is very long and includes cardiogenic, neurologic, and psychiatric illnesses. Although epilepsy is characterized by recurrent, highly stereotyped episodes of transient neurologic dysfunction, it is often difficult to distinguish from nonepileptic disorders based on routine evaluation alone. Paroxysmal movement disorders and sleep disorders are often mistaken for seizures. Transient alterations in cerebral blood flow and oxygenation, such as those that occur during a transient ischemic attack, orthostasis, syncope,2 or migraine, may cause recurrent events that resemble epilepsy. Integral to the process of narrowing this complex differential is the EEG. By combining the EEG with time-locked video information, the value of this tool is greatly enhanced.
Localization of seizure onsets is important for several reasons. First, identification of partial seizures may direct specific treatments. For instance, if a person’s partial seizures are refractory to medical management, surgery may be considered. For surgery to be successful, accurate identification of the region of seizure onset is needed.3
Seizure semiology, or description of the clinical appearance of an event, is a critical component of both diagnosis and localization. Of course, some brain regions are “silent.” That is, a seizure may start in one area but produce no obvious outward signs or symptomatic complaints. When the seizure spreads to the “symptomatic” cortex, localizing information becomes available.4
It can be very difficult, however, to reconstruct a person’s seizure semiology from the history alone. Because an epileptic seizure can affect a person’s thinking and memory, the patient may not have sufficient recall of events.5 Although descriptions obtained from witnesses may be helpful, most family members do not accurately recall the details of the events.6,7 They may have been too frightened or may not have known what signs were important to record. If events are infrequent, it may simply have been too long since the last event to accurately remember the details. In short, witnesses’ accounts of seizures are often incomplete or inaccurate.
Video recording of a patient’s habitual events can provide the clinician with a complete picture of the semiology. However, after viewing a seizure either in person or on video, even experienced epileptologists find it difficult to classify an event accurately.8 By time-locking a video recording with EEG and electrocardiogram data, the accuracy of the diagnosis and localization of an event increases dramatically.
Video-EEG has found increasing use in the intensive care unit (ICU).9–11 Potentially treatable EEG patterns have been detected underlying otherwise unexplained obtundation in critically ill patients,12 including periodic or stimulus-induced discharges or ictal events.11 Conversely, abnormal movements from many different causes are common. Establishing a precise relationship between observed movements or clinical events and the EEG is best done using EEG with time-locked video; this can greatly improve the quality and clinical impact of an EEG study. In addition, artifacts are common in the ICU and may easily be mistaken for physiological findings if simultaneous video is unavailable.13
Video data can be included in different types of EEG studies, both short and long term. Guidelines for the clinical advantages and disadvantages of these study modalities have been published14 (Table 4-1).
Study Type | Typical Duration | Probability of Capturing Event | EEG Quality | Video Quality |
---|---|---|---|---|
Routine EEG | 20–30 minutes | Low | High | High |
Ambulatory EEG | 24–96 hours | Medium | Low | Low |
Long term (EMU) | Days to weeks | High | High | High |
Long term (bedside) | Days to weeks | High | Medium to high | Low |
Long term (ICU) | Days to weeks | High | Medium | Medium to high |
Routine EEG is an integral part of the evaluation of transient episodes of neurologic dysfunction. Lasting on average 20 to 30 minutes, routine EEG, however, is very unlikely to capture a seizure. Because information about the person’s seizures is usually not recorded, the physician looks instead for markers of epilepsy called epileptiform discharges. In partial epilepsies, sporadic localized epileptiform discharges are the most characteristic paroxysmal interictal abnormality. Focal slowing may also occur. In idiopathic generalized epilepsies, generalized (usually bifrontal) rhythmic discharges are commonly found superimposed on an otherwise normal background. Often the epileptiform discharges in idiopathic generalized epilepsies have a frequency of 3 to 4 Hz. In symptomatic generalized epilepsies, multifocal epileptiform discharges are common, usually superimposed on a diffusely slow background.
A routine EEG does not always capture epileptiform discharges, even when a technically good study is performed. In other words, not every EEG in a person with epilepsy is abnormal. Marsan and Zivin15 looked at the presence or absence of epileptiform abnormalities on serial EEGs. The first recording showed epileptiform discharges in 56%. Repeated EEGs captured characteristic abnormalities in an additional 26%. Salinsky and colleagues16 found that four EEGs brought the yield for epileptiform abnormalities to 90%. When seizures are suspected, but the first EEG is “negative,” additional EEGs may help to confirm the diagnosis. If there is still doubt, prolonged EEG, such as ambulatory EEG or video-EEG monitoring (VEM), may be of benefit.
Because of the low likelihood that an event will occur during a routine study, most institutions do not record video when performing routine EEGs. In some cases, however, events during routine EEG may be much more likely to occur, for example, in patients with very frequent or reliably triggered events. Video recordings are simple to set up and could easily be done with each routine EEG. If an event were captured, the clinician would be able to visualize its semiology in the context of the technologist’s observations and the EEG recording. After the study has been read, any unnecessary video information could be discarded to conserve data storage space.
In addition to routine studies, video recorded in conjunction with EEG may be needed during specific medical tests. For instance, positron emission tomography (PET) and single-photon emission computed tomography (SPECT) may be performed as part of the surgical evaluation for refractory epilepsy. These tests measure brain metabolism, which is chronically affected by seizures.17 A seizure that occurs during the test will directly affect the test results; for example, an area of hypometabolism during interictal periods may become hypermetabolic during a seizure.18 When video-EEG is combined with these tests, any ambiguity as to whether a subtle seizure may have occurred can be dispelled.
Ambulatory EEG is a long-term study typically performed at home for 1 to 5 days. It offers one significant advantage over routine EEG: length of recording.19 Longer recordings equate to a higher chance of capturing clinical events, as well as a slightly improved chance of recording epileptiform discharges compared with a sleep-deprived routine EEG.20 In practice, ambulatory EEG recordings are much more likely to capture extended periods of sleep than a routine EEG. Epileptiform discharges are more likely to occur in sleep. In short, an ambulatory study has a greater chance of capturing abnormalities, especially those that might be infrequent.
It is difficult, however, to have a video component in an ambulatory study. The recording device is highly portable, but video operation is difficult in a home setting. To capture the events, the person must consistently refocus the camera unless it has an automatic focusing feature. In addition, the camera will not automatically “follow” the patient’s movements at home. The patient must always remain on camera, or at least remember to realign the camera in order to capture the events being studied. This becomes inconvenient, and more often than not, the person is “off camera” or outside the camera range when an event occurs.21
Video-EEG is usually performed in a hospital setting, either in a dedicated VEM unit or a neurologic intensive care unit (NICU) in which the equipment is built in, or by means of portable recording stations that can be placed anywhere in the hospital. EEG is recorded continuously with time-locked video and audio. The setting in the controlled environment of the hospital enables staff to monitor camera position, equipment operation, and EEG recording quality frequently and to correct technical problems as they arise. Clinical events can be marked into the record by means of an event button, under control of the patient or family members, or by staff who can type the information on a keyboard connected directly to the acquisition station. The time-lock function allows clinical event semiology to be directly tied to the EEG with an accuracy of better than 50 msec (Figure 4-1). In this way, a very detailed analysis can be done, significantly enhancing clinical care.
Figure 4-1.
Video time-locked to electroencephalogram (EEG). The vertical heavy black dashed line indicates the time point in the EEG corresponding to the video frame currently being displayed. The reviewer is able to play the video at different speeds to determine the precise correlation between even brief clinical events and the EEG. In the study depicted in this example, direct control of the cursor allowing the reviewers to play the video at arbitrarily slow speeds enabled them to determine that the frontally predominant spike and wave discharges shown correlated precisely with eye blinking.

In the evaluation of seizures, the video component of the EEG is essential. It allows the clinician to observe the seizure semiology directly. Whereas historical descriptions can be incomplete or inaccurate, the video is not. It can be reviewed and re-reviewed, and fine details about the person’s seizures can be documented. Family members can also watch the video and confirm that the event was truly representative of what they have observed at home.
Equipment used in conjunction with commercial video-EEG acquisition systems are typically “off-the-shelf” components that are designed for video surveillance over a network. The specifications for these components, outlined in the sections to follow, are not difficult to meet. In addition, components should be appropriately sized, relatively inexpensive, reliable and durable, and easily replaceable. The goals of monitoring, the chosen location, and the video-EEG acquisition system selected will dictate the type and arrangement of equipment that are ultimately selected.
Rooms dedicated for video-EEG use are optimal for VEM units and are a reasonable option for the NICU setting as well.13 These typically employ ceiling- or wall-mounted cameras and microphones connected directly to a built-in EEG acquisition station. Out of the reach of most people, and typically protected by a transparent plastic bubble or shield, these cameras are directed in such a way as to include the patient’s bed and living area in their fields of view (Figure 4-2) and can be controlled remotely. Specially trained staff members are available to monitor the video recordings, adjust camera positions to keep the patient in view, and respond to clinical events as the need arises. Audio and video data are recorded continuously, time-locked with the EEG by the data acquisition station, then transmitted to a centralized database. Events are reviewed frequently, at least once a day, by the treating team directing clinical care.
Figure 4-2.
Schematic diagram of a typical video-EEG monitoring unit (VEMU) bed, showing the camera, infrared light, and microphone locations in the patient’s living area. In this room, the patient spends most of his or her time in the bed or the chair next to the window. Both of these locations are well covered by the video camera mounted near the opposite wall and by the microphone mounted above the bed. The small size of the room makes it easier to keep the patient on camera as often as possible, while allowing for privacy in the bathroom area.

It is often advantageous to perform video-EEG studies at locations other than these specialized units. This may occur in an overflow situation, where video-EEG is clinically indicated, but no dedicated beds are available, or when the patient’s medical needs require placement elsewhere. For example, a patient may require an isolation room for infection control purposes or may need specialized nursing care. Portable systems may also be a more cost-effective and practical solution for hospitals with limited resources or low clinical volume. In fact, some hospitals use portable units exclusively. Portable video-EEG units can be used in nearly any clinical setting: regular hospital rooms, isolation units, ICUs, and even emergency department, where prolonged EEG may be initiated to aid in the treatment of status epilepticus, for instance.
Portable systems usually consist of a rolling cart with an EEG acquisition machine and an affixed camera, essentially comprising a moveable seizure monitoring station. As with hard-wired rooms, the video and EEG are time-locked. Events are recorded, then reviewed by the treating team. Portable units, however, have several disadvantages compared to permanent installations. Because of frequent handling of the camera and its location in the high-traffic area next to a patient’s bed, the equipment is susceptible to mechanical breakdown, accidental movement, and usage errors.13 A portable camera also cannot be adjusted constantly to keep the patient in its field of view, as is the case with a permanently mounted camera controlled remotely by trained hospital staff. Depending on the specific layout of the room, it may be difficult to position the camera to obtain a clinically useful view of the patient even under the best of circumstances.
Guidelines for the selection of cameras and related equipment are published by the American Clinical Neurophysiology Society (ACNS).14 Vendors of commercial EEG systems generally offer specific suggestions on a per-site basis.
Video systems that are used in dedicated VEM units or NICU beds should be fixed in place. The best mount configuration places the camera in the ceiling, in a location that permits viewing of all areas in the room to which the patient has access (except in areas where privacy is needed, i.e., the bathroom) while in wide-angle mode. If possible, the room should be small to facilitate optimal coverage.
The video system should be designed for 24-hour use and be able to work in environments with very low light. Standard monochrome (black-and-white) cameras require illumination of 0.5 foot-candles. Color imaging, which is preferred due to its improved resolution of facial features, requires 25 foot-candles of illumination; this mode can be used during normal daytime conditions but is not suitable for the reduced lighting that is typical during the night. Attention should be given to light levels in rooms intended for video-EEG studies, as many hospital rooms may not have sufficient lighting for standard videography. Low-light monochrome cameras can be used with as little as 0.03 foot-candles of illumination, making them suitable for nighttime use. The camera should be equipped with an automatic iris to prevent “blooming” caused by sudden increases in light level. Low-light-level color can be achieved with as little as 1.0 foot-candle of illumination, which would require the use of a night-light, which may be acceptable to some patients. However, the incremental value of this type of recording is not established.
Ideally, cameras should be able to capture good quality color images in daylight conditions and operate in low-light mode at night (Figure 4-3). To improve image quality at night, an infrared light source should be installed. Low-light-level cameras are particularly sensitive to red light, whereas humans have limited ability to perceive infrared wavelengths. The infrared light source in Figure 4-3, though visible in the photo, is not noticeable to persons in the room. Such lighting will not interfere with the patient’s normal sleep schedule and light-level preferences.
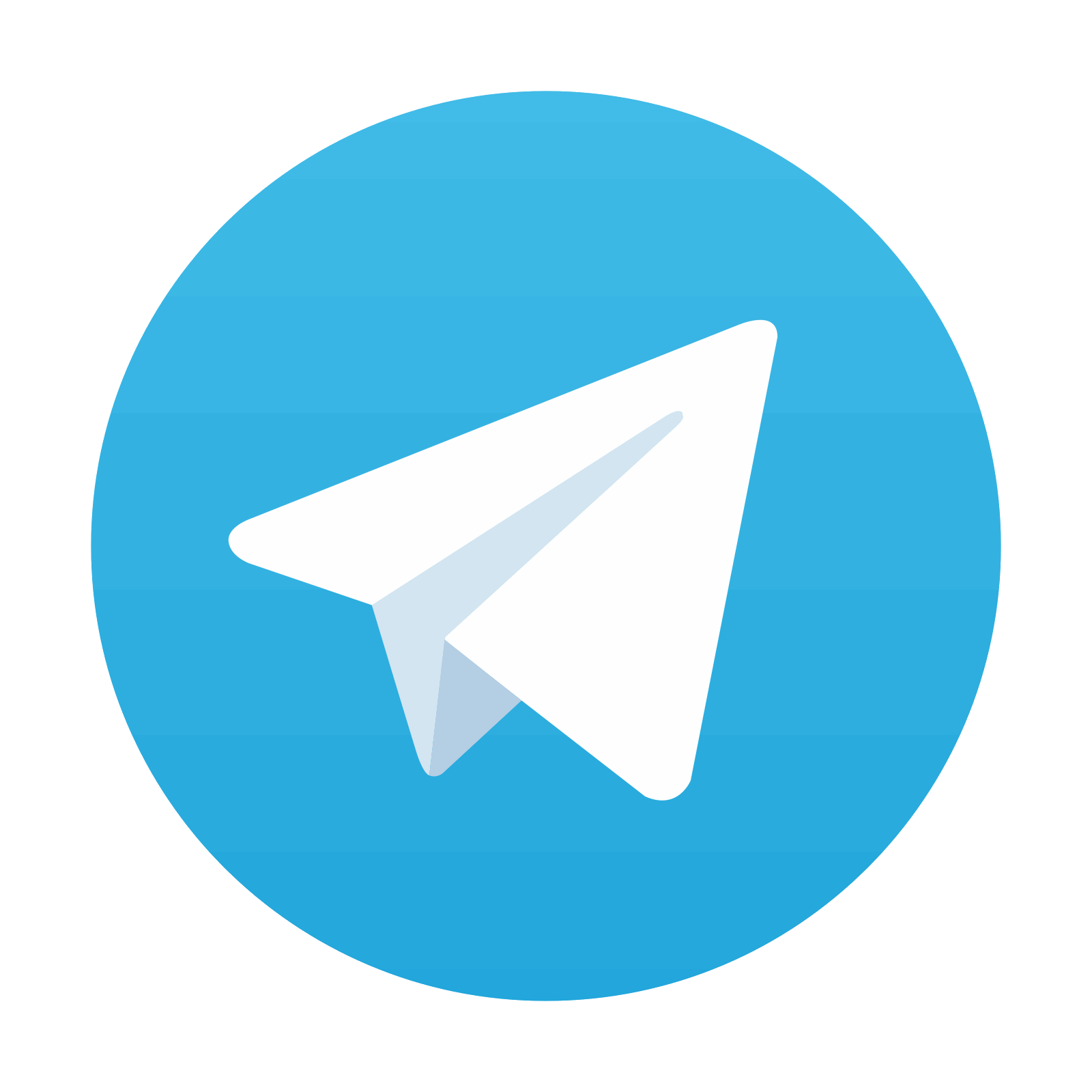
Stay updated, free articles. Join our Telegram channel

Full access? Get Clinical Tree
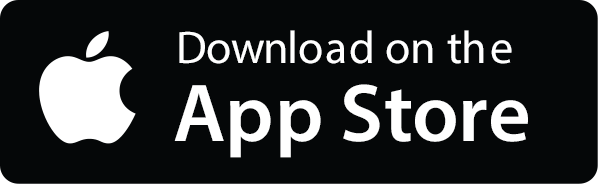
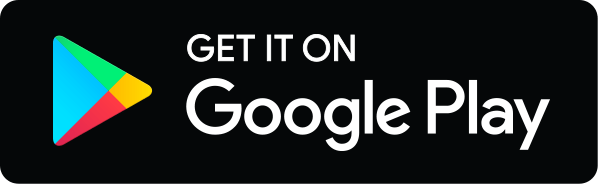