A better understanding of the biology of chordomas is required to deal with this debilitating disease. Significant progress has been made in understanding the biology of chordomas. Probably the biggest discovery was that of a frequent gene duplication of the transcription factor brachyury in patients with familial and sporadic chordomas. 3,4,5 Aberrant activation of this transcription factor is considered to be the hallmark of chordomas and differentiates them from other skull base tumors with similar appearance such as chondrosarcomas. 6 Even fetal notochordal cell rests, which were thought to be precursors of chordomas, do not have nuclear brachyury expression. Very little is known on the regulation of brachyury signaling in development and cancer. 7 However, such a unique alteration also carries the potential of very selective therapeutic targets in chordomas. Inhibition of brachyury by RNA interference has resulted in growth arrest, apoptosis, and a more differentiated morphology in cell culture. 7,8 Alterations in intracellular signaling pathways related to brachyury expression have also been investigated. Finally, brachyury has also been utilized as an immunotherapy target, and a heat-killed yeast vaccine has entered clinical trials (NCT02383498). 9 These findings show that brachyury and related signaling is a valuable target for therapeutic interventions. Nevertheless, brachyury is not the only promising candidate. Intracellular signaling changes specific to chordomas all result in differences from the normal biology. 10,11,12,13 Identification of these differences as therapeutic targets in chordomas is another forefront. 12 This would lead to personalized chordoma care, where individual variations in chordoma oncogenesis can be exploited to stop or slow down growth. 12,14 Several tyrosine kinase receptors were shown to be overexpressed in chordomas and preclinical and clinical trials targeting these signaling mechanisms have been performed. 10,11,13,15,16 Better understanding of the biology of chordomas will also lead to development of more sophisticated in vitro and in vivo disease models. Numerous cell lines have been isolated from chordomas and characterized, and these models have been serving to better understand the biology and test therapeutic interventions. 15,16,17,18,19,20 Xenograft models using these cell lines or primary cultures have also been used. 16,18,19,20 In the last few decades, cell lines and xenograft models were followed by more sophisticated cellular models where appropriate cellular precursors were transformed by the addition of human cancer oncogenes and tumor suppressor genes. 7,21 These animal models carry the molecular changes present in human cancers and therefore are better models to test therapeutic strategies for human diseases. 7 There is only one such experimental chordoma animal model, which was developed in zebrafish. 7 Similar sophisticated and innovative animal models, similar to those utilized in gliomas, are needed to facilitate chordoma research. It has long been recognized that not all chordomas behave the same. Factors underlying this dramatic difference in survival have not been studied in detail. It is still not known today whether the difference stems from individual differences (location, size, and extent of the individual chordoma), therapeutic interventions (efficiency of surgery and radiation therapy), or from variation in biological behavior. If such biological subtypes with variable clinical behavior can be identified, there is also the potential of identifying molecular fingerprints and exploiting these differences in developing rational treatment algorithms (aggressive treatments for aggressive tumor biology and conservative treatment paradigms for cases with more benign biology). Another important aspect is the interaction of chordoma cells with the surrounding bone and connective tissues. Better characterization of this tumor forefront and understanding the biology of chordoma bone invasion has the potential of augmenting both surgical interventions and radiation treatment and may yield new therapeutic targets. Last but not least, deciphering of personal predisposition and vulnerability to chordoma development is another aspect of chordoma biology. Although rare familial cases have been described, we know today that chordoma is not a Mendelian inherited disease. On the other hand, it is not known whether chordomas are related to environmental exposures. Sophisticated epidemiologic research is needed to clarify causative factors of chordomas. Large national and international databases provide the material for epidemiologic research and shall be promoted. 22,23,24 Most chordomas in the skull base, spine, and sacrum have a very typical radiologic appearance, and magnetic resonance imaging (MRI) has very high sensitivity and specificity in the differential diagnosis of bone lesions along the axial skeleton. However, exceptions are not rare. It has also been shown that conventional MRI sequences cannot reliably make the differential diagnosis between ecchordosis physaliphora, spinal fetal notochordal cell rests, chordomas, and chondrosarcomas, all of which have quite distinct biology and clinical behavior. 25,26 Therefore, there is still room for further development and refinement for MRI in chordoma imaging. Another exciting subject is molecular imaging.18F-2-fluorodeoxyglucose positron emission tomography (FDG-PET-CT) was successfully utilized in skull base, spinal, and sacral chordoma imaging. 27,28 It was also shown to be useful as a tool in the differential diagnosis of clival bone tumors. 29 PET imaging of specific chordoma markers could aid in detection and follow-up of postsurgical residual tumor and tumor recurrence and response to treatment. Studies have shown that 18F-fluoromisonidazole PET-CT is used to detect tumor hypoxia as a potential marker for radiation resistance in chordomas. 30,31 Advanced PET imaging with novel techniques and novel protein targets carries significant potential in chordoma imaging, but such novel technologies need validation in the clinic. Wide adoption of skull base surgery, its advancement, and use of assistive intraoperative technologies have greatly improved surgical results in the last few decades. The application of various or multiple approaches with the aim of a radical resection is paramount now toward the best outcome. Most of the intraoperative imaging and monitoring technologies have also been demonstrated useful in chordoma surgery. These technologies include intraoperative imaging modalities such as intraoperative MRI and intraoperative CT, as well as neuronavigation, endoscopy, and intraoperative neuromonitoring. Most of these techniques are widely available today, and most are utilized for skull base chordoma surgery. Nevertheless, there is still a need to make them available to any center around the world specializing in skull base surgery. The use of these systems certainly needs to be justified, because scientific, quantitative studies assessing the accuracy and reliability as well as those assessing their impact on surgical results and patient outcome are still sparse. Current knowledge indicates that an oncologic total resection can seldom be achieved in chordomas. This is due to the invasive nature of the tumor in the deep central skull base. It is of great importance to determine the extent of resection and the extent and anatomical localization of residual disease after chordoma surgery. There is compelling evidence that the surgeon’s estimation on the extent of resection is inaccurate. Therefore, early postoperative MRI and CT must be standards in chordoma care. MRI and CT provide complementary information, and together these early postoperative studies will be the baseline to compare follow-up imaging. However, this is not enough. When residual tumor tissue is identified in postoperative imaging, it is already too late. An unplanned and unanticipated second session in surgery is traumatic for the patient and for the surgeon and comes at the cost of increased complication rates. Therefore, residual tumor must be identified while still in surgery, before the patient is awakened. This will create an opportunity for further tumor resection. Gross tumor remnants can be hidden at corners and blind spots of surgical corridors in any tumor surgery, but in chordomas this is further complicated by the infiltration pattern of chordomas. Tumor remnants in chordoma surgery can be identified at blind spots as well as behind normal-appearing bone. Unlike other benign skull base tumors such as meningiomas that grow by expansion from an epicenter, chordomas invade the surrounding skull base in an unpredictable pattern. It is almost routinely seen that grossly normal-appearing bone tissue hides chordoma extension in the back. Some form of intraoperative imaging and navigation are therefore necessary to achieve extensive tumor resections. Intraoperative MRI and CT provide an almost excellent delineation of residual tumor tissue. This can be further augmented by direct comparison with preoperative imaging studies. Intraoperative MRI has been successfully instituted in chordoma surgery and has shown great promise in improving surgical outcome. Almost all imaging sequences can also be performed intraoperatively to provide a comprehensive estimation of the surgical efficiency. The impact of intraoperative MRI on volumetric tumor resection or the impact on long-term patient outcome has yet to be evaluated. After 2015, the high-field MRI systems and the ultra-low-field portable systems dominate the field of intraoperative MRI. There are no comparative studies that have assessed which system is more practical and efficient in chordoma surgery. Neuronavigation is also extremely helpful during chordoma surgery. As there is no intraoperative anatomical shift during surgery in the stiff, bony skull base (comparable to the brain shift that is observed in surgery for intrinsic brain tumors) neuronavigation provides a very accurate and real-time guide during chordoma surgery. 32,33
31.3 Imaging of Chordomas
31.4 Surgery of Chordomas
31.4.1 Intraoperative MRI and CT
31.4.2 Neuronavigation
Stay updated, free articles. Join our Telegram channel

Full access? Get Clinical Tree
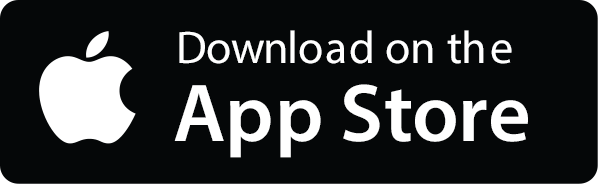
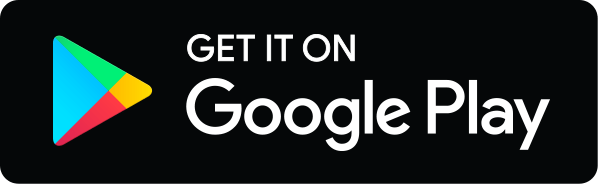