Chapter 3 Gait analysis
Gait analysis offers an opportunity for clinical assessment of the act of walking, one of the most important features of the individual’s use pattern that displays posture in action – acture. Under normal conditions when no dysfunctional factors impact on gait, the act of walking operates at a virtually unconscious level. However, when modifications to normal locomotion are demanded as a result of dysfunctional neuromusculoskeletal or other pathological states (e.g. intermittent claudication or other vascular disease), unconscious and conscious adaptations, often of a carefully considered nature, may be demonstrated. A sound understanding of gait mechanics (discussed in this chapter) as well as the anatomy of the foot and ankle is needed to apply the information of this chapter. The reader is referred to Chapter 14 for details regarding foot anatomy as well as discussion of some of the dysfunction patterns referred to in this chapter.
Normal joint and segment motion during the gait cycle
In order for the individual to progress from one location to the next, muscular action, together with gravity, propels the ‘primary machinery of life’ (Korr 1975) – the musculoskeletal frame – through a series of complex and, when normal, highly efficient steps. For the purpose of discussion, only the components of forward walking are considered in this text as the processes of walking backwards or sideways, climbing stairs, race walking and running are completely different and, while clearly having assessment value, are beyond the scope of this discussion of basic analysis.
When gait is looked at simply, two functional units emerge (Perry 1992): the passenger unit and the locomotor unit. The passenger unit incorporates the head, neck, arms, trunk and pelvis and presents its center of gravity just anterior to the 10th thoracic vertebra (T10). It is referred to as the HAT unit (Elftman 1954, Perry 1992) and it sits upon the locomotor unit, composed of the pelvis and lower extremities, which is responsible for weight bearing while simultaneously providing ambulation. (Note that the pelvis plays a role in both units.)
The locomotor unit performs the exceptional feat of providing structural stability while at the same time providing mobility, by transferring support from one lower limb to the other, then propelling the relieved leg forward in front of the other to catch the body mass as it falls forward, at which time it prepares to regain balance and bear the full weight again. This remarkable ‘gait cycle’ is repeated by first one leg and then the other, at varying speeds, on numerous terrains and often while the passenger unit is carrying a variety of items (purses, luggage, children, etc.), which can alter its own center of gravity (located just anterior to T10) as well as the center of gravity of the body as a whole (located just anterior to S2) (see Box 3.1).
Box 3.1 Gait characteristics
• initial contact (heel strike)
• loading response (foot flat)
• terminal stance (heel lift, push-off)
Swing phase (40% of gait cycle), when the foot is moving forward, is divided into:
In other words, walking is the forward offsetting of the body’s center of gravity, causing the mass to fall forward, at which time a limb is advanced to stop the forward fall. Perry (1992) explains:
At the end of the step the falling body weight is caught by the contralateral swing limb, which by now has moved forward to assume a stance [weightbearing] role. In this manner a cycle of progression is initiated that is serially perpetuated by reciprocal action of the two limbs (Fig. 3.1).
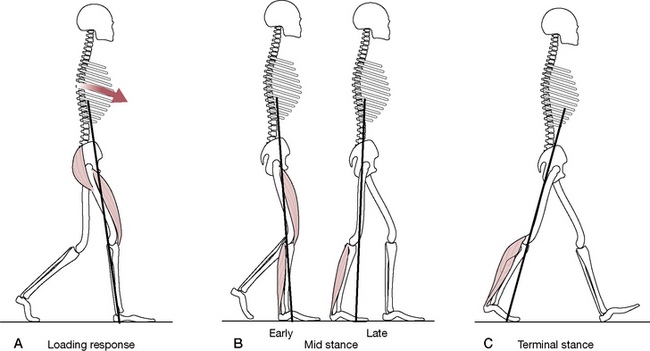
(adapted from Perry J 1992 Gait Analysis: normal & pathological function, with permission from SLACK Incorporated).
Each limb, as it transits through its gait cycle, has three basic tasks. It must first accept the weight of the body (weight acceptance – WA), then transfer all the weight onto a single limb support (SLS) and then provide limb advancement (LA) when unloaded. In accomplishing these three tasks, the motion of individual involved joints must be functional and their movements choreographed with each other in a seamless, well-timed manner. Even minute variations from normal may demand significant compensations by numerous muscles and from other body regions (see Box 3.2).
Box 3.2 Observation of gait
Greenman (1996) offers the following gait analysis in multiple directions as step one of his screening examination of the patient.
1. Observe gait with patient walking toward you.
2. Observe patient walking away from you.
3. Observe the patient walking from the side.
4. Observe the length of stride, swing of arm, heel strike, toe off, tilting of the pelvis, and adaptation of the shoulders.
5. One looks for the functional capacity of the gait, not the usual pathological conditions. Of particular importance is the cross-patterning of the gait and symmetry of stride.
Specific body motions, which are universally observable, occur as the body attempts to maintain a relatively narrow margin of displacement of its center of mass (CoM) during the gait cycle. These help form rather unique characteristics within the gait that are sometimes so distinct that the person is recognizable from a moderate distance simply from the walking ‘style’. Three different deviations are of particular importance. (Inman et al 2006):
1. The body speeds up and slows down as the swinging leg accelerates forward, then slows the body down as it impacts the ground, then speeds the body up again as it passes to the rear. A ‘surging’ is readily observed as one carries a full pan of water when walking.
2. The body rises and falls as it passes over the supporting limb. Functional mechanics of both knee and ankle help determine the amount of undulation.
3. The body displaces laterally to balance over the supporting limb. The amount of lateral excursion is influenced by the tread width, that is, the distance of the two feet from the forward plane of progression.
Rose & Gamble (2006) note, ‘While individual variations in the measured magnitudes of these motions will always be observed in any group of people, the motions will be present to some degree in everyone. Normally there is a symmetry in the movement, and the patterns repeat themselves with each successive cycle.’
The gait cycle consists of the full cycle that an individual limb goes through from its initial contact (heel strike, in the normal gait) to the next (heel) contact by the same foot. This cycle is also sometimes called a stride, which contains two steps – one by each foot. In actuality, the starting point could be considered to be at any part of the cycle but it is most often thought of as the heel strike, since the floor contact is the most definable event (Perry 1992).
Each gait cycle is divided into:
• stance period (60% of gait cycle) during which time the foot is in contact with the surface (25% of the gait cycle involves double limb support with both feet touching the ground) (see Box 3.3)
• swing period (40% of gait cycle) during which time the foot is moving forward, usually not in contact with the walking surface.
Box 3.3 Stance period
The stance phase begins with the heel strike, also called the initial contact (Perry 1992) since some people are unable to strike the heel and present the entire flat foot instead. Ideally, the knee is fully extended and the hip is flexed. The ankle is at 90°, being maintained there by the dorsiflexors (tibialis anterior, extensor hallucis longus, extensor digitorum longus). This contact also begins initial double limb stance since the second leg is still in contact with the floor, though there is not yet equal sharing of body weight by both legs.
To assist the acceptance of body weight, the heel functions as a rocker. The posterior portion of the calcaneus contacts the surface and the body ‘rocks’ over the rounded bony surface as the remainder of the foot simultaneously falls to the floor in loading response (foot flat). This rapid fall of the foot is decelerated by the dorsiflexors, which also restrain ankle motion and act as shock absorbers (Fig. 3.4).
Once the forefoot contacts the floor, joint motion shifts to the ankle as the movement of the tibia begins to ‘rock’ over the talus (ankle rocker) at which time the knee slightly flexes. This period of mid-stance is the introduction of single limb support, which requires not only the acceptance of full body weight but also the repositioning (laterally) of the passenger unit to align over the weight-bearing foot. The soleus muscle must utilize selective control to stabilize the lower leg while simultaneously allowing the tibia to advance over the ankle (Fig. 3.5).
Once the body weight has passed over the ankle, the knee and hip extend and the weight begins to transfer to the forefoot. As the foot prepares to leave the ground (push-off), the heel lifts from the ground (initiating terminal stance) and the movement shifts to the metatarsal heads, which serve as the forefoot rocker through pre-swing, after which the swing period begins (Fig. 3.6).
At the onset of the stance period, the forward limb is in initial double support, as long as both feet are still touching the ground. Single support is initiated by toe-off of the contralateral limb and ends as the contralateral heel strikes the ground, which begins terminal double stance for the supporting leg and initial double stance for the swinging leg. These terms are less confusing when one notes that as one leg is in initial double support, the other is in terminal double support. In between the double supports, one leg experiences a swing period while the other is in single support of the body’s weight (see Figs 3.3 and 3.9).
Each period (sometimes also called a phase) may be subdivided into smaller units or subphases (Cailliet 1997, Gray’s Anatomy 2005, Hoppenfeld 1976, Perry 1992, Root et al 1977), the terms for which vary from author to author (see Boxes 3.3 and 3.4). This text lists Perry’s descriptive system, which names eight functional patterns (subphases) with alternative names from other authors noted in parentheses (see also Figs 3.2 & 3.3).
Box 3.4 Swing period (limb advancement)
• Perry (1992) describes three phases in limb advancement, these being initial swing, mid-swing and terminal swing. Other authors (Cailliet 1997, Hoppenfeld 1976) note a similar division using different nomenclature (acceleration, mid-swing, deceleration).
• Initial swing begins as the foot lifts from the floor and ends when the swinging foot is opposite the contralateral foot, which is in its own mid-stance. The initial swing phase must produce foot clearance through ankle dorsiflexion and increased knee flexion as well as advancing the thigh through hip flexion. Without adequate flexion of all three of these, the toe or foot may strike the ground during the swing, as happens in conditions of foot drop.
• Mid-swing continues to provide floor clearance of the foot through continued ankle dorsiflexion as the hip continues to flex and the knee begins to extend. During the early part of this phase the tibialis anterior and extensor hallucis longus increase their activity significantly.
• Terminal swing provides full knee extension, neutral positioning of the ankle and preparation for initial floor contact (heel strike). Terminal swing phase ends the gait cycle and with surface contact, a new gait cycle begins.
Stance period | Swing period |
---|---|
Initial contact (heel strike, acceleration) | Initial swing |
Loading response (foot flat) | Mid-swing |
Mid-stance (deceleration) | Terminal swing |
Terminal stance (heel lift, push-off) | |
Pre-swing (toe-off) |
The task of weight acceptance begins with initial contact with the surface and the subsequent loading response of the limb. This period can also be described as a ‘rocker’ system in which there is an initial contact with the heel and a loading response (heel rocker); this is followed by mid-stance (ankle rocker) and finally a terminal stance and pre-swing (metatarsal rocker or forefoot rocker) (Perry 1992, Prior 1999) (Figs 3.4–3.7).
While more problems are evident during the stance period due to its weight-bearing responsibilities, the swing period nevertheless presents high demands on the body to maintain balance while also lifting and advancing the limb in preparation to begin the cycle again. This highly orchestrated chain of events includes flexion of the hip, flexion of the knee, eventual extension of the knee and positioning of the foot in preparation to bear weight, as well as three movements of the pelvis – rotation, tilt and shift (discussed further following this section). Detailed accounts of stance period and swing period are found in Box 3.3 and Box 3.4, respectively.
Musculoligamentous slings and influences and the gait cycle
It is tempting to focus on the muscular components as the source of movement in gait, since it is logical that when muscles fire, joints move, and this appears to drive the body forward. However, this alone cannot create the monumental ‘push’ that is required in order to walk. Instead, Dananberg (2007) suggests that the body be viewed as a perpetual motion gait machine. ‘The pendular actions of arms and legs act reciprocally, storing potential energy and returning kinetic energy in the process. These actions are visible as counter-rotations between the pelvic and shoulder girdles. Storage occurs in the ligamentous, muscular, and tendinous structures of the lower back (Dorman 1995).’ Highlighting the continuity between the ipsilateral latissimus dorsi and contralateral gluteus maximus muscle via the thoracolumbar fascia Dananberg highlights how ideally suited this structure is for this storage capacity. ‘Each step prepares for the next one; the effect is to create a forward-directed rotation on the pelvic hemisphere as it coordinates with the limb that is about to begin the swing phase motion (Gracovetsky 1987).’
• As the right leg swings forward the right ilium rotates backward in relation to the sacrum (Greenman 1996).
• Simultaneously, sacrotuberous and interosseous ligamentous tension increases to brace the SIJ in preparation for heel strike.
• Just before heel strike, the ipsilateral hamstrings are activated, thereby tightening the sacrotuberous ligament (into which they merge) to further stabilize the SIJ.
• Vleeming & Stoeckart (2007) have demonstrated that as the foot approaches heel strike there is a downward movement of the fibula, increasing (via biceps femoris) the tension on the sacrotuberous ligament, while simultaneously tibialis anterior (which attaches to the first metatarsal and medial cuneiform) fires, in order to dorsiflex the foot in preparation for heel strike.
• Tibialis anterior links via fascia to peroneus longus (which also attaches to the first metatarsal and medial cuneiform) under the foot, thus completing this elegant sling mechanism (the ‘anatomical stirrup’) that both braces the SIJ and engages the entire lower limb in that process.
• Biceps femoris, peroneus longus and tibialis anterior together form this longitudinal muscle–tendon–fascial sling, which is loaded to create an energy store (see p. 315), to be used during the next part of the gait cycle.
• During the latter stage of the single support period of the gait cycle, biceps femoris activity eases, as compression of the SI joint reduces and the ipsilateral iliac bone rotates anteriorly.
• As the right heel strikes, the left arm swings forward and the right gluteus maximus activates to compress and stabilize the SI joint.
• There is a simultaneous coupling of this gluteal force with the contralateral latissimus dorsi by means of thoracolumbar fascia in order to assist in counterrotation of the trunk on the pelvis.
• In this way, an oblique muscle–tendon–fascial sling is created across the torso, providing a mechanism for further energy storage to be utilized in the next phase of the gait cycle.
• Lee (2004) mirrors Dananberg’s view when she points out that together, the gluteus maximus and latissimus dorsi tense the thoracodorsal fascia, which facilitates the force closure mechanism through the SIJ. ‘In optimal gait, the unlocking of the SIJs allows for slight mobility which dissipates some of the rotational force away from the lumbosacral junction and facilitates shock absorption within the pelvis. The locking of the SIJs facilitates stability during times of high load.’
• Vleeming & Stoeckart (2007) describe what happens next, as some of the gluteal tension is transferred into the lower limb via the iliotibial tract. ‘In addition, the iliotibial tract can be tensed by expansion of the huge vastus lateralis muscle during its contraction … during the single support phase, this extensor muscle is active to counteract flexion of the knee.’ This protects the knee from forward shear forces.
• As the single support phase ends and the double support phase starts, there is a lessened loading of the SI joints and gluteus maximus reduces its activity and as the next step starts, the leg swings forward and nutation (see p. 307) at the SI joint starts again.
• Therefore, there is (or there should be) a remarkable synchronicity of muscular effort during the gait cycle, which combines with the role of ligamentous structures to form supportive slings for the joints, such as the SIJ, knees and ankles, as well as to act as energy stores. Within this complex framework of activities there is ample scope for dysfunction should any of the muscular components become compromised (inhibited, shortened, restricted, etc.) (Fig. 3.8).
Lee (2004) provides an insight into the potential disasters that await.
Optimally, the center of gravity should travel along a smooth sinusoidal curve both vertically and laterally and the displacement in both planes should be no more than 5 cm (Inman et al 1981). This displacement is exaggerated when the pelvic girdle is unable to transfer load (insufficient in either form closure or force closure) (Lee 1997a). The patient attempts to compensate by reducing the forces through the pelvic girdle. In a fully compensated gait, the patient transfers weight laterally over the involved limb (compensated Trendelenberg), thus reducing the vertical shear forces through the SIJ.
An alternative possibility exists in which overuse of (and the possible presence of trigger points within) the hamstrings may be part of a natural SIJ stabilizing attempt. Lee (1997b) points out that in response to a need for enhanced stability of the SIJ, the hamstrings may be overused, thereby increasing tone and shortening. However, because the hamstrings ‘are not ideally situated to provide a force closure mechanism’, ultimately this compensation is likely to fail, leading to hypermobility of the SIJ.
Energy storage during gait
Vleeming & Stoeckart (2007) describe how elastic energy is stored by muscles when they are in active tension. This stored energy can be utilized if a muscle placed under applied tension is allowed to relax and shorten. The example is given of actively and forcefully extending a single finger maximally and then releasing it. They report that differing views exist regarding energy storage during gait. One model holds that the process occurs in the tendons as ‘elastic strain’, most specifically in the extensor muscles of the knee and ankle, which act as ‘springs’ (Alexander 1984). Another perspective is that fascia acts as the energy storage site (Vleeming & Stoeckart 2007).
Vleeming et al (2007) question both these views as being inadequate explanations, particularly in relation to attempts to separate the muscles, tendon and fascia, which functionally work together. Instead they promote a view that the myofascial chains, such as the longitudinal and oblique slings described previously (involving biceps femoris?peroneus longus?tibialis anterior and/or latissimus dorsi–thoracolumbar fascia–gluteus maximus, etc.), coupled as they are to the stabilization of the SIJ, offer effective energy storage systems that can reduce demands on muscular action during walking. They observe (1997): ‘…activities such as strolling inadequately energize the slings. This could be the reason why shopping is such a hardship for many people’.
Gracovetsky (1997) has developed a model that describes energy transference during the gait cycle.
• …gait is the result of a sequential transformation of energy.
• Beginning with the legs, muscular chemical energy is first used to lift the body into the earth’s gravitational field where the chemical energy is stored in a potential form.
• When the body falls downwards, this potential energy is converted into kinetic energy, which is in turn stored into a compressive pulse at heel strike.
• The pulse, properly filtered by the knees and the massive ligamentous structures across the SIJ, travels upwards.
• The energy is then distributed to each spinal joint to counterrotate pelvis and shoulder, while the head is stabilized by derotating the shoulders.
Wallden (2009) has described energy storage during the gait cycle as follows:
When the movement of the hands meets resistance from the elasticity of the supporting musculature and connective tissue, an elastic recoil begins to swing the arm in the opposite direction, thus counterbalancing the forward swing of the ipsilateral leg as it enters its swing phase. (See Fig. 3.9.)
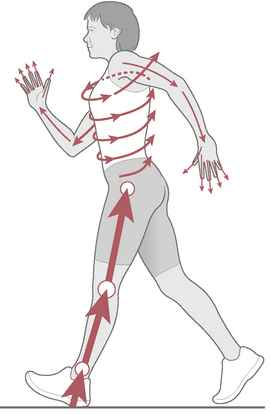
Figure 3.9 A schematic representation of energy storage and transfer during gait.
From: Journal of Bodywork & Movement Therapies 13(4):350–361, 2009.
A detailed accounting of joint and segment motion during the gait cycle can follow each joint through its motions during initial double support, followed by single support and, finally, through terminal double support. A detailed analysis of normal motion throughout the support phase is shown in Table 3.2, which also compares these movements with the condition of functional hallux limitus (Fhl), which is discussed further on p. 71 and p. 79.
Potential dysfunctions in gait
Evaluation of static posture and gait characteristics and analysis of seated postures, reclining postures, and habits of use provide information that is critical to the development of strategic treatment and home care programs. The value of a perspective which evaluates the whole body moving in a normal manner, as in gait analysis when walking, is that it focuses on global features, such as crossed syndrome patterns (see Chapter 10), rather than local ones, such as assessment of individual joints or muscles (for restrictions, shortness, strength, presence of trigger points, etc.), which is performed separately.
Janda (1996) provides a useful caution:
Kuchera et al (1997) suggest that the key elements of normal gait should involve:
• weight transferred in a continuous manner from heel to toe, for push-off
• correct angle of toe orientation, with no toeing in or out
• no evidence of excessive supination or pronation
• symmetrical motion through the pelvis, lumbar and thoracic regions, and the shoulders
Both stance and swing periods will have their own inherent potential problems, the characteristics of which help pinpoint the etiology of the patient’s condition. Hoppenfeld (1976) cites Inman (1973) with these measurable determinants.
1. The width of the base (distance between the two heels when walking) should be no more than 5–10 cm (2–4 inches) from heel to heel, with a wider base usually indicating unsteadiness (perhaps cerebellar), dizziness or decreased sensation of the sole of the foot.
2. The center of gravity for the body as a whole (not just the passenger unit) lies 5 cm (2 inches) anterior to the second sacral vertebra (S2) and should oscillate no more than 5 cm (2 inches) vertically in the normal gait.
3. Except in heel strike, the knee should remain flexed through the stance phase. Locked or fused knees create excessive vertical displacement of the center of gravity [Perry (1992), Cailliet (1997) and others, note the knee reextends toward the end of the stance period.]
4. The pelvis and trunk shift laterally to center the weight over the hip of the supporting limb. This shift is approximately 2.5 cm (1 inch) to each side but may be markedly accentuated when gluteus medius is weak.
5. The average length of a step when walking is 26 inches (65cm) for females and 28 in (70cm) for males, but this may decrease with pain, aging, fatigue or lower extremity pathology in hip, knee and/or ankle joints. [Others (Rose & Gamble 2006, Perry 1992) note a much longer average step length of 70 cm (28 inches). The step is approximately 14% longer for men, generally shorter in people who have shorter legs, as easily observed in children attempting to ‘keep up’ when walking with adults, and is influenced by speed.]
6. The average adult spends only 100 calories per mile and walks with a cadence of 90–120 steps per minute. Pain, aging, fatigue, slick surfaces and unsure footing may decrease the number of steps per minute.
7. During swing phase, one side of the pelvis rotates 40° forward with the swinging leg, thereby requiring normal rotation around the hip of the fixed stance leg, which acts as a fulcrum for that rotation (see Fig. 3.10 and Box 3.5).
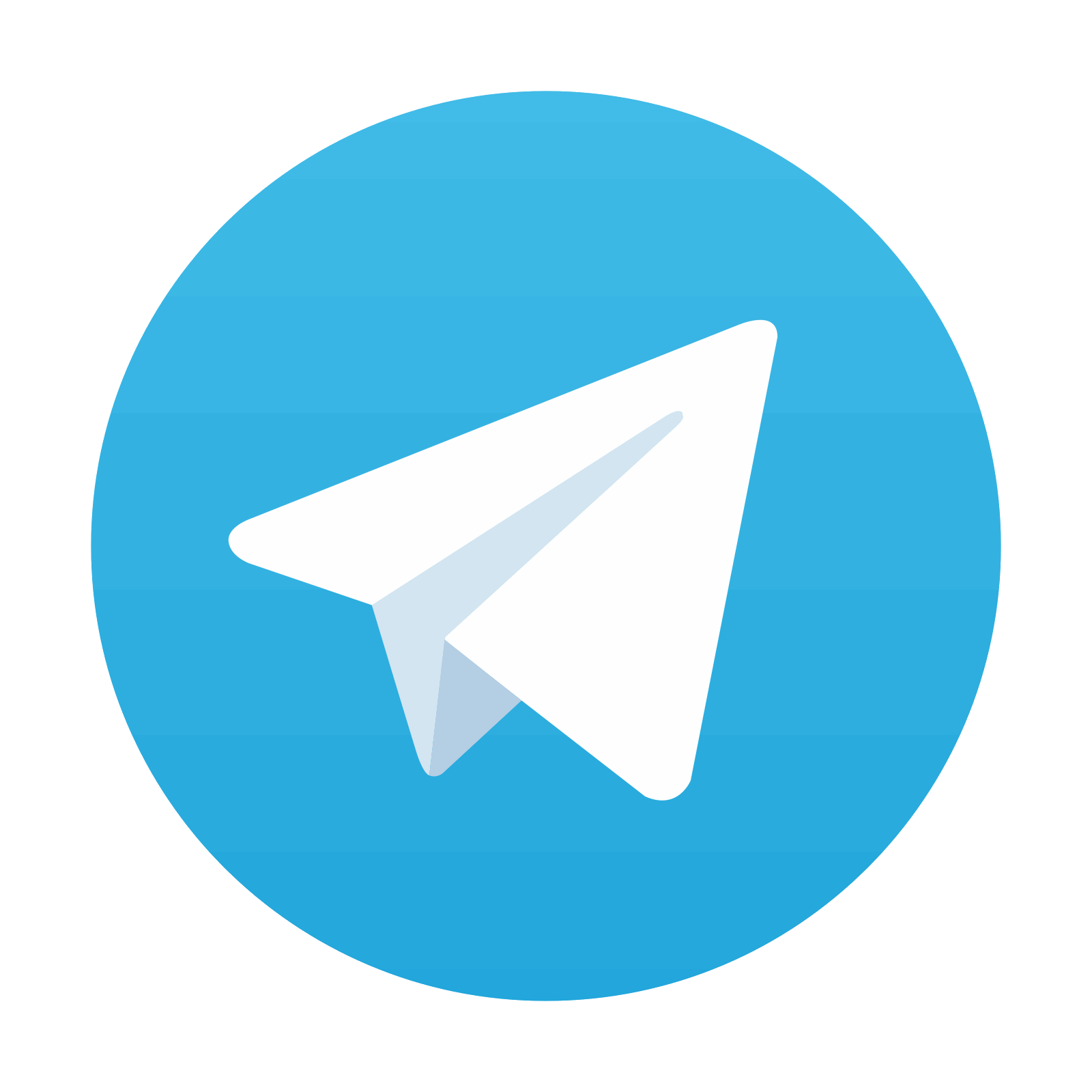
Stay updated, free articles. Join our Telegram channel

Full access? Get Clinical Tree
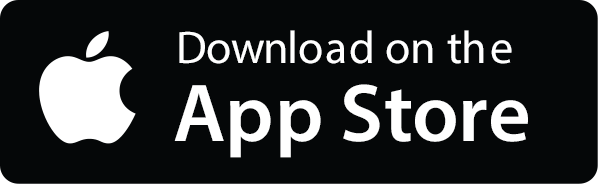
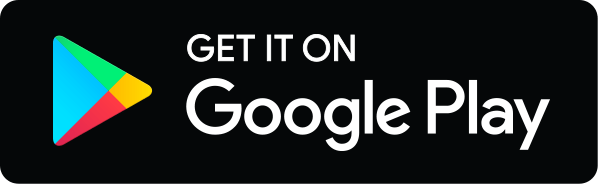