Gait and balance disorders are common and a significant source of disability, decrease in quality of life, and falls. A description of the gait cycle and gait parameters is offered as a starting point of understanding gait abnormalities. Gait patterns such as parkinsonian gait, disequilibrium, high-level gait disorders, and the neural control of gait are discussed. This may help in the evaluation of individuals with abnormalities of gait and balance and allow to clinically localize the pathological process in the neuraxis or establish a non-neurological etiology. Recommendations regarding prevention of falls, evaluation of patients with gait difficulties, and management strategies to minimize disability and potentially increase quality of life are offered.
Walking is often perceived as a simple task; we stand up and initiate walking without giving it much thought. However, this is actually a very complex motor skill with well-choreographed interplay of multiple anatomical structures including locomotor centers at the spinal cord, brainstem and cerebellum, basal ganglia, frontal lobes, the musculoskeletal system, and sensory inputs from visual, vestibular, and proprioceptive pathways.1 Consequently, any disruption to the multiple parts involved in generating and maintaining a normal posture and gait could be associated with a gait disorder.
Gait and balance disorders are common and an important cause of disability and diminished quality of life especially among older adults. It is estimated that about 20% of noninstitutionalized older adults have difficulty walking or require an assistive device or someone’s help in order to ambulate.2
The prevalence of gait difficulties among individuals older than 85 years has been estimated to be as high as 50%.3 One third of individuals over 65 may fall at least once a year;4 25% of the elderly with falls suffer a serious injury; and 5% have a fracture.5 The incidence of falls in hospitals and nursing facilities are almost 3 times the rates for community-dwelling people over 65 years of age. The incidence of injuries and fractures related to falls is also higher among institutionalized individuals.6
CASE 29-1
A 68-year-old man with multiple vascular risk factors, rheumatoid arthritis, Sjögren syndrome, and Raynaud’s phenomenon presented to an outside hospital 3 years earlier with sudden onset of left-sided paresthesias and imbalance, veering to the left side. He was found to have multiple small right hemispheric ischemic strokes, he had good neurological recovery, and 6 weeks after the index event, his gait was normal. Brain imaging studies showed extensive periventricular white matter microangiopathic changes. He was neurologically stable until 3 months prior to his consultation with us, when he had a partial lung resection due to a nonmalignant pulmonary mass. After surgery, he noticed difficulty with balance, 15-pound weight loss, and diminished stamina. He also experienced decreased hearing in both ears and was evaluated by ENT who recommended vestibular rehabilitation. He continued to have walking difficulties and fell on rare occasions. He then started to use a cane for ambulation and eventually progressed to a walker. The family reported he was becoming increasingly more unsteady on his feet, and he had experienced some urinary incontinence, but no cognitive difficulties. On neurological examination, muscle strength was normal with mild impairment of proprioception. Gait was cautious, wide-based, and unsteady with inability to keep his balance with his eyes open. A head CT showed enlarged lateral and third ventricles, out of proportion to the degree of cerebral atrophy. Ten days later, he presented with left-sided weakness, unconsciousness, and seizures. A repeat head CT showed a right subdural hematoma requiring surgical evacuation. Following surgery, his left-sided weakness improved and he had no recurrent seizures. However, his gait and station continued to be severely affected with some magnetic features. Review of prior brain imaging studies showed increasing ventricular size in the last 6 months. He underwent high-volume (therapeutic lumbar puncture LP) followed by ventriculo-peritoneal (VP) shunt placement. Few weeks after VP shunting, he had a remarkable improvement of his gait and resolution of his urinary incontinence.
Gait is understood as the pattern of how a person walks. There is no accepted definition of what is an “abnormal” gait due to lack of agreement on what a “normal” gait is. It is assumed that any deviation from a smooth, synchronous, symmetric, and even esthetically pleasant gait could represent an abnormal gait.7
Balance could be described as the ability to keep the stance against the forces of gravity without falling, while gait could be described as cyclic, patterned movements of the limbs associated with the advancement in space, that is, locomotion. Postural control is required for effective locomotion and to assure dynamic stability. To assure stability while walking, coordinated locomotor patterns have to be produced; these include the appropriate relationship of body segments to the environment and to one another. In addition, dynamic postural control while walking requires the interplay of goals, environmental factors, biomechanics, and sensory integration.8
Balance and gait disorders are often grouped together despite different pathophysiologies. Grouping them together makes clinical sense as abnormalities of balance and gait are consistent robust predictors of future falls. Balance and gait disorders almost always coexist, and thus, traditionally are discussed together. In addition, the distinction between balance and gait might not help to decode the so-called dynamic balance, understood as postural changes that precede locomotion, turning, obstacle avoidance and stopping among others. Conversely, considering balance and gait as separate but complementary processes also makes clinical sense, as balance deficits are more closely related to falls than gait disturbances are.9 In this chapter, we consider balance and gait disorders together.
The gait cycle can be divided into a stance phase and a swing phase. The stance phase represents the time when the foot is on the ground and the swing phase the time when the foot is in the air. About 60% of the gait cycle is in the stance phase, with 10% of the time spent in bipedal support. In addition, the stance phase can be divided into initial double-limb support, single-limb stance, and second double-limb support. The swing phase is divided into initial swing, mid swing, and terminal swing (Figure 29-1). Gait parameters are shown in Table 29-1.10 Gait velocity and step length are lower among women, while step frequency, that is, cadence, is higher among women. In advanced age, the most commonly affected gait parameter is a reduction of gait speed and step length and not much change of the cadence.11
Gait Parameters10
Stance phase | When the foot is on the floor |
Swing phase | When the foot is in the air |
Stance time | The time when the foot is on the floor |
Swing time | The time when the foot is in the air |
Cadence | Number of steps per minute |
Step length | Distance advanced by one foot compared to the position of the other |
Stride length | The sum of 2 consecutive step lengths or the distance advanced by one foot compared to its prior position |
Step time | Time between heel strike of one foot to heel strike of the other foot |
Gait cycle | The time between 2 consecutive heel strikes of the same foot |
Stride time | Time for a full gait cycle |
Average gait velocity | Stride length divided by stride time |
Gait and balance can be considered as a three-level system in which spinal cord locomotor pattern generators are controlled by cortical–basal ganglia–brainstem circuits while receiving sensory inputs from visual, vestibular, and proprioceptive pathways (Figure 29-2).1 Clinical classification of gait disorders has been based in a hierarchical anatomical system as proposed by Nutt, Marsden, and Thompson.12 According to these authors, gait disorders can be classified as low-level, middle-level, and high-level disorders. The analogy with a balance/walking “building” in which each level of the locomotor system from the cortex to the muscle could be understood as a “floor” is a didactic way of approaching gait disorders (Table 29-2).3 However, non-neurological conditions impacting balance and walking should be taken into account, as not all balance and gait disorders are related to neurological disease. Factors that slow gait speed, such as aerobic function, deconditioning, decreased leg strength, or joint impairment, may contribute to gait disorders, especially while in combination.7 What we observe as a gait disorder is in reality a combination of a primary dysfunction of the locomotor system and compensatory mechanisms. The compensatory mechanisms can be so efficient that the deficit can only become apparent when the system is maximally challenged (eg, adding a complex secondary task while the patient is walking or balancing; worsening gait of a parkinsonian patient suffering an intercurrent infection).9
Figure 29-2
Summary of important areas involved in human locomotion derived from functional imaging studies.1 CLR, Cerebellar locomotor region; CPG, central pattern generator; DLPFC, dorsolateral prefrontal cortex; PMC, primary motor cortex; PMRF, pontomedullary reticular formation; MLR, messencephalic reticular formation; SMA, supplementary motor area.

Anatomical Structures and Associated Gait Patterns in the Balance/Walking “Building”
Anatomical Level | Levels | Floors | Balance and Gait Pattern |
---|---|---|---|
Psychological/psychiatric | 10+ | Variable: slow, buckling knees | |
Cortex | Higher level | 10 | Different patterns: cautious, parkinsonian, ataxic, spastic, magnetic, gait ignition failure, disequilibrium |
Subcortical | Higher level | 9 | |
Basal ganglia | Middle level | 8 | Parkinsonian/dystonic/choreic |
Thalamus | Middle level | 7 | Astasia/ataxia |
Cerebellum | Middle level | 6 | Cerebellar ataxia |
Brainstem | Middle level | 5 | Ataxia/spasticity |
Spinal cord | Middle level | 4 | Spastic gait/tabetic gait |
Peripheral nerve Proprioception, vestibular, visual | Lower level | 3 | Sensory ataxia/vestibular disequilibrium/visual disequilibrium |
Neuromuscular junction | Lower level | 2 | Waddling |
Muscle | Lower level | 1 | Waddling, steppage, Trendelenburg |
Skeleton | 0 | Antalgic/compensatory for deformities |
The three-level system of locomotion generation and control requires anticipatory postural adjustments mediated by the corticoreticulospinal system. The three levels include: (I) the spinal central locomotor pattern generator (CPG); (II) the brainstem locomotor regions, basal ganglia output and its descending pathways; and (III) the control from the cerebral cortex (Figure 29-3).13
Spinal locomotor CPG. Spinal cord preparations generate reciprocal burst of activity in flexor and extensor muscles, which mutually inhibit each other. This rhythmic activity is transmitted to second-order interneurons (Laminae IV to VII of Rexed), and lamina VIII interneurons project to the contralateral side to contribute to the right-left alternation of limb movements. The activity of the spinal cord locomotor centers is modulated by sensory afferents including proprioception feedback and skin afferents; the skin afferents have a very strong influence on the CPG.
Brainstem structures involved in balance and locomotion. Three areas linked to locomotion, at least identified in animals, are the mesencephalic locomotor region (MLR), the subthalamic locomotor region (SLR), and the cerebellar locomotor region (CLR). The MLR is located mainly in the cuneiform nucleus, by the pedunculopontine nucleus (PPN) that receive inputs from the prefrontal cortex (Figure 29-2). Excessive GABAergic inhibitory effects from basal ganglia over the PPN/MLR maybe the pathophysiological basis for gait disturbances and increased tone in Parkinson disease (PD). The ventromedial reticular formation (VMRF) acts as a rhythm generating system that activates the spinal CPG as well as increase muscular tone. The SLR may contribute to emotional motor behaviors and activates the rhythm generating system through the MLR. The CLR is located in the medial aspect of the cerebellar white matter and appears to activate locomotor rhythm generation through the MLR. Muscle tone is influenced by both inhibitory and excitatory pathways at the brainstem level (Figure 29-4).
Effects of the cerebral cortex in gait generation and modulation. The premotor (PM) and the supplementary motor area (SMA) are very important anatomical structures in gait initiation. The SMA may be important for postural control, and the PM cortex may be responsible for sensory guided gait initiation. SMA and PM send multiple projections to the pontomedullary reticular formation and the spinal cord. Conversely, the temporoparietal cortex integrates signals from visual, vestibular, and proprioceptive inputs that help the generation of motor programs by the PM and SMA (Figure 29-5).
Figure 29-3
Fundamental signal flows involved in gait control. (A) Schematic illustrations of basic signal flows involved in gait control. Sensory signals acting on the cerebral cortex and limbic system generate “volitional and cognitive reference” and “emotional reference,” respectively. The volitional process requires cortical information processing. Projection from the limbic system to the brainstem is responsible for emotional processes. The brainstem and spinal cord are involved in automatic processes. The basal ganglia and the cerebellum control volitional and automatic processes by thalamocortical projections and by direct projections to the brainstem, respectively. See text for further explanation. Reproduced with permission from Takakusaki K. Neurophysiology of gait: from the spinal cord to the frontal lobe. Mov Disord. 2013;28(11);1483–1491.

Figure 29-4
Brainstem mechanisms of controlling postural muscle tone and locomotion in cats. (A) Signals from the MLR activate muscle-tone excitatory and rhythm-generating systems. The rhythm-generating system is from the excitatory reticulospinal tract arising from the ventromedial MRF (v-MRF) and CPG in the spinal cord. The excitatory reticulospinal tract also operates as the muscle-tone excitatory system as well as the coerulospinal tract from the locus coeruleus (LC) and raphespinal tract from the raphe nuclei (RN). Signals from the SLR and those from the CLR activate the rhythm-generating system to evoke locomotion. (B) Cholinergic neurons in the pedunculopontine nucleus (PPN) activate the muscle-tone inhibitory system, which is composed of the pontine reticular formation (PRF) neurons, inhibitory reticulospinal neurons descending from the dorsomedial MRF (d-MRF), and lamina VII inhibitory interneurons in the spinal cord. Signals from the limbic system act on the muscle-tone inhibitory system through the PPN. GABAergic basal ganglia output from the internal segment of the globuspallidus (GPi) and the substantia nigra reticulata (SNr) to the MLR/PPN controls locomotion and muscle tone. See text for further explanations. 5-HT, serotonin; α, alpha-motoneurons; ACh, acetylcholine; E, extensor motoneurons; F, flexor motoneurons; γ, gamma–motoneurons; Hypoth, hypothalamus; NA, noradrenaline. Reproduced with permission from Takakusaki K. Neurophysiology of gait: from the spinal cord to the frontal lobe. Mov Disord. 2013;28 (11);1483–1491.

Figure 29-5
Cortical mechanisms involved in movement control. Motor programs of precise movement and postural control are generated in the premotor area (PM) and the supplementary motor area (SMA). Descending signals from these areas to the brainstem by the corticoreticular projection may contribute to anticipatory postural adjustment by activating the reticulospinal tract. Motor command for precise limb control during locomotion is carried by the corticospinal tract arising from the primary motor cortex (M1). Somatosensory, vestibular, and visual sensations are integrated at the temporoparietal–posterior parietal cortices, where the body schema is generated and updated. This bodily information is transmitted to the PM/SMA and utilized to generate motor programs. S1, primary sensory cortex. Reproduced with permission from Takakusaki K. Neurophysiology of gait: from the spinal cord to the frontal lobe. Mov Disord. 2013; 28(11);1483–1491.

Skeletal gait disorders are mainly related to pain and skeletal deformities, including the antalgic gait, coxalgic gait, knee hyperextension gait, knee flexion contracture gait, knee extension contracture gait, stooped gait of lumbar spinal canal stenosis, and inadequate hip extension gait (Table 29-3).5
Description of Musculoskeletal Gait Disorders
Gait Type | Characteristics | Notes |
---|---|---|
Antalgic gait | Decreased time in the stance phase on the affected limb | |
Coxalgic gait | Shifting of the upper torso toward the painful hip during the single limb stance on the affected hip, the so-called “abductor lurch.” There is no hemipelvis drop | Trendelenburg gait: the contralateral hemipelvis drops during the single limb stance phase on the affected side due to hip abductor weakness (“painless coxalgic gait”) |
Knee hyperextension gait | Results from ankle equinus contracture or from quadriceps deficiency | |
Knee flexion contracture gait | Inadequate knee extension with increased compensatory ankle dorsiflexion and/or premature heel rise | |
Knee extension contracture gait | Affects mainly the swing phase, and to compensate, the ipsilateral hip may be abducted to circumduct the limb. May be associated with leaning of the trunk to contralateral side | Hemiparetic gait shows some similar features |
Inadequate hip extension gait | Reduces stride length and the duration of single limb support. Exaggerated lumbar lordosis is used to compensate for deficit in hip extension | |
Stooped gait of lumbar stenosis | Caudaequina compression with pain exacerbated by standing, walking, and being erect. Improves by sitting down or leaning forward that decreases lumbar epidural pressure |
Steppage gait. Steppage gait is typically observed in patients with a foot drop or weak foot dorsiflexion related to peroneal nerve injury, radicular compromise, or a demyelinating neuropathy. For the toes to clear the ground and avoid tripping, an exaggerated knee and hip flexion on the affected leg is required.14
Trendelenburg and waddling gaits. In Trendelenburg gait, hip abductor weakness causes ipsilateral lurching of the torso and hip drop on the contralateral side with single leg stance on the affected side.5 The waddling gait pattern can be seen with bilateral hip girdle muscle weakness, in particular the gluteus medius, causing excessive hip drop and alternating lateral trunk tilting. Waddling gait can also be seen in patients with bilateral orthopedic hip problems.
Sensory ataxia. The most striking feature of ataxic gait is the variability in step length, width, and gait cycle time15 with associated unsteadiness and increased base. This type of gait disorder is not specific for a particular location in the neuraxis, as it can be seen with proprioceptive deficits peripherally, posterior columns (sensory ataxic disorders), cerebellar dysfunction, and pontine and thalamic pathologies.3
Visual disequilibrium. This is associated with a sensation of being off balance and associated cautiousness. Acute visual distortions may cause a visual ataxia with broad-based support and tentative steps.14
Vestibular ataxia. Acute vestibular dysfunction may cause instability and a tendency to veer or fall toward the affected side, with associated lateropulsion. The gait tends to be cautious, mildly wide-based, and the patients exhibit difficulties with Romberg maneuver and tandem.3
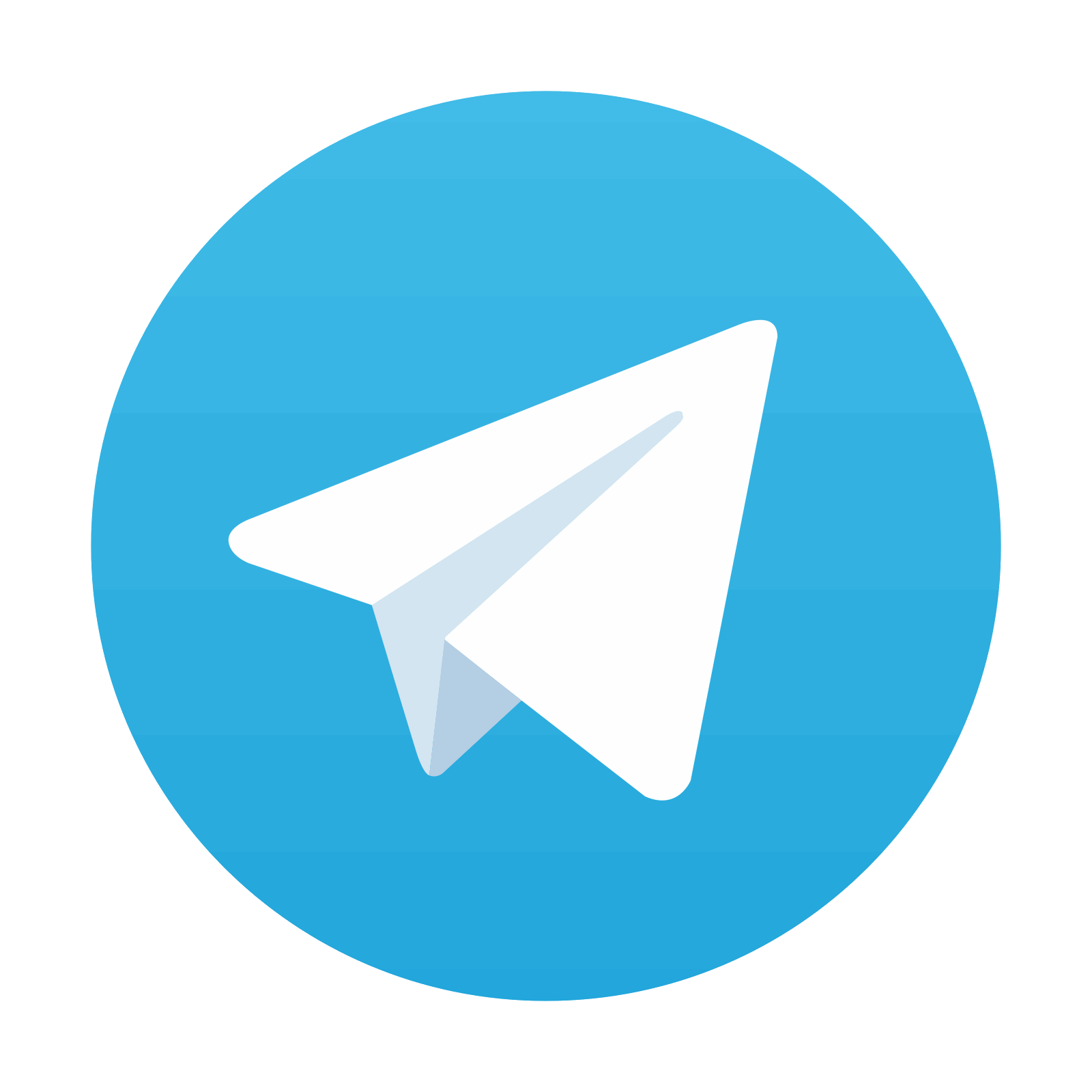
Stay updated, free articles. Join our Telegram channel

Full access? Get Clinical Tree
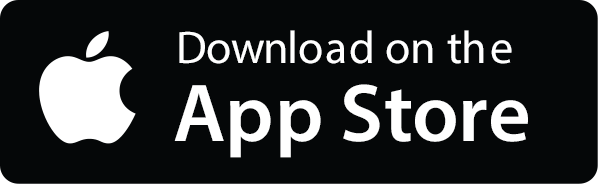
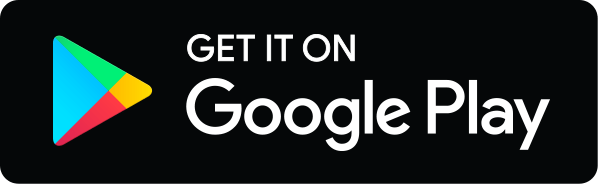