16 Gamma Knife Ronald F. Young Lars Leksell developed the idea of radiosurgery specifically for the performance of functional neurosurgical procedures. He sought a less-invasive technique to create lesions within the brain to treat a variety of neurological disorders, including chronic pain, Parkinson disease, and trigeminal neuralgia. In his seminal report in 1951, in which he coined the term radiosurgery, he specifically referred to the performance of thalamotomy using a radiosurgical technique.1 His search for the ideal instrument—which was simple, accurate, reliable, and could be used as a neurosurgical tool—led him through a series of prototypical instruments that employed a variety of radiation sources. He eventually settled on the multisource, fixed position, cobalt-60 gamma unit called the Gamma Knife (Elekta, Inc., Norcross, GA). Several early publications documented the experience of Leksell and his colleagues with functional neurosurgical procedures with the Gamma Knife. With the development of Gamma Knife radiosurgery in the United States in the late 1980s, the emphasis was primarily on the treatment of vascular malformations and brain tumors. Beginning in the early 1990s, there was a resurgence of interest in functional neurosurgery with the Gamma Knife to treat a variety of disorders, including chronic pain, Parkinson disease and other movement disorders, trigeminal neuralgia, epilepsy, and certain psychoneuroses. This author’s personal experience includes more than 500 patients who have undergone Gamma Knife lesioning procedures for the treatment of movement disorders in addition to over 500 more who have undergone radio frequency lesioning procedures, deep brain stimulation (DBS), and, for a time, neurotransplantation, in addition to radiosurgical lesioning. The Gamma Knife procedures were performed at two centers, one located at the Northwest Hospital in Seattle, Washington, and the other at Good Samaritan Hospital in Los Angeles, California. At both centers, multidisciplinary interventional techniques are available for the treatment of movement disorders. Our selection criteria for radiosurgical lesioning are identical to those for open surgical procedures except as outlined subsequently under “Specific Indications.” Preoperative mental status examination is performed in all patients, and dementia is considered a contraindication to any form of surgical intervention. The lesions, in our experience, were made initially with the Model U, 201 Source Gamma Unit and more recently with the Model C. The unit consists of a sealed “central body,” which contains 201 Co 60 sources in a hemispheric array. A set of primary collimators within the central body forms the gamma rays into beams. To perform radiosurgical lesioning procedures for treatment of movement disorders, the patient’s head is fixed in a Leksell stereotactic frame (Model G, Elekta Inc.), which is positioned within a secondary collimator helmet. The secondary collimator helmet forms the 201 gamma rays into beams 4 mm in diameter, such that all beams are focused on the same fixed point. The target for lesioning is placed at the focal point by application of the calculated x, y, and z stereotactic coordinates of the planned lesions. The theory of the Gamma Knife is that the radiation dose along any single gamma ray beam is too low to produce neural injury, but at the focal point the radiosurgical dose represents the summed dosage of all 201 beams. The beam profile of the 4 mm secondary collimator helmet is such that the 10% isodose line (14 Gy for radiosurgical lesioning) encompasses a volume of only ~500 mm3. Thus the radiation exposure of tissue outside the intended lesion is minimal. Radiosurgical lesioning has frequently been proposed only for patients who do not meet the criteria for any form of open surgical procedures for the treatment of movement disorders (e.g., lesioning or DBS). Such conditions include chronic anticoagulation, coagulopathies, cerebral atrophy, inability to understand or follow commands that might be given during surgery, and advanced age. Based on our experience, however, we believe that radiosurgical lesioning can be discussed as an alternative for any patient with a movement disorder who is a candidate for a surgical procedure for treatment of movement disorders. There are two specific indications for radiosurgical lesioning. These are tremor and levodopa-induced dyskinesias. Patients with parkinsonian, essential, or familial tremor or tremor due to conditions such as multiple sclerosis, cerebral infarction, cerebral infections, and cerebral trauma are considered possible candidates for radiosurgical thalamotomy. Parkinsonian patients who exhibit a major difficulty with levodopa-induced dyskinesias, but who do not have significant difficulties with motor fluctuations and gait and balance disturbances, may be considered candidates for radiosurgical pallidotomy. For patients whose primary problems include motor fluctuations, bradykinesia, rigidity, and particularly gait and balance problems, we believe that currently DBS in the subthalamic nucleus is a better form of surgical treatment than is lesioning, regardless of whether one uses the radiofrequency technique or radiosurgery with the Gamma Knife. As with the radiofrequency thalamotomy, we perform unilateral thalamotomy with the Gamma Knife as an initial procedure. Although bilateral radiofrequency thalamotomy has been associated with a high incidence of complications, including speech and swallowing difficulties, cognitive disorders, and akinesia, we have not seen these complications with bilateral radiosurgical thalamotomy in a series of 22 patients (six with Parkinson disease and 16 with essential tremor). This is conditional on the interval between the two procedures being at least 1 year apart and that no complications or side effects present after the unilateral procedure. In addition, the MRI scan must demonstrate a lesion of the expected size that is properly located anatomically and without significant surrounding perilesional changes. We have performed bilateral pallidotomy in 12 patients with Parkinson disease; in four patients the lesions were performed on the same day, and in the others with intervals of 1 year or more. Currently, we would not recommend that bilateral pallidotomy be performed on the same day and, as with bilateral thalamotomy, we recommend an interval of at least 1 year between bilateral lesions and MRI confirmation that the first lesion is as normally expected before proceeding to a contralateral pallidotomy. Overall, the mean length of follow-up for patients who underwent bilateral lesioning (thalamotomy and pallidotomy) is 45 months from the second procedure. The primary criticism of Gamma Knife lesioning for treatment of movement disorders is the lack of any method to electrophysiologically corroborate the correct targeting. Typically, in open stereotactic procedures for the treatment of movement disorders, a combination of electrophysiological procedures, including microelectrode recording, microstimulation, and/or macrostimulation, have been employed. In addition, with radio frequency lesioning it is possible to gradually enlarge the size of the lesion and examine the patient as the procedure moves forward to ascertain an end point that includes maximum resolution of preoperative symptoms and minimal or no side effects. This type of monitoring is impossible with radiosurgical lesioning in which the procedure is closed and the targeting is based strictly on anatomical localization. For many years, a controversy has existed regarding whether the target for lesioning for the treatment of movement disorders is an anatomical or physiological target. Even if the target is agreed to be anatomical, there has been additional controversy regarding the ability of MRI stereotactic localization to accurately identify the target. Concerns about MRI distortion have been expressed because it relates to purely anatomical target localization. We have reviewed and discussed this point in previous publications.2–4 We believe that, with detailed attention to the stereotactic MRI technique, imaging distortion can either be eliminated or corrected for, so that anatomical target localization is sufficient to accurately locate an intended target for radiosurgical lesioning In addition, Gamma Knife lesioning does not allow for the lesion to be gradually made and the patients’ responses, either positive or negative, assessed. Once the radiosurgical dose is delivered and the response set into motion, there are no external means to control the lesion. The primary cause of complications following radiosurgical lesioning for treatment of movement disorders has been due to lesions that develop larger than expected.5 Complications or side effects due to mistargeting have not been seen in our experience, although when radiosurgical lesioning fails to improve the symptoms it certainly suggests mistargeting. In most situations when symptoms are not improved, however, radiosurgical lesions develop considerably smaller than expected, and this problem seems to account for the majority of instances in which there is a failure to improve symptoms. We usually do not recommend a repeat radiosurgical procedure to enlarge a small lesion, due to the unpredictable consequences in terms of lesion size for superimposed or closely spaced lesions. Instead, for patients who fail a radiosurgical procedure, we recommend either enlarging the lesion by radio frequency methods or employing a DBS procedure instead. Finally, there has been considerable concern about long-term radiation complications following radiosurgical lesioning. The Gamma Knife dose profile is extremely steep, however, and the dose of radiation delivered to the majority of the brain is extremely small. Generally, any complications of a radiosurgical lesioning procedure for the treatment of movement disorders appear ~6 to 12 months following the procedure. However, occasionally symptoms may develop earlier, or in some cases substantially later, than this interval. We have attempted to place our radiosurgical lesions for the treatment of tremor in the ventral intermediate thalamic nucleus, and we have utilized the Schaltenbrand and Wahren atlas as a guide to target localization.6 The procedure begins by application of the Leksell model G stereotactic frame (Electa, Inc., Norcross, GA). We apply the frame with the patient in the sitting position. Mild intravenous sedation (midazolam 1 to 2 mg and fentanyl 50 to 100 μg) is usually employed for patient comfort. Five milliliters of 1% Xylocaine solution (AstraZeneca Pharmaceuticals LP, Wilmington, DE) is injected into the scalp at each of the four pin placement sites to provide local anesthesia. We attempt to place the stereotactic frame as accurately as possible, to avoid errors due to pitch, roll, and yaw. We place the base of the frame parallel to the line extending from the external auditory canal to the floor of the orbit. This line generally corresponds to the trajectory of the anterior commissure (AC)–posterior commissure (PC) line. In our current protocol, we employ a Siemens MAGNETOM Symphony 1.5 tesla magnetic resonance imaging (MRI) scanner (Siemens Medical Solutions USA, Inc., Malvern, PA). A series of axial scans is performed utilizing the protocol, as described in Table 16.1. The axial magnetization prepared rapid gradient echo (MPRAGE) scan is used to localize the AC and PC, aided by sagittal reconstruction images. Two series of short tau inversion recovery (STIR) images are obtained through the area of interest only. Slice thickness for these two sequences is 2 mm with a 2 mm gap. The two series of images are then interleaved to give a contiguous set of scans without any interslice gap. The images are transported via Ethernet to the Leksell Gamma Plan (Elekta Inc., Norcross, GA) UNIX-based computer workstation for Gamma Knife target planning. A mathematical formula is then used to correct the images for pitch, roll, and yaw errors. To develop our computer algorithm to correct for errors in frame placement, we assumed that the correlation between Leksell stereotactic coordinates and the reference system of the AC—PC plane as utilized in the Schaltenbrand and Wahren Stereotactic atlas could be viewed as the relationship between two orthogonal coordinate systems. This relationship is mathematically defined by the distance between the origins in each dimension and the relative rotation about each axis. The origin of the Leksell coordinate system is taken at the intersection of the diagonals of the fiducial markers in the axial, coronal, and sagittal planes. The origin of the AC—PC reference system is the midpoint of the AC—PC line in the midsagittal plane. The rotation of the coordinate systems makes use of the Euler angles used in classical physics for the orientation of a rigid body. We developed an in-house computer program, which calculates the target localization independent of the frame position. From the Leksell Gamma Plan, we input the stereotactic coordinates of AC, PC, the target, and the axial, coronal, and sagittal angles of rotation. The output is the AC—PC line length, the distance of the target lateral to the AC—PC line (x coordinate), posterior to the mid-AC—PC point (y coordinate), and above the AC—PC plane (z coordinate). The calculations are verified by comparing the AC—PC length between the Leksell Gamma Plan and our program. The accuracy of this method has been repeatedly demonstrated by two methods. The first being the clinical outcomes, which, in terms of safety and effectiveness, are comparable to open stereotactic techniques that employ intraoperative electrophysiological target corroboration. The second is repeated comparisons of the target coordinates and locations of actual lesions demonstrated on postoperative MRI scans and the coordinates used in planning the procedures. These comparisons have been made by obtaining the postoperative MRI scans with the patient’s head within the Leksell stereotactic frame and the attached fiducial box, but without skeletal fixation. We have also studied the reproducibility of the same observer making repeated calculations of lesion localizations on the same postoperative MRI scan. The difference between pre- and postoperative target coordinates is nearly always within the same range as the interobservation differences on repeated observations of postoperative lesion coordinates (~0.5 to 1.0 mm). The position of the AC and PC is identified on the axial MPRAGE images. The anteroposterior (or y) coordinate is determined empirically. For an average intercommissural distance of 26 mm, we select a y coordinate ~5 to 6 mm posterior to the intercommissural point. A reconstructed coronal MRI scan image at that point is then observed. Utilizing the Gamma Plan computer functions, we place the simulated target in the inferolateral corner of the thalamic mass. The target is placed such that the 50% isodose line of the simulated lesion, utilizing the 4 mm secondary collimator helmet of the Gamma Knife, is exactly at the inferolateral border of the thalamus (Fig. 16.1A,B). The y and z coordinates for the center of the target are then displayed on the Gamma Plan computer.
Indications and Controversies
A Brief History of Radiosurgery for Movement Disorders
Specific Indications
Controversies
Target Selection and Identification
Thalamotomy
Siemens MAGNETOM Symphony 1.5 Tesla Magnetic Resonance Imaging Scanner | ||
MPRAGE Axial | STIR Axial and Coronal | |
No. of slices | 48 | 12 |
Slice thickness | 1 mm | 2 mm |
Field of view (FOV) | 256 × 256 | 256 × 256 |
T1 (inversion time) | 1100 | 140 |
T4 (repetition time) | 1500 | 3190 |
TE (echo time) | 4.1 | 33 |
Nex (No. of excitations) | 1 | 2 |
Resolution | 256 × 256 | 256 × 256 |
Time for scan | 6 minute 26 second | 4 minute 0.1 second |
Abbreviations: MPRAGE, magnetization prepared rapid gradient echo; STIR, short tau inversion recovery.
Pallidotomy
The target for Gamma Knife pallidotomy is the ventral posteromedial (GPi) segment of the globus pallidus. The protocol for stereotactic frame application and MRI scanning is identical to that for thalamotomy. The intercommissural distance is determined utilizing the Gamma Plan as for thalamotomy. The anteroposterior coordinate (or y coordinate) for pallidotomy is selected empirically ~3 mm anterior to the intercommissural point. A coronal image is reconstructed at that point utilizing the Gamma Plan computer, and the target is placed in the internal segment of the globus pallidus, such that the 50% isodose line of the simulated lesion, utilizing the 4 mm secondary collimator helmet of the Gamma Knife is immediately superior to the optic tract and immediately inferolateral to the internal capsule (Fig. 16.2). The x and z coordinates for the center of the simulated lesion are then displayed by the Gamma Plan computer. The same algorithm previously described for thalamotomy is used to correct for errors of pitch, roll, and yaw in stereotactic frame placement.
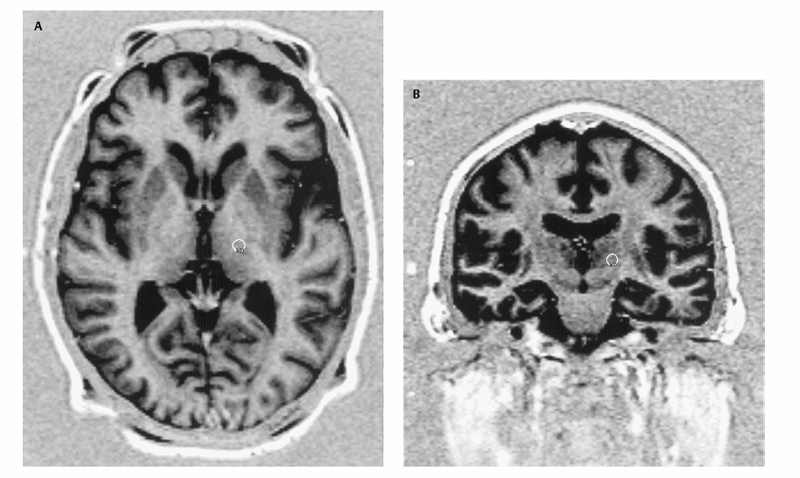
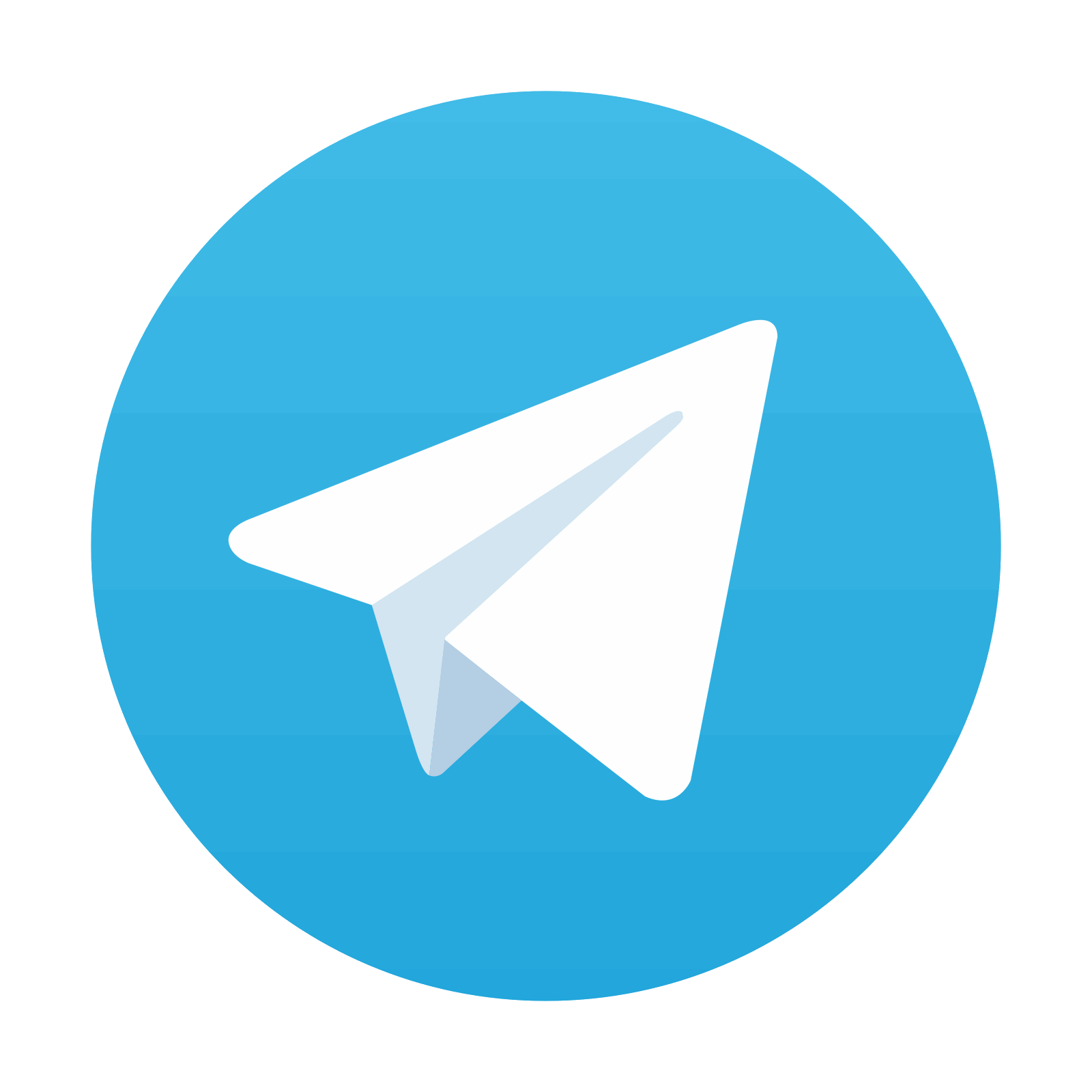
Stay updated, free articles. Join our Telegram channel

Full access? Get Clinical Tree
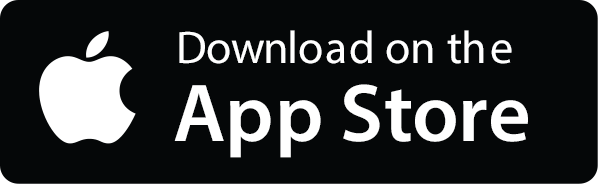
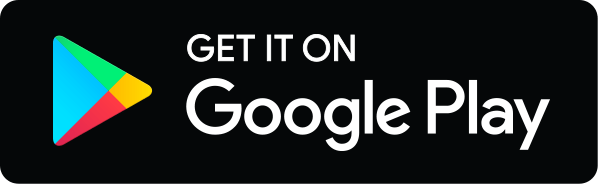