Fig. 1
Behavioral tests for the functional recovery after rubrospinal tract transection. (a) Photo showing the symmetrical use of both forelimbs of the rat during vertical exploration of the cylinder wall. (b) Photo showing the asymmetrical use of the forelimbs 3 days after unilateral RST transection during vertical exploration. Arrow points to the forelimb on the lesioned side of the spinal cord. (c) Photo showing the crossing of the rope-wrapped rod of an unlesioned rat. (d) Photo showing the crossing of the rope-wrapped rod of a rat 3 day after unilateral RST transection
Note (1): The rats may not rear up so frequently after they have got used to the glass cylinder. If not enough rear-ups are recorded, the rat is placed back into its home cage and repeat the session again after a while.
Note (2): Place a mirror on the opposite side of the video camera at a suitable angle so that the camera can capture the positions of the forelimbs when the rat turns its back to the camera.
Modified rope crossing: In this test, rats are to cross a 125 cm long horizontally oriented rope (4 cm in diameter) between two platforms. This task requires hind-limb weight support, precise paw placement, posture and coordinated balance of the body. However, as rats with RST lesion fall easily from the rope due to the swinging of the rope, which may mask small improvement in limb motor function, we modified the device by wrapping a thinner rope around a wood pole to make it rigid (Fig. 1c, d). Unlesioned rats run across the rope very quickly and rarely slip or fall during the run, while lesioned rats cross slowly with foot slips and sometimes fall from the rope. During each run the number of slips and falls is counted and the ratio of slips versus total steps in two runs at each time point for each animal is calculated and presented as error index. The ratio is defined as 1 whenever the rat falls.
3.2.5 Immunohistochemistry and Quantification of RST Axons
1.
Dissecting the tissues. Under deep anesthesia, perfuse the animals with 0.9 % saline first and then with 4 % paraformaldehyde in phosphate buffer. Dissect the midbrain and spinal cord segments containing the lesion site.
2.
Processing the tissues. The dissected tissues are post-fixed in 4 % paraformaldehyde for overnight, and cryoprotected in 30 % sucrose solution until they sink to the bottom. Divide each spinal cord into three blocks, a 10 mm lesion block centered at the lesion site (‘lesion block’), and two 5 mm blocks at either ends of the lesion block (rostral and caudal blocks).
3.
Tissue sectioning. Cut horizontal sections at 15 μm in thickness for the lesion block, transverse sections for both the rostral and caudal blocks of the spinal cord as well as the midbrains.
4.
Immunostaining. In LV/GFP injected group, the expression of GFP in the red nucleus and in RST axons could be viewed directly under a fluorescence microscope. As the GFP and DNROCK cDNAs are under the control of human synapsin promoter, for confirmation of the neuronal specific expression of the transgenes, sections of midbrain can be immunostained with a mouse anti-NeuN antibody (1:500; Chemicon, MAB377). In the DNROCK group, the animals are injected with a mixture of LV/DNROCK and LV/GFP for easy identification of the RST axons. For assessment of the co-expression of DNROCK and GFP in the red nucleus and spinal cord section, sections of midbrain and cervical spinal cord can be immunostained with a mouse anti-Flag M2 antibody (1:250, Sigma, F3165) as a 3 × FLAG tag is fused to the DNROCK construct (See Note (1) for viral vectors injected in our study and Note (2) for the use of an anterograde tracer).
5.
Quantification of regenerating axons. GFP-labeled axons in the white matters are counted in 6–7 horizontal sections at 45 μm intervals and in one transverse section 5 mm caudal to the lesion site. If the DNROCK expression is effective in promoting axonal growth, there should be more GFP-labeled RST axons growing longer distance toward the lesion border, crossing the lesion site, and even growing into the spinal cord caudal to the lesion. Also there may be more GFP-labeled axons sprouting to the grey matters. Therefore, to accurately assess the extent of axonal growth, the number of axons needs to be counted at different regions and at 3–5 distance points, e.g., 4–5 mm to the lesion center, rostral border of the lesion site (within 1.5 mm rostral to the lesion center), the lesion site, and caudal part (within 5 mm caudal to the lesion center) on horizontal sections, and at 5 mm caudal to the lesion center on transverse sections [9].
Note (1): Titers of LV/GFP in the LV/DNROCK and LV/GFP mixture is adjusted so that it has the same titer as that of LV/GFP only viral stock. We showed that the co-transduction efficiency of red nucleus neurons by LV/DNROCK and LV/GFP is 95.2 ± 0.5 % [9]. Therefore, GFP fluorescence in RST axons in the DNROCK and GFP co-injected group can be used as an indicator of DNROCK expression in the same axons.
Note (2): We injected biotinylated dextran amine (BDA) into the red nucleus to trace the RST axons in LV/DNROCK injected animals. However, we found that most LV/DNROCK transduced neurons were not labeled by BDA for some unknown reasons. Therefore, BDA may not be suitable as an anterograde tracer for LV transduced RST axons.
3.3 Gene Delivery to the Spinal Cord in a Dorsal Hemisection Model
For assessing the effects of treatments in promoting sensory axon regeneration, a commonly used model is the dorsal column transection, which cuts the ascending sensory axons that are responsible for transmitting fine touch, vibration and conscious proprioceptive information to the cerebral cortex. We used this model to assess the effect of LV mediated expression of NGF-sNgR1 fusion protein in the spinal cord to promote sensory axonal regeneration [44].
3.3.1 Surgical Procedure for Dorsal Hemisection
1.
Anesthetize female rats (200–250 g) as described above with Fluothane (ICI).
2.
Shave the operated areas around the eighth thoracic vertebra (about 4 cm × 3 cm) using veterinary hair clippers and clean the exposed skin with iodine and 70 % alcohol.
3.
Place the rat on a heated pad and cover with a sterile drape.
4.
Make an incision about 3 cm in the skin at the level of the eighth thoracic vertebra (T8) rostrocaudally. Incise the underlying musculature using a scalpel blade along both sides of the spine to expose the lamina of T8. Perform blunt dissection to split the deeper muscle layers.
5.
Perform a laminectomy of T8 using a Malleus Nipper (Fine Science Tools, #16140-11) under an operating microscope. Open the dura with a pair of microsurgical scissors.
6.
Crush the dorsal column of T8 spinal cord with a pair of watchmakers’ forceps at a depth of 1.5 mm to prevent heavy bleeding of the posterior spinal vein, and then cut the dorsal column using microsurgical scissors at the same depth. Place a small piece of fibrinogen flake from rat plasma (Sigma-Aldrich, #6755) on the surfaces of the spinal cord to cover the transection site and prevent further bleeding.
3.3.2 Injection of the Viral Vector into Spinal Cord
To minimize the injection damage caused by breathing the stereotactic frame used for injection is modified with a frame connected with two forceps to immobilize the spinal cord by holding the spines of the T7 and T9 (See Fig. 2).
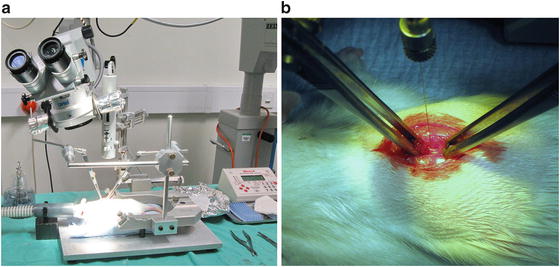
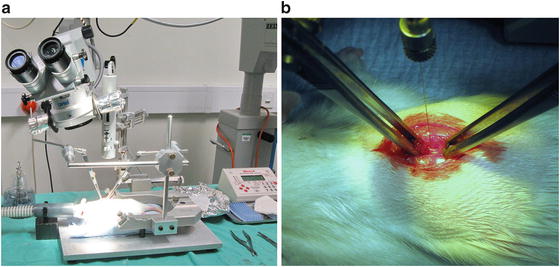
Fig. 2
Set-up for microinjection of viral vectors into rat thoracic spinal cord. (a) A photo showing the whole setup for microinjection of viral vector to thoracic spinal cord. Note how the two pairs of forces are fixed to the stereotaxic frame and hold the rat spine to reduce the movement of spine due to animal breathing. (b) A close-up photo showing how the forceps hold the rat spine
1.
After the spinal cord transection transfer the rat to the above device.
2.
Load 3–4 μl of viral vectors into a 10 μl Hamilton syringe.
3.
Stop the bleeding of injury before the viral vector injection.
4.
Under the operation microscope, using a 30 gauge needle to make a small puncture through the arachnoid mater and pia mater at the ipsilateral of rostral margin of the lesioned spinal cord. The insertion point should be away from blood vessels to avoid bleeding.
5.
Lower the injecting needle (33 gauge) through the puncture. When the needle reaches the surface of the spinal cord, take a reading on the stereotactic frame, and then slowly insert the needle into the spinal cord. When the needle reaches 1 mm into the spinal cord, withdraw the needle slightly and wait for approximately 2 min to allow the tissue to seal around the needle and help to prevent leakage (See Note (1) and (2) for more options of injection).
6.
Inject 1 μl of LV at a speed of 200 nl/min as described above.
7.
After the injection leave the needle in situ for 5 min and then gradually withdraw over 2–3 min to allow the diffusion of viral vector from the injection site and to prevent leakage from the needle track.
8.
Make a second injection at the caudal margin of the lesion site of the ipsilateral spinal cord as described above.
9.
Suture the muscles and skin with a 3-0 absorbable suture.
Note (1): A glass pipette needle can be pulled to a much finer tip than that of a 33 gauge stainless steel needle, therefore, glass needles cause less damage to the tissue. However, glass needles may clog or break easily, which prolongs the surgery time and causes wastage of viral vectors as the loaded needle has to be discarded and a new needle is loaded.
Note (2): Instead of inserting the needle vertically into the spinal cord, the needle may be inserted to the site of injection at an angle to the spinal cord. The advantage is that the longer needle track will reduce the leakage and increase the spread of the viral vector. The disadvantage is that it will cause more tissue damage.
3.3.3 Injection of Retrograde Tracers to the Sciatic Nerve
To study the regeneration of sensory axons after spinal cord injury cholera toxin β subunit (CTB) can be used to transganglionically label the ascending sensory axons. The animals are maintained for a designed period (normally for about 6–10 weeks after surgery). CTB can be injected 3 days before the termination of the experiment.
1.
Anesthetize the rat as before and place the rat in a prone position on a heated mat.
2.
Shave and sterilize the mid-thigh area of the ipsilateral leg.
3.
Make an incision of the skin and expose sciatic nerve.
4.
Load 5 μl 1 % CTB (List Biological Laboratories, Campbell, CA) into a 10 μl Hamilton syringe attached with 30-gauge needle (bevelled 30°).
5.
Make a small nick in the perineurium of the sciatic nerve using a pair of microscissors, through which the needle is inserted and pushed along sciatic nerve for a further 1 cm.
6.
Inject the tracer slowly into the sciatic nerve. At the end of the injection leave the needle in situ for 2–3 min and then gradually withdraw the needle.
7.
Close the skin incision with 3-0 absorbable sutures.
3.3.4 Immunohistochemistry and Quantification of Regenerating Axons
Perfuse the animals with 0.9 % saline and then 4 % paraformaldehyde 3 days after CTB injection. Remove segments of spinal cord containing the lesion site and brainstem for morphological examination. Cut sagittal cryostat sections (15 μm thick) of spinal cord containing the lesion site and transverse sections of brainstem. To view the glial scar around the lesion border, stain the sections with an antibody against glial fibrillary acidic protein (GFAP), an astrocyte marker. CTB-labeled sensory axons can be identified by immunostaining with a goat anti-CTB IgG antibody (1:4,000, List Biological Laboratories, Campbell, CA, USA. # 703).
Quantification of CTB-labeled axonal profiles within and rostral to the lesion cavity can be done by counting the number of CTB+ axonal profiles in several regions including various distance points to the caudal border of the lesion, at caudal border of lesion, in the lesion cavity, at the rostral border, and at various distance points from the rostral border of the lesion. CTB+ axonal profiles in 3–5 sections from each animal may be counted.
3.4 Transplantation of Genetically Modified Schwann Cells in a Corticospinal Tract Injury Model
As it has been described in Sect. 1, cell transplantation is considered as an important component in combinatorial treatments for spinal cord injury. Schwann cells (SCs) have been used for grafting into injured spinal cord in animal experiments for many years as they can be obtained easily and can myelinate CNS axons [75, 76], which is crucial for functional recovery. However, because they are the myelinating cells for peripheral nerves, Schwann cells do not mingle well with CNS glial cells and do not migrate into the host tissue. Since PSA is a highly hydrated sugar chain attached to NCAM, expression of PSA on the surface of Schwann cells will change their interaction with host CNS glial cells [63]. Furthermore, induced expression of PSA in and around the lesion site can further enhance the migration and integration of Schwann cells into host tissue [63]. Here we describe the combined transplantation of PST-transduced Schwann cells into the lesion with induced expression of PSA by direct injection of LV/PST around the lesion site after dorsal column transection of rat spinal cord, which severs the dorsal corticospinal tract (CST) axons deep in the dorsal column.
3.4.1 Culture and Transduction of Schwann Cells
1.
Isolate Schwann cells from sciatic nerves and brachial plexus of Wistar rats of postnatal day 2 as described previously [77].
2.
Culture the cells in DMEM containing 10 % fetal bovine serum, 2 μM forskolin (Sigma-Aldrich, #F6886), 25 ng/ml fibroblast growth factor basic (PeproTech, #450-33), and 5 μg/ml insulin (Sigma-Aldrich, # I6334) (See Note (1) on how to evaluate the purity of Schwann cells).
3.
When the Schwann cells are 60–70 % confluent, dissociate one dish of cells and count the number of cells.
4.
Add LV/GFP or LV/PST viral stocks into the culture at a multiplicity of infection (MOI) of 50 and infect the cells overnight (See Note (2) for visualization of PST transduced cells).
5.
Replace the medium the following day and split the cells when they reach 90 % confluent.
6.
Observe the cells under an inverted fluorescence microscope for the expression of GFP. GFP fluorescence may be visible after 2–3 days and reach a relatively high level after 5–7 days (See Note (3) on how to increase the transduction efficiency).
7.
After being passaged three times, the transduced Schwann cells can be dissociated for transplantation. If they are not used immediately, the cells can be aliquoted and stored in liquid nitrogen for future use.
Note (1): The purity of primary Schwann cell cultures can be evaluated by immunostaining for Schwann cell markers p75 neurotrophin receptor (p75NTR) and S100. Highly purified cultures should have >95 % cells stained positive for p75NTR and S100. The main contaminating cells are fibroblasts.
Note (2): In our construct, GFP is fused to the C-terminal of PST. In primary cells, the PST-GFP fusion proteins appear as tiny green dots on Golgi apparatuses. However, it is very difficult to identify the PST transduced Schwann cells grafted into spinal cord. For easy identification, Schwann cells can be co-transduced with LV/PST and LV/GFP. The efficiency of co-transduction can be assessed by staining the Schwann cells with a mouse monoclonal anti-PSA antibody (IgM, 5A5, 1:200, Hybridoma Bank, Iowa City, IA). Efficiency of co-transduction can reach 93–97 %.
Note (3): If the transduction efficiency is below 90 %, more viral vectors can be added to the culture while expanding the cells.
3.4.2 Dorsal Hemisection, Transplantation of Schwann Cells, and Injection of Viral Vectors
1.
Dissociate Schwann cells from culture dish and wash the cells with DMEM twice to remove serum from the cells (See Note (1) for the use of cells stored in liquid nitrogen).
2.
Count the cells and resuspend the cells in DMEM to a suitable density (250,000 cells/μl in this study). Keep the cells on ice.
4.
Inject 1 μl viral vectors at each of the four points: at 1 mm left and right of midline to the rostral and caudal margins of lesion site as described in Sect. 3.3.1.
5.
Load 6 μl (1.5 × 106) Schwann cells into a 25 μl Hamilton syringe attached with a 33 gauge needle and inject the cells into the lesion site at 400 nl/min. Leave the needle in situ for 5 min.
6.
Place a small piece of fibronectin mat [78] to cover the surface of transplants and the adjacent spinal cord to seal the lesion site, stop bleeding, and prevent the leakage of grafted cells.
7.
Suture the muscle and skin in separate layers with 3/0 absorbable sutures.
8.
Administer normal saline equivalent to 2 % of body weight intraperitoneally immediately after surgery and then subcutaneously for the first 3 days after surgery to prevent dehydration.
9.
To prevent immune rejection of the transplanted cells, treat the animals with cyclosporine (10 μg/g body weight) subcutaneously before surgery and then daily for the first 2 weeks after surgery.
Note (1): May need to administer by subcutaneous injection of buprenorphine immediately following anesthesia and 24 h post-surgery for pain relief.
Note (2): Pipette the resuspended cells several times before loading into the Hamilton syringe to assure that the density of cells remains the same for each loading. Avoid air bubbles when pipetting the cells.
3.4.3 Behavioral Assessment for Motor Function
Locomotor impairments of the rats after spinal cord injury were assessed using a 120 cm long horizontal ladder walking test. Metz and Whichaw has described a similar test in detail [79]. Animals are trained for 2–3 weeks before surgery. Perform the first test 3 days after surgery and then weekly for 9 weeks. Place the video camera below the level of the ladder and adjust the shooting angle to capture the rat’s feet that slip through the rungs of the ladder. The total numbers of hind-limb steps made and the numbers of slips are recorded.
3.4.4 Injection of Anterograde Tracer
To trace the regenerating CST axons after the treatment, a commonly used neuronal tracer is BDA (MW 10,000, Life Technologies, Cat. No: D-1956). We injected the BDA into the motor cortex bilaterally at 6 points of each brain hemisphere 3 weeks before the sacrifice of the animals.
1.
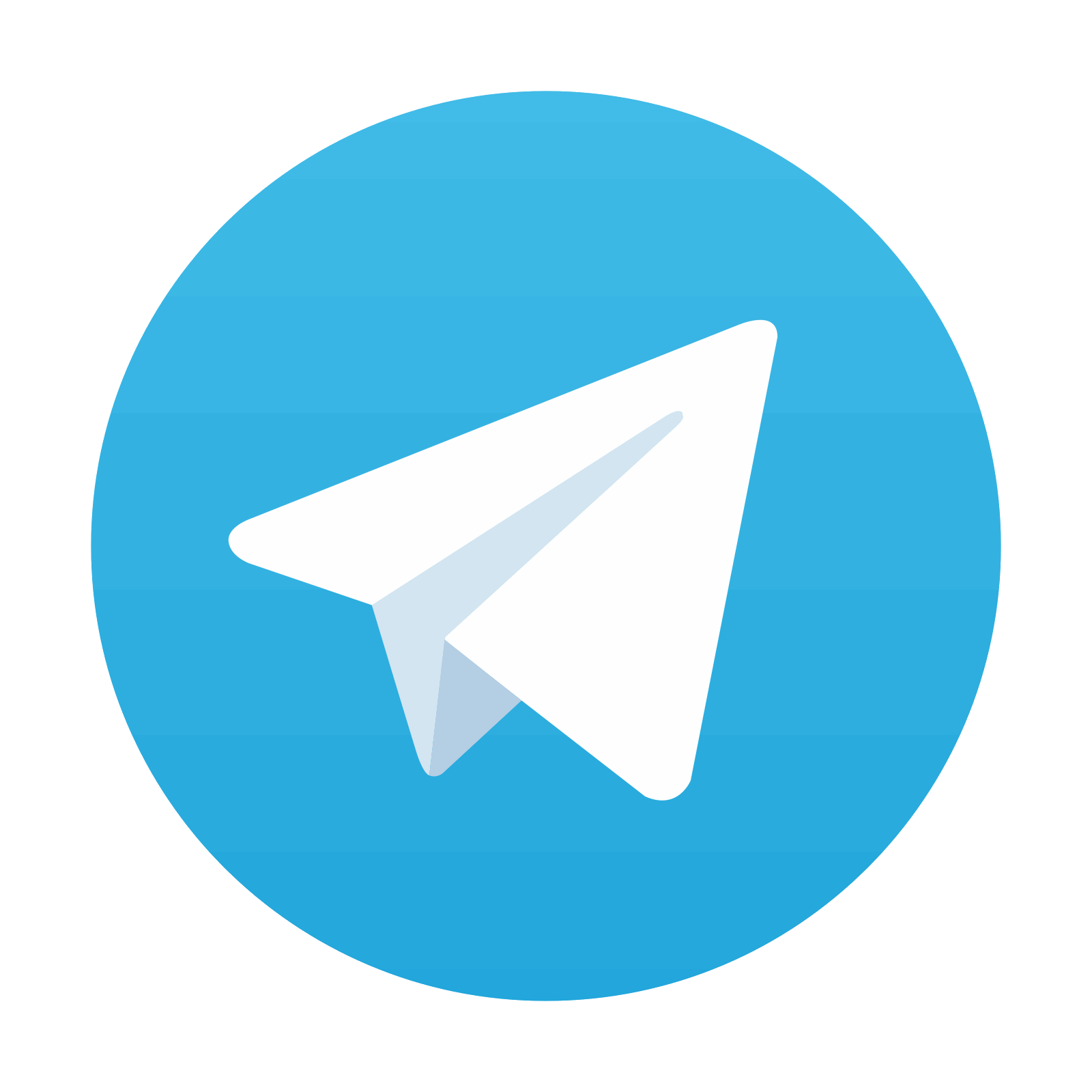
Anesthetize the rat as described above and place the rat in a stereotactic frame.
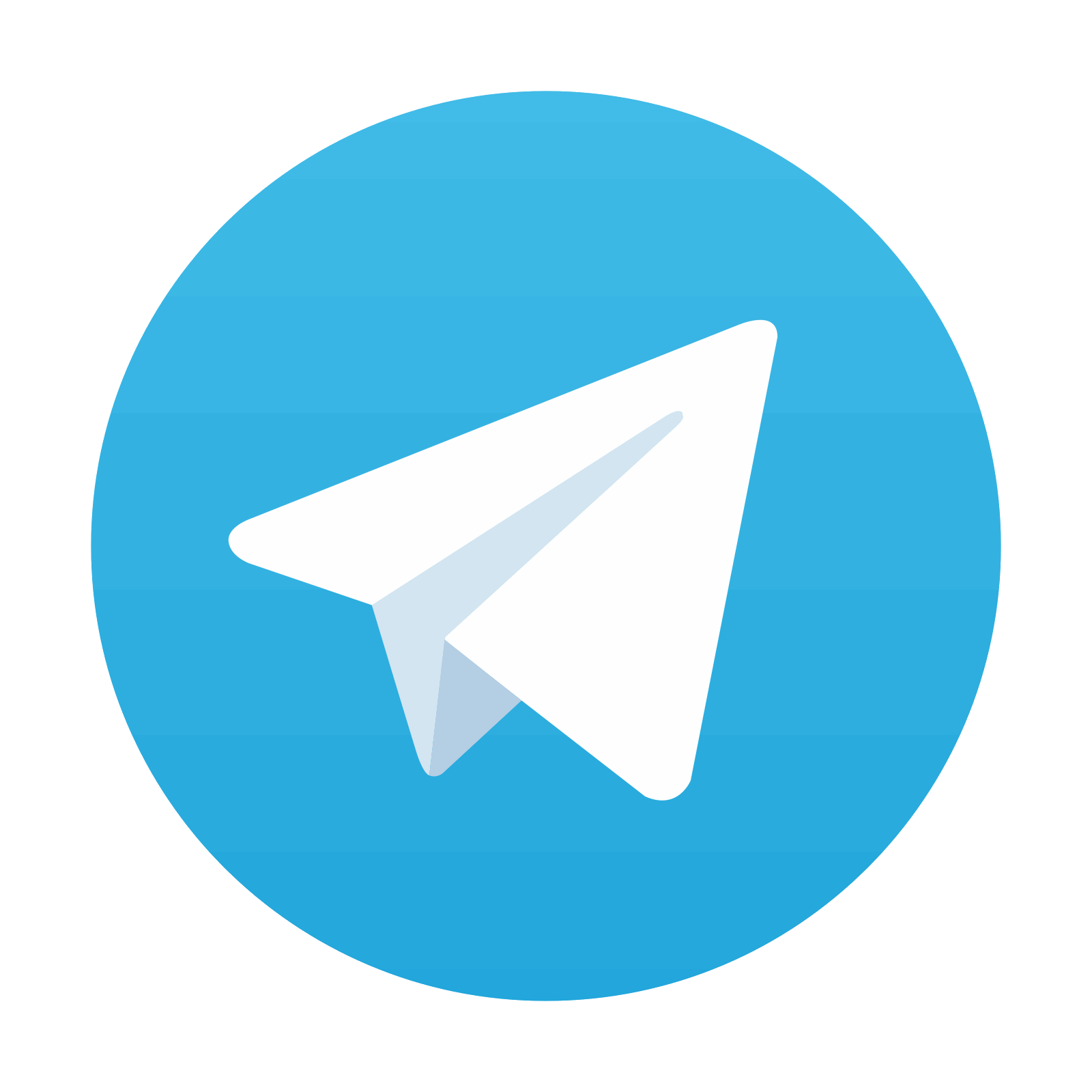
Stay updated, free articles. Join our Telegram channel

Full access? Get Clinical Tree
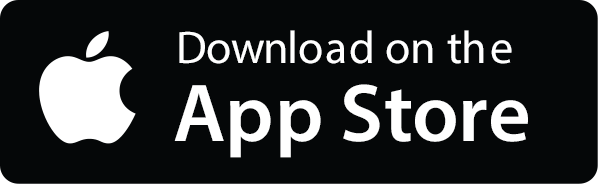
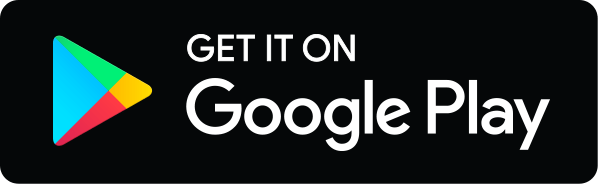
