Author (reference)
Animal
System
Age/injection site
Proliferation (RTK/KRAS/PI3K)
Apoptosis (p53/ARF)
Cell cycle (Rb/INK4)
Best combined penetrance
Holland et al. 2000 [13]
Mice
RCAS
Neonatal/R frontal
AKT, KRAS
26 %
Dai et al. 2001 [9]
Mice
RCAS
Neonatal/R frontal
PDGF
INK4a
70 %
Uhrbom et al. 2002 [16]
Mice
RCAS
n/a
KRAS
ARF
INK4a
42 %
Dai et al. 2005 [10]
Mice
RCAS
n/a
PDGF, KRAS, AKT
n/a
Hambardzumyan et al. 2009 [12]
Mice
RCAS
4–6.5 weeks
PDGF
p53, ARF
INK4a
100 %
Assanah et al. 2006 [8]
Rats
Retrovirus
Adults/corpus callosum
PDGF
100 %
Lei et al. 2011 [14]
Mice
Retrovirus
Adults/white matter
PDGF, PTEN
p53
100 %
Wiesner et al. 2009 [17]
Mice
Sleeping Beauty
neonatal/R lateral ventricle
NRAS, SV40-LgT, AKT
p53
100 %
Marumoto et al. 2009 [15]
Mice
Lentivirus
8–16 weeks/hippocampus
HRAS, AKT
p53
100 %
de Vries et al. 2010 [11]
Mice
Lentivirus
8–16 weeks/cortex
KRAS
p53
INK4a
100 %
Lynes et al. 2013 [29]
Rats
Lentivirus
7–10 weeks/cortex
PDGF, HRAS, AKT
100 %
There are multiple ways to create somatic mutations in rodents. Initial models were built on RCAS (replication-competent avian sarcoma-leukosis) vectors, which required mice with germline TVA (tumor virus A) retroviral receptors linked to cell-type-specific promoters [9, 10, 12, 13, 16]. Canoll et al. used a construct with replication-deficient retrovirus plasmids and vesicular stomatitis virus G (VSV-G) coats to somatically introduce oncogenes into rodents [8, 14]. The Sleeping Beauty [6] model employs the SB transposase enzyme to transfer genetic material from plasmids into host chromosomes after injection into neonatal mice lateral cerebral ventricles. Using various combinations of human oncogenes and inhibitors of tumor suppressor function, they were able to reliably induce spontaneous tumors resembling human GBM [17]. Finally, lentivirus vectors have been used with increasing frequency to express oncogenes (HRAS, KRAS, PDGF, AKT) and to knock down tumor suppressors (p53 and INK4) [11, 15, 18]. The lentivirus vector system is ideal due to the ability of LV vectors to transduce both dividing and nondividing cells and encode large (up to 9.7 kb) DNA sequences [19–21].
Models utilizing lentivirus to induce GBM often take advantage of the Cre-Lox system [11, 15]. One recent report used a lentiviral vector encoding floxed HRAS and AKT oncogenes in GFAP-Cre transgenic mice (with or without p53 heterozygosity) [15]. The combination of these oncogenes was sufficient to cause high-grade gliomas when injected into neurogenic areas (hippocampus and subventricular zones), and the loss of p53 increased their incidence and decreased their latency. In another report, a GFAP-Cre lentivirus was injected into transgenic mice carrying various combinations of floxed genes [11]. In this model, activated KRAS and loss of INK4a/ARF were sufficient to cause high-grade gliomas, and the additional loss of p53 caused higher-grade tumors to form more rapidly. The latter model was also used as a screen for therapeutic interventions, in which temozolomide was tested and shown to be effective [11].
Lentivirus-mediated tumor induction in rats is more effective than in mice, often not requiring mutations in tumor suppressor pathways. Intracranial injection of a retrovirus expressing PDGF-β has induced GBM with complete penetrance and short latency in rats [8]. In recent experiments, injection of lentiviral vectors expressing PDGF-β and HRAS-V12 respectively induced GBM in Sprague-Dawley rats with complete penetrance and latency ranging from 27 to 60 days [18]. The resulting glioblastomas demonstrated the nuclear atypia, mitoses, endothelial proliferation, and necrosis that constitute the pathologic hallmarks of the human disease (Fig. 1). Injection of lentiviral vectors expressing HRAS-V12 alone produced slower growing gliomas with high penetrance but did not cause animal morbidity until 6-month post-virus delivery. In addition, this model allowed us to test a gene therapy strategy for glioblastoma, i.e., adenovirus vectors encoding herpes simplex type 1 thymidine kinase (b), which induces tumor cell death when used in combination with ganciclovir (GCV), thus adding relevant knowledge related to a therapeutic modality recently tested in a Phase 3 clinical trial for GBM in Europe [22–24]. These models demonstrate the versatility and ease of modifying the genetic profile in situ with observable, meaningful results.
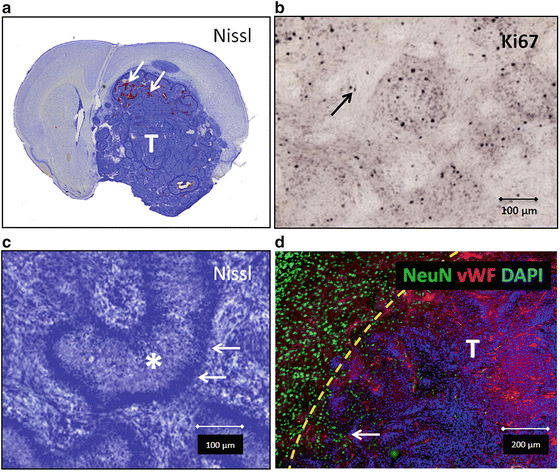
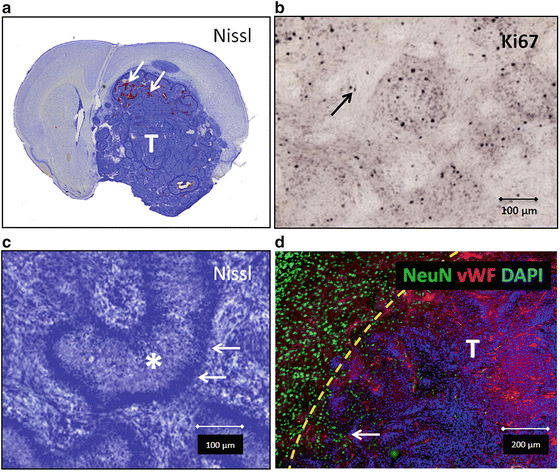
Fig. 1
Representative histology from tumors induced using lentiviral vectors expressing oncogenes PDGF and HRAS-V12. (a) Nissl staining of large glioblastoma (indicated by “T”). Areas of tumor hemorrhage indicated (arrows). (b) Ki67 staining (arrow) showing moderate to high proliferation index. (c) Nissl staining showing pseudopalisades (arrows) surrounding necrosis (asterisk), a hallmark of human GBM. (d) Immunostaining of neuronal tissue (NeuN, green) being infiltrated by tumor with vasculature immunoreactive with Von Willebrand factor (VWF, red). Yellow dotted line delineates tumor border as evident by increased cellular density with DAPI staining. This infiltrative high-grade tumor entraps healthy neurons (arrow) (color figure online)
The protocols below will address (Sect. 3.2, step 1) lentiviral vector production, (Sect. 3.2, step 2) intracranial lentiviral injection in rats, and (Sect. 3.2, step 3) testing of a conditional cytotoxic gene therapy, i.e., herpes simplex virus type I thymidine kinase (HSV1-TK) encoded within a first-generation adenovirus vector (Ad-TK) in combination with systemic delivery of ganciclovir (GCV), in the LV-induced endogenous GBM model.
2 Materials
1.
Lentiviral vectors of interest, i.e., pLVX-Puro-hPDGFb, pLVX-Puro-Flag-HRAS-V12, and pLVX-Puro-HA-AKT.
2.
Therapeutic and control adenoviral vector, i.e., Ad-TK and Ad-0.
3.
Adult rat (250 g body weight, Fisher or Sprague-Dawley Strain, Charles River Inc., http://www.criver.com).
4.
Drugs:
5.
Betadine (Bruce Medical, cat# FR-2100-88)
6.
Virkon S (DuPont, www.dupont.com)
8.
Warm lactated Ringer’s solution (Hospira Inc., cat# 0409-7953-30)
9.
Stereotactic frame with rat adapters and blunt ear bars (Stoelting Inc.)
10.
Stereomicroscope (e.g., Zeiss Stemi 1000 zoom) equipped with 16× eyepieces and 0.4× auxiliary objective lens and mounted on hinged coupling arm on a heavy foot stand (or equivalent)
11.
Electric drill with 1.75-mm and 0.6-mm drill bit (Stoelting Inc., cat# 58610).
12.
Digital scale for animal weighing (Harvard Apparatus, cat# 724586)
13.
Fiber-optic illuminator with twin gooseneck pipes (Leica Inc.)
14.
10 μl, 26-G Hamilton syringe with needle (Fisher, cat# 701RN)
15.
5–10 μl, 33-G Hamilton syringe with needle (Fisher, cat# 75RN)
16.
3–0 nylon sutures (Ethicon Inc., cat# 1663H)
17.
Petri dish, plastic (BD Pharmingen, 353002)
18.
Surgical equipment:
(a)
Surgical shavers (Stoelting Inc., cat# 58610)
(b)
Scalpel and blades (Cardinal Health, cat# D2862-15)
(c)
Skin retractors (Fine Science Tools Inc., mouse cat# 17000-03, rat cat# 17000-04)
(d)
Cotton swabs (VWR, cat# 89031-270)
(e)
Curved and straight forceps
(f)
Holding scissors
(g)
Sharp scissors
(h)
Sterile gauze (VWR, cat# 95038-720)
(i)
Bead sterilizer (VWR, cat# IS-350)
(j)
Red lamp (Sylvania, cat# 14663)
3 Methods
3.1 Lentiviral Vector Production
2.
Prepare transfection materials. Approximately 24 h before transfection, seed 4–5 × 106 Lenti-X 293T cells/100 mm plate, in 10 ml of growth medium. Make sure that the cells are plated evenly. Incubate at 37 °C, 5 % CO2 overnight. Continue to incubate the cells until you are ready to add the transfection mixture in step 8. The cells should be 80–90 % confluent at the time of transfection.
3.
Thoroughly vortex Xfect polymer (Lenti-X Packaging System from Clontech, cat# 631247).
4.
For each transfection sample, prepare two microcentrifuge tubes by adding reagents in the following order:
Get Clinical Tree app for offline access
(a)
Tube 1 (plasmid DNA)
557 μl Xfect reaction buffer
36 μl Lenti-X HTX packaging mix
7 μl Lenti-X vector DNA (1 μg/μl)
600 μl total volume
(b)
Tube 2 (polymer)
592.5 μl Xfect reaction bufferStay updated, free articles. Join our Telegram channel
Full access? Get Clinical Tree
