Fig. 8.1
An 83-year-old man without any vascular risk factors developed dysarthria and confusion. DWI showed multiple scattered infarcts in bilateral anterior and posterior circulations. MR angiography, electrocardiography and echocardiography findings were normal. His serum d-dimer level was elevated, and a further workup revealed that he had lung adenocarcinoma. Cancer associated hypercoagulopathy was considered as a stroke mechanism
In patients with significant internal carotid artery (ICA) steno-occlusive disease, DWI lesions occurring in hemodynamic risk zones, along with large perfusion defect suggests the presence of hemodynamic impairment [14] (Fig. 8.2) while ipsilateral territorial infarcts suggest embolic mechanism (Fig. 8.3). In patients with intracranial atherosclerosis (ICAS), ipsilateral territorial, cortical infarcts are also associated with embolic mechanisms (Fig. 8.4), whereas deep perforator infarcts in the territory of perforating arteries suggest branch occlusion as a responsible stroke mechanism (Fig. 8.5) [15, 16].





Fig. 8.2
An 85-year-old hypertensive man developed dysarthria, aphasia, and mild right hemiparesis. Initial DWI showed dot like infarcts in the middle cerebral artery–posterior cerebral artery borderzone and internal borderzone areas (left upper and middle images). Perfusion weighted MRI (right upper image) using regional mean transit time showed hypoperfused areas in the left MCA territory. The symptoms fluctuated and then his limb weakness worsened (MRC grade III) four days later when DWI showed enlarged ischemic lesions (left lower image). MR angiography showed severe atherosclerotic stenosis in the left carotid bulb area (right lower image, arrow). Angioplasty and stenting procedures were performed, and the patient’s symptoms gradually improved

Fig. 8.3
A 76-year-old hypertensive, cigarette smoker developed sudden sensory aphasia and right hemiparesis. DWI showed an infarct in the left parietal area. MR angiography showed focal stenosis with ulceration in the left carotid artery (middle image, arrow). Conventional angiography showed similar findings (right image, arrow). The stroke mechanism was considered an artery-to-artery embolism from the ulcerative plaque

Fig. 8.4
A 62-year-old cigarette smoker developed dysarthria, acalculia, mild left hemiparesis, and paresthesia. DWI showed scattered infarcts in the right frontal, temporal and parietal area. MR angiography showed focal severe stenosis in the M1 portion of the left middle cerebral artery (right lower image, arrow). Mild stenosis was also observed in the right distal M1 portion. Five days later, repeat MR angiography showed that the middle cerebral artery stenosis was unchanged (not shown). The stroke mechanism was considered an artery-to-artery embolism from the left M1 atherosclerotic lesion

Fig. 8.5
A 52-year-old hypertensive and diabetic woman presented with dysarthria and left hemiparesis. DWI showed an infarct involving the right putamen/internal capsule and corona radiata. MR angiography showed right middle cerebral artery stenosis (left lower image, thin arrow) that probably induced branch (perforator) occlusion. High resolution vessel-wall MRI showed an eccentric, enhancing lesion, consistent with atherosclerotic plaque (right lower image, thick arrow)
Because these DWI lesion patterns provide only partial clues, other information such as medical history, findings on neurologic examinations, and vascular and perfusion imaging results should be concomitantly considered to determine stroke mechanisms in individual patients.
Gradient Echo MRI
Gradient echo MRI (GRE) can identify microbleeds as small dark signals, due to the paramagnetic properties of deoxyhemoglobin. The presence of multiple microbleeds indicates that these patients have fragile, hemorrhage prone small vessel disease [17], associated either with advanced hypertension or amyloid angiopathy [18]. In addition, GRE provides information on the composition of thrombi during the acute stage of stroke, by revealing the so-called “GRE susceptibility vessel sign” (GRE SVS) [19–21]. Because red thrombi formed in low pressure areas such as cardiac chambers and are rich in fibrin and trapped red blood cells [22, 23], GRE SVS is more frequently observed in patients with cardioembolic stroke than in those with other stroke subtypes [24].
Perfusion Weighted MRI
Perfusion weighted MRI (PWI) is a set of techniques that creates images depicting hemodynamics at the microvascular level. PWI has an advantage over other tools, such as PET or SPECT, in that it can easily be combined with structural and vascular MRI in a single session. Perfusion parameters are most commonly obtained following the intravenous bolus injection of a paramagnetic contrast agent, with perfusion parameters changing as the contrast agent passes through the brain vasculature. This technique generates time-contrast concentration curves, which allow the computation of regional mean transit time (rMTT), time-to-peak (rTTP), cerebral blood volume (rCBV), and cerebral blood flow (rCBF). The most sensitive MRI measure of abnormal perfusion is the rTTP map, which includes benign oligemic tissue as well as the penumbral area. Thus, the abnormal areas detected by rTTP and rMTT images are usually larger than those shown in rCBF and rCBV images.
PWI-DWI mismatches are used to assess potentially salvageable areas in patients with acute stroke and can help in deciding whether to perform recanalization therapy. These mismatches are also useful in identifying hypoperfusion mechanisms in patients with strokes and TIAs (Fig. 8.2, right upper image).
High Resolution Vessel Wall MRI
High resolution cross sectional vessel wall MRI (HRMRI) is occasionally used to assess the diseased vessel wall. Unlike other MR images, axial images are acquired based on a localizing two-dimensional time-of-flight MRA technique, focusing on the stenotic segment. HRMRI has emerged as a noninvasive method for identifying the characteristics of carotid plaques, including the state of the fibrous cap, the presence of necrotic lipid cores, and intraplaque hemorrhages [25, 26]. In patients with intracranial artery stenosis, HRMRI can detect atherosclerotic plaques (Fig. 8.5, right lower image), and help in determining the other etiologies of stenosis (e.g., dissection or moyamoya disease) [27], but is still largely a research tool.
Doppler Ultrasonography
Duplex ultrasonography is a noninvasive method that can detect the stenosis of atherosclerotic vessels and identify the nature of plaques. Doppler findings of ulcerated, heterogeneous plaques suggest that these plaques are associated with a high risk for embolic stroke. Duplex scans in combination with MRA are frequently used to select patients for carotid endarterectomy.
Transcranial Doppler (TCD) imaging is helpful in assessing ICAS, by showing altered blood flow velocity and wave patterns in stenosed vessels. Despite its higher negative predictive value (83 %) TCD has a lower positive predictive value (55 %) than MRA [28]. Therefore, it cannot detect mild stenosis without producing significant hemodynamic alteration. Nevertheless, it can noninvasively identify and monitor the stenosed intracranial artery, and assess the status of collateralization. In addition, microembolic signals (MES) detected by TCD are likely to represent an embolus passing through the insonated artery [29–32] and help in assessing the risk of embolism [33]. Unfortunately, TCD results are dependent on the skills of individual technicians, and Doppler signals cannot be obtained in some elderly women due to temporal window failure [34].
Mechanisms of TIA and Stroke
Large Artery Disease
Large artery disease (LAD) is a major cause of cerebral infarction and TIA. Its main pathology consists of thrombosis superimposed on atherosclerosis. The process of atherosclerosis is complex. Repeated mechanical or toxic injuries to the intima associated with turbulent flow, and vascular risk factors such as hypertension, diabetes, hypercholesterolemia and cigarette smoking, promote the process of atherosclerosis. Circulating lipids, especially low density lipoproteins, enter the vascular wall and inflammatory processes are initiated. Cholesterol containing macrophages and smooth muscle cells proliferate and result in atheroma formation [35–37].
Atherosclerosis is prone to occur in bifurcation areas, where blood turbulence occurs, which include the carotid bulb, siphon, proximal middle cerebral artery (MCA), proximal vertebral artery, proximal/mid-basilar artery, and proximal posterior cerebral artery (PCA). Although extracranial atherosclerosis (ECAS), especially ICA bulb disease, is the most common form of LAD in Caucasians, ICAS is more frequent than ECAS in Asians, Hispanics, and Blacks [38]. The reason for this ethnic difference is not fully understood [39].
Detailed stroke mechanisms in LAD include artery-to-artery embolism, hypoperfusion, branch occlusion, in situ thrombotic occlusion, and their combinations.
Artery-to-Artery Embolism
Examination of carotid endarterectomy specimens revealed that plaque inflammation, ulceration, erosion and hemorrhages were more common in symptomatic than asymptomatic patients [40–42]. Rupture of this unstable plaque promotes thrombus formation [43, 44], that may be broken up by blood flow and migrate through the bloodstream to occlude distant arteries, resulting in clinical symptoms (artery-to-artery embolism). The plaques can be identified in vivo by CTA, MRA, or conventional angiography (Fig. 8.3). On duplex scans, vulnerable plaques appear as echolucent and heterogeneous, with occasional evidence of intraplaque hemorrhage [45]. MES detected by TCD is also helpful in predicting future embolic stroke [46]. Artery-to-artery embolism is the predominant stroke mechanism in patients with ECAS, and one of the important stroke mechanisms in patients with ICAS (i.e., proximal MCA to distal MCA) [47] (Fig. 8.4).
DWI is useful in assessing the embolic mechanism of stroke, in that it can reliably detect small, scattered, cortical infarcts in the territory of the diseased artery. In many patients, DWI-identified lesions are also located in border zone areas, suggesting that embolism often develops in combination with hypoperfusion. Underlying perfusion deficits may contribute to artery-to-artery embolism by promoting a thrombotic condition and by the impaired clearance of migrated emboli [48].
Although less frequent than in anterior circulation stroke, artery-to-artery embolism also occurs in the posterior fossa. Significant atherothrombosis in the proximal vertebral artery often induces embolization, occluding distal arteries such as the PCA, superior cerebellar artery, posterior inferior cerebellar artery and the tip of the basilar artery [49]. Stenoses in the distal vertebral and basilar arteries may also produce embolism, although they more often cause brainstem infarction by way of branch occlusion [50, 51]. As in the anterior circulation, embolisms seem to occur more frequently in the setting of posterior fossa hypoperfusion caused by bilateral vertebral artery disease or hypoplasia.
Embolisms may develop in more proximal arteries such as the common carotid artery, subclavian arteries, ascending aorta and aortic arch. Transesophageal echocardiography is required to detect plaques in the aorta. Case control studies showed that thick (≥4 mm), mobile atherosclerotic plaques in the aorta are prone to develop recurrent strokes [52], although this argument has not been unanimously agreed [53, 54].
In Situ Thrombotic Occlusion
Extensive thrombus formation in areas of atherosclerotic plaque can ultimately occlude the vessel. In patients with ECAS, the clinical consequences of arterial occlusion are not so grave because of the ample collateral circulations in the circle of Willis. Therefore, total arterial occlusion may remain asymptomatic or produce minor hemodynamic TIAs or strokes. In rare patients with insufficient collaterals or those with previous contralateral ICA occlusion, ICA occlusion may result in devastating infarcts involving whole ICA (MCA and ACA, plus PCA in the presence of fetal circulation) territories [55].
In contrast to ECAS, insitu thrombotic occlusion in patients with ICAS more often produces significant cerebral infarction as the collateral circulation is less sufficient. The resultant infarcts are usually larger than those caused by branch occlusion. However, unlike cardiogenic embolism, in situ thrombotic occlusion rarely produces sudden, whole territory infarction because of the relatively well developed collateral circulation in patients with a chronic atherosclerotic process [56]. In patients with in situ thrombotic occlusion, the initial infarct size frequently grows in following hours or days, accompanied by progressive neurological worsening (Fig. 8.6). Thus, the ultimate size of the infarct varies according to the status of the collateral circulation, the speed of arterial occlusion and hemodynamic stability after the occurrence of stroke. With sufficient collaterals, total thrombotic intracranial occlusion may remain asymptomatic or result in only minor stroke or TIA.


Fig. 8.6
A 64-year-old hypertensive man developed mild right hemiparesis and sensory aphasia. (a) DWI at the time of admission showed acute infarcts in the left lenticulocapsular and borderzone areas between middle cerebral artery and posterior cerebral artery. (b) MR angiography showed thrombotic occlusion of the left middle cerebral artery. The patient’s neurologic symptoms progressively worsened to have severe right hemiparesis and global aphasia. (c) Follow up MRI 4 days later showed increased lesion size (from Wong KS CL, Kim JS. Stroke mechanisms In Kim JS, Caplan LR, Wong KS (ed) Intracranial Atherosclerosis, John Wiley & Sons, 2008:57–68, with permission)
Branch Occlusion
Branch or perforator occlusion is a stroke mechanism unique to patients with ICAS. Atherosclerotic plaques in the intracranial artery can occlude the orifice of the perforators, causing infarcts limited to the subcortical (Fig. 8.5) or brainstem areas [57] (Fig. 8.7). Pathological studies [58, 59] have shown that the pathologic substrates occluding the branching vessel are microdissection, plaque hemorrhage, and platelet-fibrin materials. Branch occlusion is an important, yet so-far neglected mechanism of LAD [57, 60]. It is one of the major mechanisms of brainstem stroke [50, 51, 61, 62]. Branch occlusion is currently easily recognized by imaging methodologies such as MRA and CTA [63–66]. Compared with arterial lesions producing embolism or hemodynamic impairment, branch occlusion is related to milder atherosclerosis [67].


Fig. 8.7
An 81-year-old woman with hypertension and dyslipidemia developed dysarthria, right facial palsy, hemiparesis, and hemiataxia. DWI showed an infarct involving the left paramedian area of the pons. MR angiography showed focal basilar artery stenosis (lower image, arrow) that probably resulted in branch (perforator) occlusion
The infarcts associated with branch occlusion do not differ fundamentally from “lacunar infarcts” caused by lipohyalinotic small artery disease (SAD), although their pathologic nature is different. The subcortical infarcts caused by branch occlusion tend to extend to the basal surface (Figs. 8.5 and 8.7), whereas a lacune caused by lipohyalinosis usually produces an island of ischemic tissues within the parenchyma (Fig. 8.8). Although both conditions equally produce lacunar syndromes clinically, the former is more often associated with atherosclerosis in other vascular beds [68], and an unstable and adverse clinical course than the latter [51, 69, 70].


Fig. 8.8
A 54-year-old hypertensive man suddenly developed dysarthria, left hemiparesis, and sensory symptoms limited to the perioral area and the left hand. DWI showed an infarct involving the right putamen and internal capsule. MR angiography findings were normal. The stroke mechanism was considered a perforator occlusion associated with small vessel (lenticulostriate artery) disease
Hypoperfusion
Progressive narrowing of the atherosclerotic vessel leads to hypoperfusion distal to the site of stenosis. Generally, the degree of hypoperfusion depends on the severity of vascular stenosis/occlusion, and there is a correlation between the occurrence of ischemic stroke and the severity of occlusive disease [71, 72]. However, the ischemic event is also influenced by the status of collateral flow, from arteries at the circle of Willis, external carotid artery system and cervicothyroid arteries, etc.
In patients with severe vascular stenosis/occlusion and insufficient collaterals, hemodynamic TIAs can occur. Typically, symptoms such as hemiparesis, aphasia, monocular blindness, limb shaking (in anterior circulation disease) or dizziness, diplopia, and visual disturbances (in posterior circulation disease) occur briefly and stereotypically in patients who are dehydrated or fatigued or at the time they suddenly stand up. Revascularization therapies, such as angioplasty/stenting or bypass surgery, may rapidly relieve these symptoms (Fig. 8.2). When stroke develops, the symptoms may fluctuate widely according to the degree of hydration, blood pressure and the position of the patient’s head. With a continued perfusion defect, the symptoms may worsen gradually.
In the anterior circulation, infarcts caused by hemodynamic impairment usually develop in superficial (anterior cerebral artery-MCA, MCA-PCA) and/or internal borderzone areas (areas between superficial MCA pial penetrators and lenticulostriate arteries), the latter being more specifically associated with hemodynamic impairment than the former. Hypoperfusion as a pathogenic mechanism can therefore be recognized by DWI lesion patterns, the degree of vascular stenosis, the status of collateral vessels, perfusion imaging findings and appropriate clinical histories. In the posterior circulation, hemodynamic TIAs and strokes occur following severe steno-occlusive lesions occurring in both vertebral arteries or the basilar artery. Here, DWI patterns of hemodynamic infarction have not been clearly established.
Although hypoperfusion is an important stroke mechanism, strokes caused by hemodynamic failure alone are uncommon in clinical practice. More often, hypoperfusion plays an additive role in the development of stroke, together with other major stroke mechanisms. As discussed earlier, the co-occurrence of hypoperfusion and embolism in patients with severe steno-occlusive vascular diseases is common, due in part to both mechanisms being related to complicated atherosclerotic plaques protruding into the lumen, and in part to ineffective wash out of emboli in hypoperfused areas [48].
Small Artery Disease (SAD)
A single subcortical infarction, traditionally called a “lacunar infarction” usually results from SAD [73]. Its pathological hallmarks include irregular cavities, less than 15–20 mm in size, located deep in the cerebral hemisphere, brainstem, and the cerebellum. Penetrating arteries associated with these lesions have disorganized vessel walls, fibrinoid material deposition and hemorrhagic extravasation through arterial walls, called “lipohyalinosis” by Fisher [59, 73–79] (Fig. 8.8). More benign and common vascular changes include the deposition of collagen replacing smooth muscles cells, with preserved lumen size and overall vascular architecture. Although these simple vascular changes do not produce arterial occlusions, they are associated with reduced vascular distensibility and are probably related to white matter ischemic changes commonly seen in elderly patients and those with vascular risk factors [80].
These vascular changes occur at arteries or arterioles 40–400 μm in diameter, and frequently affect the lenticulostriate branches of the MCA, the thalamoperforating arteries from the PCA and the perforators of the basilar arteries. The resultant subcortical infarcts produce “lacunar syndromes,” including pure motor stroke, pure sensory stroke, sensorimotor, dysarthria clumsy hand, and ataxic hemiparesis without cortical symptoms. However, lacunar infarctions are not always caused by non-atherosclerotic lesions. As discussed earlier, single subcortical infarctions may be caused by branch occlusion associated with atherothrombotic lesion in the parental, intracranial artery (Figs. 8.5 and 8.7) These atherosclerotic causes of subcortical infarction have been collectively described as “branch atheromatous disease (BAD)” by Caplan [57]. In addition, subcortical lacunar infarcts, especially large ones, may be caused by embolism from a diseased heart or carotid artery [81, 82].
Cardiac Embolism
Embolism from a diseased heart is the cause of approximately 20–25 % of ischemic strokes [83–85], with the proportion depending on the extensiveness of cardiac workup, such as Holter monitoring or transthoracic/transesophageal echocardiography. A thrombus arising in the heart most frequently travels to the MCA territory. DWI studies have shown that the most frequent pattern of cardiogenic embolic infarctions was territorial and cortical [67]. However, cardiac embolism may affect any part of the brain, including the subcortical and brainstem areas [81, 82].
Infarcts associated with cardioembolism are typically larger than those associated with LAD, partly because the clots are larger and partly because of the insufficiently developed collateral circulation [86]. The onset is usually abrupt, with a gradual progression or fluctuation of symptoms being less common than with LAD. Unlike LAD, multiple vascular territories are often involved.
The occluded vessels are visible when angiography is performed soon after stroke. Although the MCA trunk and/or branches are the most frequently affected, larger vessels such as the ICA or common carotid arteries may be occluded. Clinical symptoms vary according to the size and location of the occluded vessels. Generally, cardiogenic embolic infarcts tend to produce more severe clinical symptoms than those associated with other etiologies. However, small cortical infarcts may produce minor or transient symptoms. Signs of peripheral artery occlusion, such as a reddish discoloration of the skin in distal limbs, are suggestive of embolic stroke.
In cardioembolic infarcts, the embolic materials are generally evanescent. Embolic occlusion of vessels has been detected in >75 % of patients evaluated by angiography within 8 h of stroke onset [87], but in only 40 % when angiography was delayed for up to 72 h [88]. Follow-up angiograms occasionally show recanalization of occluded vessels or migration of emboli from proximal to distal vessels. These characteristics may help differentiate embolic from atherosclerotic in situ occlusion [89]. Due in part to large lesion size and in part to frequent recanalization (and reperfusion), hemorrhagic transformation of an infarct is relatively common, which may result in delay in the initiation of anticoagulation therapy (Fig. 8.9). GRE SVS signs are more frequently observed in patients with cardioembolic than atherothrombotic occlusion [24], since mural thrombi formed in the heart chamber generally contain a large amount of trapped red blood cells.


Fig. 8.9
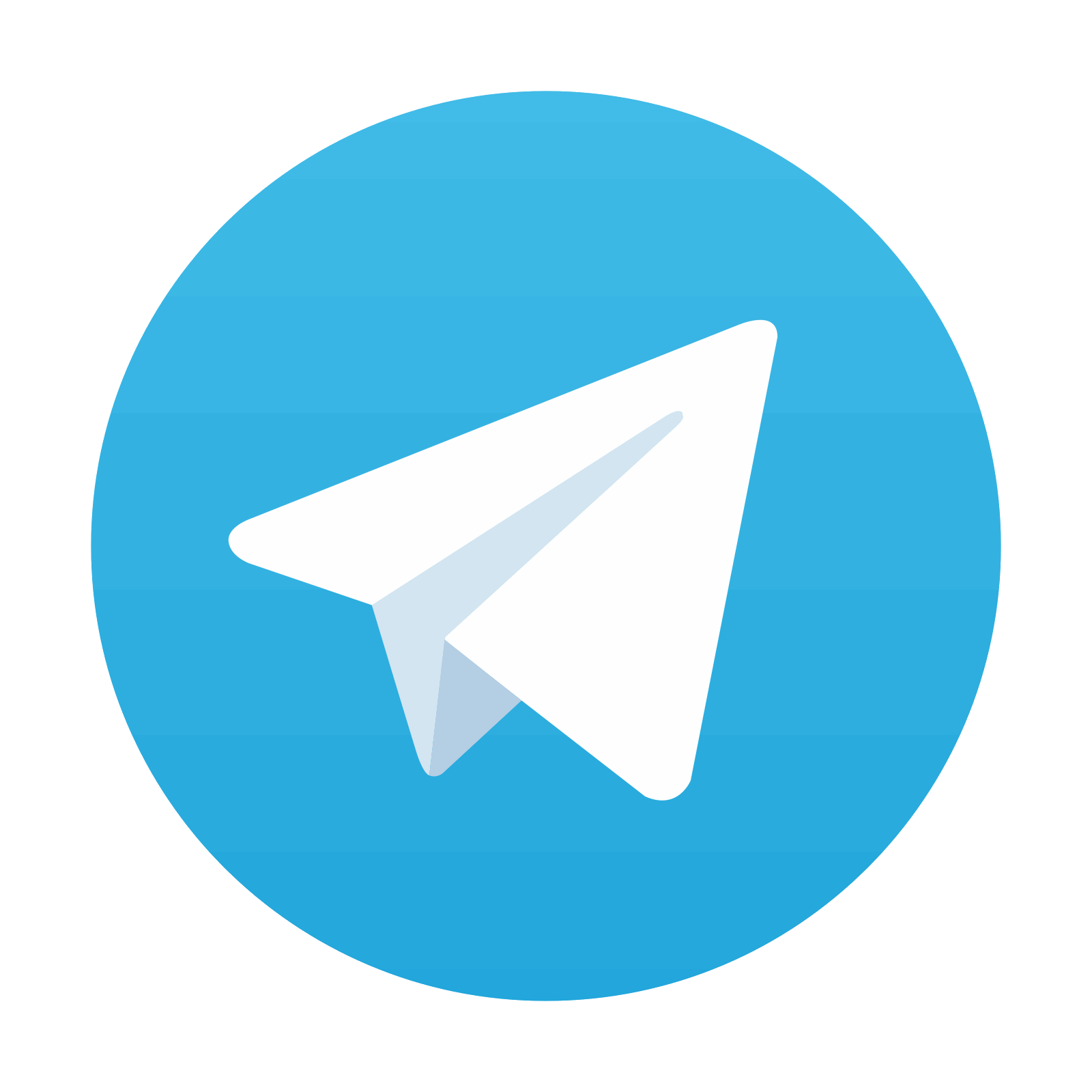
A 75-year-old woman who had had hypertension and atrial fibrillation suddenly developed dysarthria and right hemiparesis. DWI showed an infarct involving the left middle cerebral artery territory (images in the upper row). MR angiography showed occlusion of the left middle cerebral artery (right upper image, thick arrow). Three days later, follow up images (lower row) showed recanalization of the vessel (right image). The infarct size slightly increased and hemorrhagic transformation (thin arrows) is observed. The stroke mechanism was considered a cardiogenic embolism
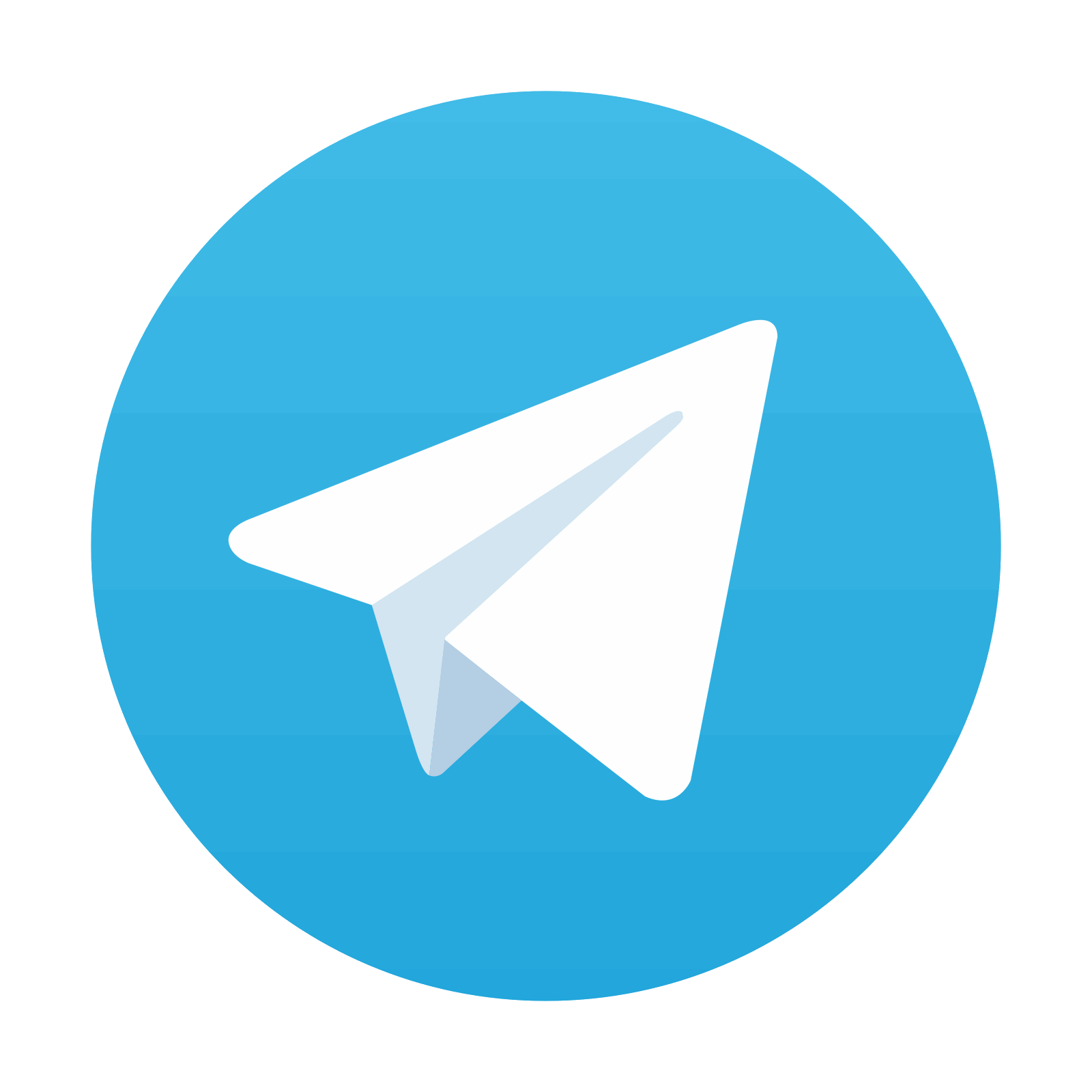
Stay updated, free articles. Join our Telegram channel

Full access? Get Clinical Tree
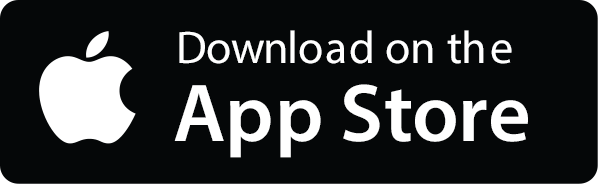
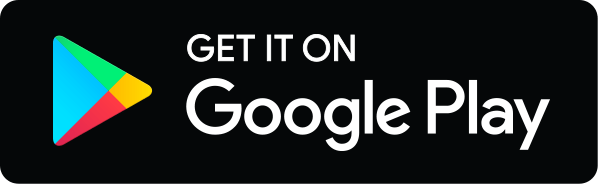
