Fig. 5.1
Potential effects of hyperglycemia over time on pathophysiological processes involved in development of cerebral ischemia
The observed relation between hyperglycemia and poor outcome in patients with ischemic stroke raises the question of whether the outcome can be improved by glucose-lowering treatment. However, improvement in the clinical outcome by administration of glucose-lowering treatment in patients with acute ischemic stroke has not yet been clinically demonstrated. Findings of randomized controlled trials specifically targeting individuals with stroke have failed to show beneficial effects of anti-hyperglycemic treatment. In a meta-analysis of 1,583 patients with acute ischemic stroke from 11 trials, intensively monitored intravenous insulin treatment (aimed at maintenance of glucose concentrations between 72 and 136 mg/dL) was compared with usual care [17]. No intergroup difference was noted in the outcome (odds ratio 1.0, 95 % CI 0.8–1.2), but the risk of symptomatic hypoglycemia was significantly higher in the group treated with insulin (odds ratio 14.6, 95 % CI 6.6–32.2). Currently, there is no clinical evidence that restricting blood glucose level at a particular level during the acute phase of ischemic stroke improves the clinical outcome. The main risk from aggressive hyperglycemia correction in acute stroke appears to be hypoglycemia. Considering this, it may be reasonable to maintain blood glucose in the range of 140–180 mg/dL in all hospitalized patients [2].
5.2 Antithrombotic Therapy in Acute Ischemic Stroke
Aspirin is the most extensively tested antiplatelet agent. The combined results of two large, randomized, unblended intervention studies indicate that aspirin administered within 48 h of stroke onset reduces the risk of recurrence (7 per 1000 patients treated) and death without further stroke (4 per 1000 patients treated) [18–20]. However, it remains to be determined whether aspirin limits the adverse neurological consequences of stroke itself.
Evidences on the use of clopidogrel or dipyridamole in acute stroke are limited. Recently, Wang and colleagues reported the results of the Clopidogrel in High-Risk Patients with Acute Nondisabling Cerebrovascular Events (CHANCE) trial [21]. Among 5170 Chinese patients with acute minor ischemic stroke or transient ischemic attack (onset within the previous 24 h) at high risk of recurrence, the addition of clopidogrel to aspirin reduced the relative risk of recurrent stroke at 90 days by 32 %. However, it is unclear whether clopidogrel facilitates early neurological recovery.
Current literature indicates a small but statistically significant decline in mortality and unfavorable outcomes with the administration of aspirin within 48 h of stroke. The primary effects of aspirin may be attributable to a reduction in early recurrent stroke. Data regarding the utility of other antiplatelet agents, including clopidogrel, with or without aspirin, in acute ischemic stroke are limited.
5.3 Intensive Care
Considerable advances have been made in the management of acute ischemic stroke, including extended time window for intravenous recombinant tissue-type plasminogen activator (rt-PA), development of endovascular devices, and aggressive therapies such as hypothermia induction and craniectomy.
These advances have been paralleled by an increase in the number of ischemic stroke patients requiring intensive care management. Intensive management is generally required for patients receiving intravenous rtPA therapy or endovascular reperfusion therapy and those with large hemispheric or cerebellar infarction, hemorrhagic complications, loss of consciousness, or respiratory compromise. Intensive care is mainly important for neurological monitoring as well as early recognition and management of complications for minimization of secondary neurological injury. Neurointensive care is reported to reduce the duration of hospitalization and improve the outcome at discharge [22].
5.3.1 ICP Monitoring
The main complications of acute ischemic stroke are ischemic brain edema and intracranial hemorrhage (ICH). Symptomatic ICH occurs in 3.6–7.7 % of the patients receiving intravenous or intra-arterial thrombolytic therapy and anticoagulation therapy [2, 23–27]. Massive cerebral infarction is associated with cytotoxic or vasogenic brain edema and ICH, which can in turn increase ICP. Increased ICP can reduce cerebral perfusion, cause tissue hypoxia, and finally lead to brain herniation [28].
Increased ICP can be recognized by serial changes in neurological symptoms, including pupillary dilatation, loss of brainstem reflex, and change in breathing patterns. However, the appearance of these symptoms may indicate that it may be too late for the effective management of ICP. Therefore, AHA/ASA guidelines recommend early monitoring of patients with high risk of brain edema [2]. Establishment of a ventricular drain with an external pressure gauge is the current gold standard for ICP monitoring [29]. This drainage system provides information about ICP as well as intracranial compliance and can be used to control ICP through cerebrospinal fluid drainage. This measurement represents the global ICP. In cases of acute ischemic stroke, focal measurement of ICP may accurately reflect the pressure changes caused by compartmentalization [30]. Intraparenchymal monitoring devices are effective for focal ICP measurement and easier to apply than intraventricular devices. However, these monitoring techniques are invasive and entail a risk of complications, including infection, hemorrhage, and technical problems.
Since most patients are not in a sedated state, it is necessary to develop noninvasive methods of ICP monitoring. One such method is fundoscopy to detect papilledema. However, papilledema develops only several hours after increase in ICP, and absence of papilledema cannot rule out elevated ICP. Ocular ultrasonography has been studied for the assessment of ICP. The diameter of the optic nerve sheath can be determined by measurement of the width measured at a distance behind the optic disc via ocular sonography (Fig. 5.2) [31]. The optic nerve sheath is enlarged when ICP is greater than 15 mmHg and is an easily measured sign of raised ICP [31–33].


Fig. 5.2
Ocular sonography for measurement of optic nerve sheath diameter
Transcranial Doppler (TCD) is also used for ICP monitoring. TCD can provide continuous hemodynamic information about blood flow velocity, pulsatility index (PI), and waveforms of major intracranial arteries. ICP and PI are reported to show a positive correlation in patients with brain injury [34, 35]. However, ICP prediction based on PI should be done with caution because of many possible confounding factors such as arterial stenosis, spasm, arterial pulse, and heart rate [35].
The normal ICP ranges from 5 to 15 mmHg. In general, ICP above 20 mmHg should be actively treated. Cerebral perfusion pressure is measured as the difference between meal arterial pressure and ICP. Because many patients with acute ischemic stroke require blood pressure management, it is necessary to account for both ICP and CPP during ICP monitoring. Before reducing ICP, maintaining the CPP is also important. Maintaining CPP over 60 mmHg is reported to prevent secondary brain injury [36].
5.3.2 Therapeutic Hypothermia
Therapeutic hypothermia is reported to exert a neuroprotective effect and facilitate ICP control in various conditions such as hypoxia, stroke, and traumatic brain injury. The underlying neuroprotective mechanism is believed to involve a reduction in cerebral metabolism, oxygen consumption, glucose metabolism, neuroinflammation, free radical production, and cell death [37]. Various neuroprotective measures have been proposed in cerebral ischemia [38]. In addition, reduction of cerebral blood volume, vasogenic edema, and blood–brain barrier disruption have been identified to be effective in ICP control [37]. Studies have demonstrated improvement of neurological outcome and decrease of mortality achieved by indication of therapeutic hypothermia after cardiac arrest [39, 40]. In experimental studies of stroke, initiation of therapeutic hypothermia early after from stroke onset revealed decrease in infarction volume and improvement in the functional outcome. Small pilot studies have been undertaken in stroke patients, which suggest the potential benefits of therapeutic hypothermia in terms of neurological outcome and mortality.
The optimal time for the initiation of hypothermia induction has not yet been clearly established. However, early initiation of hypothermia has been shown to produce a neuroprotective effect in animal models. Recent human studies on the induction of hypothermia immediately after reperfusion therapy have also shown an improvement in the clinical outcome [41] (Fig. 5.3). Most clinical studies on hypothermia for acute ischemic stroke have mainly focused on mild hypothermia (core temperature of 32–35 °C ) and cooling duration of 5–72 h [41–45]. The cooling duration differs with the purpose of hypothermia induction. Studies based on edema control pertain to the maintenance of hypothermia for a longer duration (34–72 h) [42, 46].


Fig. 5.3
Immediate post-reperfusion cooling. (a) Baseline angiography shows right MCA occlusion. (b) Final angiography reveals complete recanalization. (c) Endovascular cooling catheter (white arrow) is inserted in the inferior vena cava
The rewarming process should also be executed with caution due to the risk of rebound cerebral edema, increased ICP, and rebound hyperthermia. Gradual rewarming, generally at the rate of 0.1–0.25 °C/h, is important to prevent such complications. Recent studies pertaining to the effectiveness of therapeutic hypothermia for ischemic stroke are summarized in Table 5.1.
Table 5.1
Recent studies of therapeutic hypothermia for ischemic stroke
Studies | Cases | Patients | Target temperature | Cooling method | Cooling duration | Clinical outcomes | Notable feature |
---|---|---|---|---|---|---|---|
Martin-Schild et al. [49] | 20 | Cortical ischemic stroke | 33–34.5 °C | Surface or endovascular | 24 h | 50 % of discharge mRS 0–2 | Hypothermia with caffeinol |
Hemmen et al. [50] | 28 | Acute ischemic stroke | 33 °C | Endovascular | 24 h | 17.9 % of mRS 0–1 at 3 months | No differences between hypothermia and normothermia |
Ovesen et al. [51] | 17 | Acute ischemic stroke | 33 °C | Surface or Endovascular | 24 h | Median mRS 3 at 3 months | Feasibility of hypothermia |
Hong et al. [45] | 39 | Anterior circulation | 34.5 °C | Surface or Endovascular | 48 h | 48.7 % of mRS 0–2 at 3 months | Cooling after recanalization |
Piironen et al. [52] | 18 | Acute ischemic stroke | 34.5 °C | Surface | 23 h (median) | 38.9 % of mRS 0–2 at 3 months | Cooling after iv-rtPA |
Hwang et al. [41] | 18 | Anterior circulation | 34 °C | Endovascular | 51 h (median) | 55.6 % of mRS 0–2 at 3 months | Immediate post-reperfusion cooling |
The commonly used cooling methods are surface cooling and endovascular cooling. Surface cooling systems consist of cooling blankets or surface pads and offer the advantages of easy application, rapid initiation, and relatively low rate of complications. However, the disadvantages of this method are frequent shivering, skin irritation, and difficulty in maintaining a steady temperature. In the endovascular cooling method, a cooling catheter is introduced through the femoral or subclavian vein. Cooling is achieved by circulating cooled saline within the cooling catheter. This system offers the advantage of accurate temperature control during maintenance and rewarming period. However, no differences have been reported in the outcomes achieved with both cooling methods [47]. Potential risks associated with induction of hypothermia are infection and venous thrombosis.
The most frequent complication is shivering, which can interfere with the cooling process and increase the rate of systemic metabolism [48]. In conscious patients, endovascular cooling devices are more effective in reducing shivering than surface cooling devices. Assessment of the severity of shivering using the Bedside Shivering Assessment Scale is useful for early intervention [48]. According to literature, oral buspirone and intravenous meperidine exert an anti-shivering effect. Skin warming is also reported to be effective in controlling shivering. The cardiopulmonary complications associated with hypothermia include bradycardia, arrhythmia, hypotension, pneumonia, and pulmonary edema. Electrolyte imbalance such as hypokalemia, hypomagnesemia, hypophosphatemia, and hypocalcemia has also been reported as accompanying complications. Once therapeutic hypothermia is induced, it is difficult to recognize complications by clinical symptoms. Therefore, continuous monitoring via serial chest radiography and laboratory tests is important for the detection of complications.
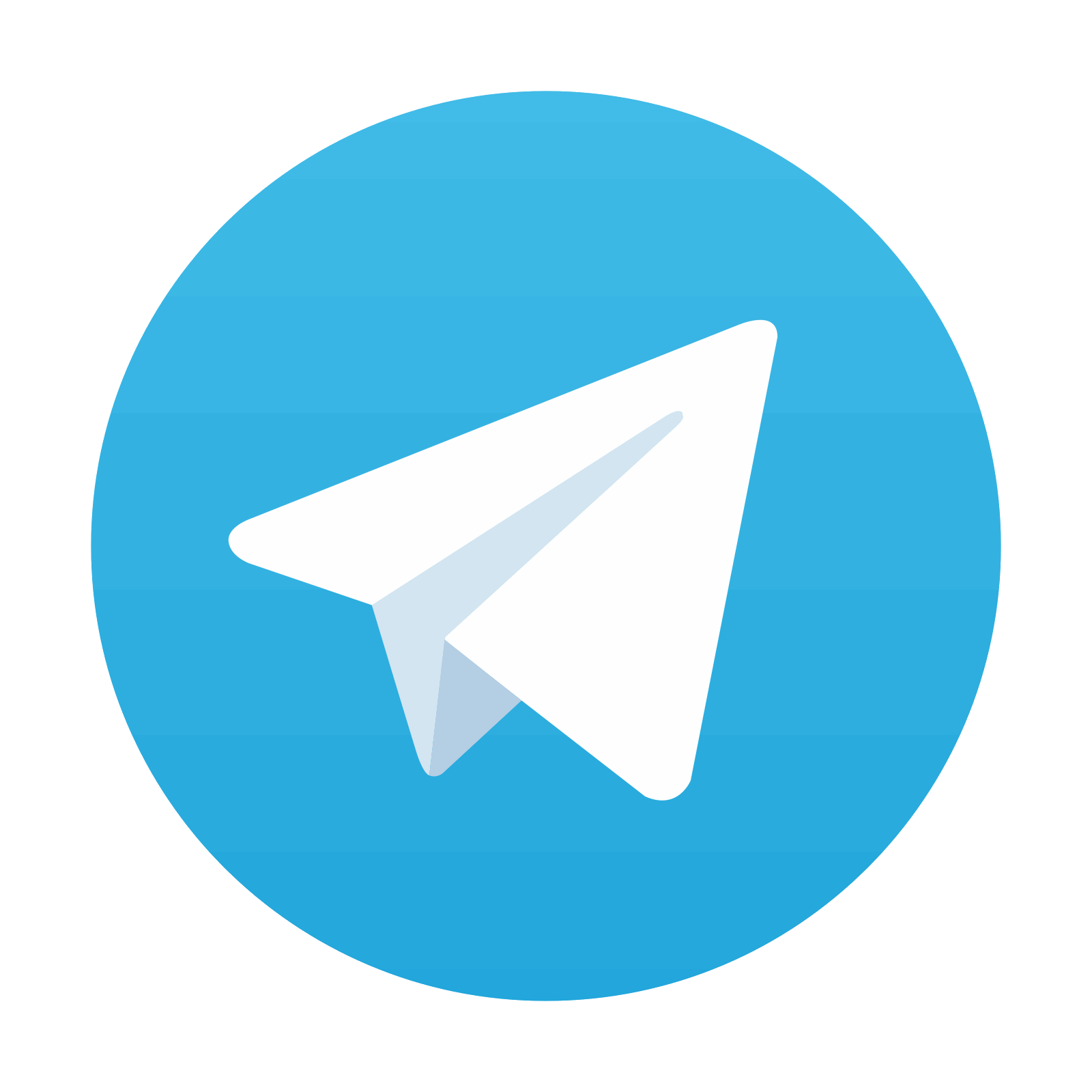
Stay updated, free articles. Join our Telegram channel

Full access? Get Clinical Tree
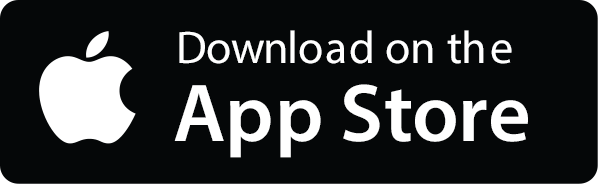
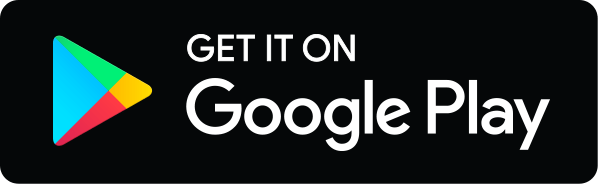