Fig. 1.1
Classification of neuroglia
Despite a remarkable heterogeneity of neuroglial cells they have one feature in common: the main function of neuroglia is the preservation of nervous system homeostasis. This function is accomplished differently by different cells and they naturally have their specializations. Yet they are indispensable for housekeeping of the nerve tissue: astrocytes assume responsibility for multiple homeostatic matters in the CNS, oligodendrocytes provide for the maintenance of interneuronal connectivity (or connectome, as it is often referred to today), microglial cells are responsible for defensive homeostasis; similarly in the periphery satellite glia, enteric glia and olfactory ensheathing cells oversee various homeostatic functions, whereas Schwann cells support nerve impulse propagation.
The range of homeostatic functions of neuroglial cells is extremely wide. Astrocytes are responsible for the structural organization of the nervous tissue, for neurogenesis and development of the CNS, for generation and maintenance of the blood- and cerebrospinal fluid (CSF)-brain barriers, for homeostatic maintenance of neurotransmitters and ions, for synaptogenesis and metabolic support, for scavenging of reactive oxygen species and for regulation of local blood flow (Table 1.1). Astrocytes are also central for various systemic functions such as central chemoception (Shimizu et al. 2007; Gourine et al. 2010; Gourine and Kasparov 2011) and regulation of sleep (Blutstein and Haydon 2013) (Table 1.1). Microglial cells exert multiple trophic effects and are actively involved in shaping the synaptic connections by removing silent or redundant synapses. NG2 cells receive synaptic inputs which might be fundamental for life-long control over myelinating capabilities of the brain tissue. Finally, neuroglial cells are secreting elements of the nervous tissue which release multiple factors that are indispensable for the survival and plasticity of neural networks.
Function | Involvement/tasks |
---|---|
Development of the CNS | Neurogenesis Neural cell migration and formation of the layered grey matter Synaptogenesis |
Structural support | Parcellation of the grey matter though the process of tiling Delineation of pia matter and the vessels by perivascular glia Formation of neuro-vascular unit |
Barrier function | Regulation of formation and permeability of blood-brain and CSF-brain barriers Formation of glial-vascular interface |
Homeostatic function | Control over extracellular K+ homeostasis through local and spatial buffering Control over extracellular pH Regulation of water transport Removal of neurotransmitters from the extracellular space |
Metabolic support | Uptake of glucose; deposition of glycogen Providing energy substrate lactate to neurons in activity dependent manner |
Synaptic transmission | Regulation of synapses maintenance and assisting in synaptic pruning Providing glutamate for glutamatergic transmission (through de novo synthesis and glutamate-glutamine shuttle) Regulating synaptic plasticity Integrating synaptic fields Providing humoral regulation of neuronal networks through secretion of neurotransmitters and neuromodulators |
Regulation of blood flow | Regulate local blood supply (functional hyperemia) through secretion of vasocontrictors or vasodilators |
Higher brain functions | Chemoception—regulation of body Na+ homeostasis Chemoception—regulation of CO2 and ventilatory behavior Sleep Memory and learning |
Brain defense, neuroprotection and post injury remodeling | Isomorphic and anisomorphic reactive astrogliosis Scar formation Catabolizing ammonia in the brain Immune responses and secretion of pro-inflammatory factors (cytokines, chemokines and immune modulators) |
1.2 Neuroglial Cells Are the Central Elements of Brain Defense
Homeostatic functions of neuroglia also extend to forming the defense of the nervous system. Every insult to the nerve tissue triggers glial homeostatic response and initiates a specific glial defensive reaction. The homeostatic response is primarily neuroprotective. For instance, astrocytes , using multiple molecules contributing to maintaining normal brain environment, contain the damage and sustain neuronal survival by maintaining CNS homeostasis. In conditions such as brain ischemia/hypoglycemia, astroglial cells sustain brain metabolism by mobilizing glycogen stores; breaking glycogen to lactate that is transferred to adjacent neural elements where it is used aerobically as fuel (Brown and Ransom 2007; Suh et al. 2007) . Similarly, microglia respond to damage by releasing multiple factors that have neuroprotective and trophic effects.
In addition, neuroglial cells are in possession of evolutionarily conserved defensive program that is generally referred to as reactive gliosis (Parpura et al. 2012) . Reactive changes in response to pathology are characteristic for all types of glial cells, and can be sub-classified as reactive astrogliosis , reactive activation of NG2 cells and activation of microglia. Oligodendroglia and Schwann cells are also activated in response to axonal injury by participating in Wallerian degeneration. Conceptually, reactive gliosis is a defensive reaction that is aimed at protecting stressed neurons, isolating injured area, removing pathogens, dying cells and cellular debris, and remodeling the nerve tissue after the resolution of pathology.
Reactive astrogliosis is activated in many (if not all) types of CNS pathology. Reactive astrogliosis is often associated with hypertrophy and proliferation of astrocytes along with an up-regulation of cytoskeletal components such as glial fibrillary acidic protein (GFAP) , vimentin or nestin (Pekny and Nilsson 2005; Sofroniew 2009; Sofroniew and Vinters 2010) ; an increase in the immunoreactivity of GFAP is generally considered as a specific marker for astrogliotic response. Astrogliosis is commonly regarded as a pathological glial reaction with a negative outcome; often astrogliosis is regarded as a sign of neuroinflammation . In contrast to this general belief, astrogliosis is primarily a defensive, survivalist response which develops in a multi-stage graded and controlled fashion (Sofroniew 2009; Sofroniew and Vinters 2010; Verkhratsky et al. 2012) . Astrogliosis results in remodeling of astroglial properties and functions, which are specific for various pathological contexts and resolve in different ways. Conceptually, reactive astrocytes increase neuroprotection and trophic support of neurons under stress, form an astroglial scar that isolates the damaged area from the rest of the CNS, promote post-lesion regeneration of the nervous tissue, and are instrumental in the reconstruction of the compromised blood–brain barrier. All these events are beneficial for nervous tissue and suppression of astrogliotic response exacerbates CNS damage (Sofroniew and Vinters 2010; Robel et al. 2011) .
Reactive astrogliosis can be isomorphic (i.e. preserving morphology) or anisomorphic (i.e. changing the morphology) , these two types of reactive changes are associated with different functional responses and outcomes. In isomorphic gliosis, astrocytes undergo hypertrophy associated with changes in physiology, biochemistry and immunological presence, all of these, however, proceeding without altering normal astroglial domain organization. Isomorphic astrogliosis facilitates neurite growth and synaptogenesis, thus being permissive for the regeneration of neuronal networks. Importantly, isomorphic gliosis is fully reversible, and astrocytes return to a healthy state after the resolution of pathology. In anisomorphic gliosis, astroglial hypertrophy is associated with proliferative response, which results in the disruption of astroglial domains and in the formation of a permanent glial scar; this scar prevents any axonal growth due to the presence of chondroitin and keratin, which are secreted by the anisomorphic reactive astrocytes (Sofroniew 2009; Sofroniew and Vinters 2010; Verkhratsky et al. 2012) .
Similar to astrocytes, insults to CNS induce reactive response of NG2 glia, characterized by an increase in proliferation and morphological remodeling, the latter being manifested by shortening and thickening of the cellular processes and a strong increase in the expression of NG2. In the spinal cord, activated NG2 cells generate new oligodendrocytes that remyelinate the demyelinated lesions (Tripathi et al. 2010; Zawadzka et al. 2010) . Being a source of chondroitin sulphate proteoglycan, NG2 cells may also contribute to scar formation.
Microglial cells represent another important component of gliotic response. It has become increasingly clear that the microglial activation is primarily a defensive reaction, which develops in many stages and results in multiple phenotypes, many of which are neuroprotective (Hanisch and Kettenmann 2007; Ransohoff and Perry 2009; Kettenmann et al. 2011) . Activation of microglia progresses between different stages and phenotypes with a variety of morphological, biochemical, functional and immunological changes. Local insults induce rapid converging of microglial processes to the injured locus (Davalos et al. 2005; Nimmerjahn et al. 2005) . Stronger insults, however, induce morphological remodeling where microglial somata enlarges and processes retract to become fewer and thicker; this parallels with changes in the expression of various enzymes and receptors, and with increased production and release of immune response molecules. Some of the activated microglia enter into a proliferative mode. With persisting insult, microglial cells become motile and using amoeboid-like movements they gather around the sites of damage; the ultimate climax of this activation is the transformation of microglial cells into phagocytes (Hanisch and Kettenmann 2007; Ransohoff and Perry 2009; Boche et al. 2013) .
Lesions to peripheral nerves and to the white matter also set in motion reactive changes in myelinating cells, Schwann cells and oligodendrocytes, respectively, that determine the functional preservation of neural connectome. Activation of myelinating cells also take a part in Wallerian degeneration, the coordinated programme, which progressively results in the disintegration of the distal axonal segment, the removal of myelin and remodeling of myelinating cells, and finally in the nerve fiber regeneration, by the axonal growth from the nerve stump proximal to the site of insult (Vargas and Barres 2007; Rotshenker 2011) . In the peripheral nervous system, severance of the axon triggers proliferation of Schwann cells , which eventually form the pathway/scaffold for axonal regeneration. In the CNS the balance between de- and remyelination (which involves both oligodendroglia and NG2 cells) defines the course of demyelinating pathology (Franklin and Ffrench-Constant 2008; Miron et al. 2011; Kipp et al. 2012) .
Neuroglial contribution to the pathology can, however, exert not only neuroprotection, but can be also neurotoxic. This represents an intrinsic dichotomy of every homeostatic system, where in conditions of extreme stress the system acquire a deleterious scope. The very same molecular cascades that protect brain environment can also contribute to neuronal death. Overstimulation of astrocytes can induce the release of glutamate through various existing release pathways and this release can add to the excitotoxicity . Abnormal water transport through astroglial aquaporins can contribute to edema, and astroglial failure of K+ buffering is contributing to spreading depression. Similarly, over-activation of microglia results in the release of neurotoxic factors and phagocytic activity can further assist neuronal death.
1.3 Neuropathology as Homeostatic Failure: Central Role of Glia in Neurological and Psychiatric Disorders
Neurological diseases are, conceptually, failures of tissue homeostasis. The systemic homeostatic function of neuroglia makes these cells fundamental for evolution and outcome of neuropathology. The neuron-centric view still dominates the thinking of neuropathologists, neurologists and psychiatrists, which is reflected by fundamental and clinical research that is mainly concentrated on reactions of neurons in the contexts of various types of neurological and psychiatric disorders . Nonetheless, evolution of the nervous system went through specialization that resulted in the appearance of two interdependent, yet interacting, cellular networks, the executive system represented by neurons which perfected their ability for rapid signaling, and the housekeeping systems represented by neuroglia, which assume full responsibility for keeping neurons alive and ascertain their proper functioning. As such neurons have little capability to counterbalance environmental stress and pathological invasions , and it is indeed the neuroglia that protects and maintains the nervous system operation. When and if the neuroglial homeostatic and defensive mechanisms are exhausted, the neural tissue dies. While these views of central role for neuroglia in neuropathology began to be explored only recently (Giaume et al. 2007; Rajkowska and Miguel-Hidalgo 2007; De Keyser et al. 2008; Heneka et al. 2010; Cheng et al. 2012; Coulter and Eid 2012; Goldman et al. 2012; Molofsky et al. 2012; Verkhratsky et al. 2012; Benedetto and Rupprecht 2013; Parpura and Verkhratsky 2013; Rajkowska and Stockmeier 2013; Seifert and Steinhauser 2013) , it is, however, becoming increasingly clear that brain pathology is, to a very great extent, a pathology of glia , and it is glial defensive capabilities that define the outcome and the scale of neurological deficit.
Gliopathology can be primary or secondary. Primary gliopathologies are represented, for instance, by the thus far only known genetic glial disease, the Alexander disease , in which an expression of mutant GFAP results in the aberration of astrocyte function and severe failure in white matter development (Messing et al. 2012) . Similarly, astroglial cells are the primary targets in various toxic encephalopathies such as Wernicke-Korsakoff syndrome, hepatic encephalopathy or poisoning by heavy metals; the common denominator of all these disorders is the astroglial failure to contain extracellular glutamate (because of specific down-regulation of astroglial glutamate transporters) with ensuing excitotoxicity and neuronal death (Hazell 2009; Hazell et al. 2009; Brusilow et al. 2010; Butterworth 2010; Ni et al. 2012; Sidoryk-Wegrzynowicz and Aschner 2013) . Secondary gliopathologies contribute to a variety of acute neurological disorders such as trauma, stroke or infection; they involve astrocytes, which define spreading of ischemic insults through the penumbra, and microglia , which act as a common target for many viruses. The nature of glial contribution in chronic diseases such as neurodegenerative disorders , neurodevelopmental disorders and neuropsychiatric disorders could be either secondary (when glia reacts to a neuronal disease specific lesion) or primary when impairment of glial function can assume etiopathological role; this matter yet requires detailed investigation.
1.4 Glial Asthenia and Paralysis Define Neuropathological Progression
A relatively new aspect in gliopathology associated with glial asthenia, atrophy and functional “paralysis” began to be unfolding in recent years. It turned out that various neuropathologies are associated not only with reactive glial responses, but include gliodegenerative changes that may underlie diminution of glial homeostatic reserve with negative consequences for neuroprotection and normal functioning of the neural networks (Fig. 1.2). Atrophic changes in astroglia are seen, for example, at the early stages of amyotrophic lateral sclerosis (ALS) , when functional atrophy and apoptosis of astrocytes precede the death of motoneurons. In ALS, astrocytes reduce their glutamate uptake, which contributes to increased excitotoxicity (Rossi and Volterra 2009; Staats and Van Den Bosch 2009) . Incidentally, selective silencing of the ALS related mutant superoxide dismutase 1 expression in astrocytes delays the progression of ALS in transgenic mice (Wang et al. 2011) . Early atrophic changes in astroglia were also observed in transgenic animal models of the Alzheimer’s disease (AD) (Verkhratsky et al. 2010; Rodriguez and Verkhratsky 2011) . In these animals, substantial functional heterogeneity of astroglial responses to pathology is also found. In particular, it appeared that astrocytes in entorhinal cortex were unable to mount astrogliotic reaction in response to tissue deposition of β-amyloid; this failure in defensive function may underlie high vulnerability of certain brain regions to AD-like pathology (Yeh et al. 2012) . Similarly functional paralysis of microglia that fails to clear β-amyloid contributes to AD progression. Inhibition of microglial inflammasome shifted the microglial activation towards the neuroprotective phenotype and reduced β-amyloid load (Heneka et al. 2013) . Similarly, astroglial asthenia and atrophy are manifested in major neuropsychiatric disorders such as schizophrenia and major depressive disorders; changes in astrocytes are substantially more prominent than those of neurons and may indicate a primary role for gliopathology in these diseases (Rajkowska et al. 2002; Hercher et al. 2009; Rajkowska and Stockmeier 2013; Williams et al. 2013) . Similarly, loss of function of microglia in neurodevelopment is implicated in the pathogenesis of certain types of autistic spectrum disorders such as trichotillomania (Chen et al. 2010) .
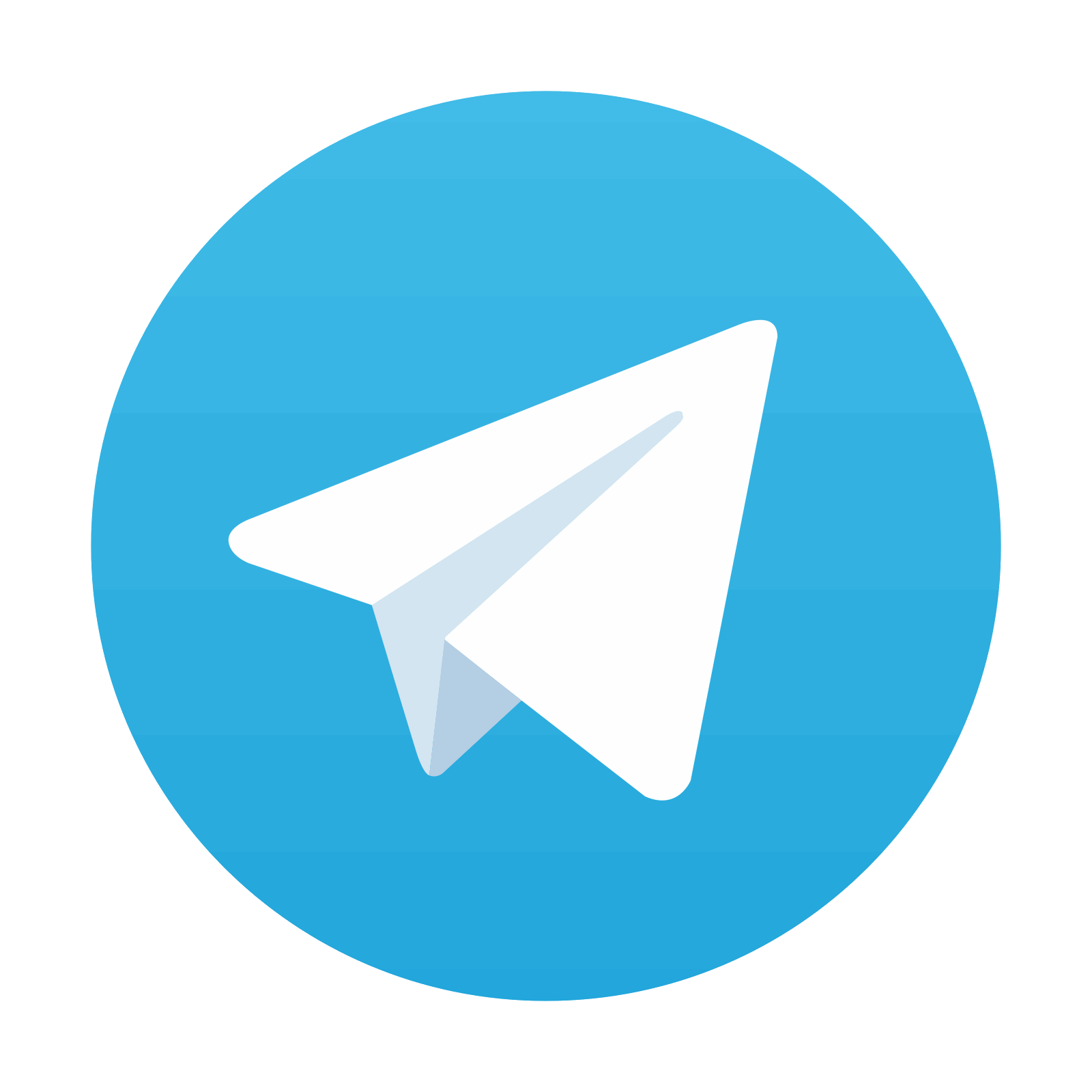
Stay updated, free articles. Join our Telegram channel

Full access? Get Clinical Tree
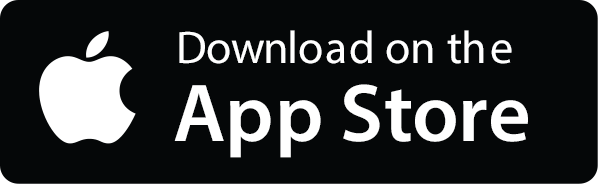
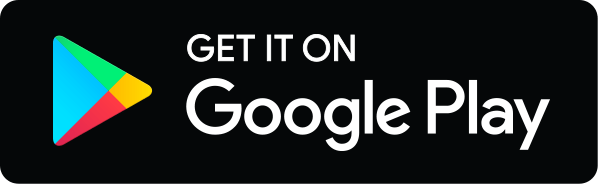