(1)
Division of Neurosurgery, Federal University of Triângulo Mineiro, Uberaba, Minas Gerais, Brazil
2.1 Historical Evolution
2.1.1 Pioneers
The first neuroendoscopic procedure reported in the literature was conducted by Victor Darwin Lespinasse (1878–1946) (Fig. 2.1) in 1910 [1]. Lespinasse, a urologist from Chicago, was also famous for being a pioneer and a defender of testicular transplantation. The neuroendoscopic procedure he reported consisted of bilateral choroid plexus coagulation, making use of a small cystoscope. In this first experiment, two children were treated; one died in the immediate postoperative period, while the other lived on for another 5 years. Lespinasse did not officially record this adventure in the scientific literature, he merely introduced the method to the local medical society and mentioned it in his application, in 1913, to the American College of Surgeons, in which he cited the “destruction of the choroid plexus for internal hydrocephalus” among his surgical areas of interest. He would later tell his daughter Victoire, an obstetrician also from Chicago, that this pioneer procedure was “an intern’s stunt” [2].

Fig. 2.1
Victor Darwin Lespinasse (1878–1946)
The great pioneer of neuroendoscopy was Walter Edward Dandy (1886–1946) (Fig. 2.2), from Johns Hopkins School of Medicine, in Baltimore. He is considered to be the father of pediatric neurosurgery and also neuroendoscopy, because of the great knowledge of ventricular anatomy he acquired from his ventriculography studies, a technique that permitted visualization of the ventricular system for the first time (Fig. 2.3). Dandy said, with respect to ventriculography:
“All of the injections have been made in children varying from 6 months to 12 years of age. Invariably, the lateral ventricle has been sharply outlined in the radiogram. In two instances, the third ventricle and the foramen of Monro were visible. In none, however, have we observed the fourth ventricle or the aqueduct of Sylvius. The practical value from pneumoventriculography is expected principally from the shadows of the lateral ventricles” [3].
In 1922, Dandy published the first endoscopic observations of the ventricles through the use of an endoscope illuminated by an external light reflection in a mirror, coining the term “ventriculoscopy” [4] (Fig. 2.4), in which he said:
“It was possible to see practically the entire extent of the lateral ventricle, the foramen of Monro, the septum pellucidum with numerous perforations in it, and the entire extent of the choroid plexus …” [5].
However, despite the good visualization achieved with “ventriculoscopy”, resection of the choroid plexus, a common technique at the time, was not possible with the use of the ventriculoscope. Dandy also stated:
“The remarkable visualization of the ventricular system provided by the ventriculoscope did not surpass the images produced by the more routine pneumoventriculography” [6].
He continued with his endoscopic experiences, and in 1923, using a small cystoscope, he performed resection of the choroid plexus in two patients [7], followed by others in the following years, and he achieved results similar to those of a craniotomy [8] (Fig. 2.5). After years of enthusiasm, at the end of his brilliant career, Dandy said, of ventriculoscopy:
“Its usefulness is probably restricted to infants and young children, and to those tumors which are accidentally disclosed during choroid plexectomies rather than tumors, however small, that are causing obstruction to the ventricular channels” [9].

Fig. 2.2
Walter Edward Dandy (1886–1946)

Fig. 2.3
From the initial studies of Dandy, pneumoventriculography in a 3-year-old child with tuberculous meningitis. It is possible to see “the third ventricle (a) and probably the foramen of Monro (b)” according to his own words (Reprinted from Dandy [3])

Fig. 2.4
Publication of 1922 by Dandy, after his first endoscopic observations (Reprinted from Dandy [5])

Fig. 2.5
Description of Dandy’s technique for removal of the choroid plexus of the lateral ventricles using two Kelly cystoscopes (Reprinted from Dandy [9])
In Germany, the pioneer of neuroendoscopy was Erwin Payr (1871–1946), professor of neurosurgery at the University of Leipzig. He was known for the use of venous grafts for draining cerebrospinal fluid (CSF) from the ventricles to the superior sagittal sinus and jugular vein [10]. Payr presented the tools, preliminary tests, and the technical feasibility of “Enzephaloskopie” to Leipzig Medical Society in 1919 [2]. William Jason Mixter (1888–1958) (Fig. 2.6), chief of the Neurosurgical Service of Massachusetts General Hospital, in Boston, from 1933 to 1946, performed the first endoscopic third ventriculostomy (ETV) in 1923. He was also famous for being the pioneer in recognizing the relationship between herniated intervertebral discs and radicular syndromes, and published the report of the first successful discectomy, in 1934 [11]. This is still a topic for debate, because, interestingly, another pioneer of neuroendoscopy, Dandy, published a surgical report of “loose cartilage from intervertebral disk simulating a tumor of the spinal cord” in 1929 [12]. Fay and Grant, from Philadelphia, reported the first images of dilated infant ventricles via a cystoscope coupled with a camera in 1923 (Fig. 2.7). The exposure time for the acquisition of these images was up to 75 s [13]. Also in 1923, Johannes Allwill Max Volkmann (1889–1982), professor at the University Surgical Clinic in Halle, Germany, published his first experiences with “Enzephaloskopie”. After some tests on cadavers, the first ventricular inspection was carried out on a 3-year-old child with hydrocephalus. In the beginning an infant cystoscope was used, but then an “Enzephaloskop” was designed that was lighter and easier to handle. It had a 22-cm sheath with a 6-mm outer diameter (OD), along with two irrigation holes. The optics were introduced inside the sheath, allowing rotation once inside the ventricle [14, 15].

Fig. 2.6
William Jason Mixter (1888–1958)

Fig. 2.7
The first register of ventricular endoscopic images. The exposure time for the acquisition of these images was up to 75 s (Reprinted from Fay and Grant [13])
In 1934, Tracy Jackson Putnam (1894–1975) (Fig. 2.8), a great neurologist and neurosurgeon from New York Neurological Institute at Columbia University, was aiming to carry out a plexectomy again, the procedure introduced by Dandy years earlier. For the procedure, Putnam described a new instrument called a “ventriculoscope,” for the purpose of cauterizing the choroid plexus in children with communicating hydrocephalus [16, 17]. This instrument had a length of 10 cm with a 6-mm OD (small model) or a length of 11 cm with a 7-mm OD (large model). With such equipment, bilateral choroid plexus coagulation was carried out in seven children with hydrocephalus, with “encouraging” results [18]. John Edwin Scarff (1898–1978) (Fig. 2.9) was the head of the Department of Neurological Surgery at Columbia University, New York, in 1935 [19]. In 1936, he published his first experience with an endoscopic plexectomy using a new apparatus [20]. His ventriculoscope, very similar to a cystoscope, was 10 cm long with a 6-mm OD (small model) or 18 cm long with a 7-mm OD (large model). The equipment permitted continuous irrigation in order to maintain constant intraventricular pressure and prevent ventricular collapse. This fact was, presumably, the cause of the bad initial results reported by Dandy, who stated in 1918:
Following the escape of the fluid, the thin brain walls collapse. … The mechanical kinking of the larger vascular trunks by angulations of the enfolding brain must have a pronounced effect upon the circulation [21].
In Scarff’s system a movable coagulation probe was also included, allowing a large range of movement, making it possible to coagulate a large area of the choroid plexus. Through the same channel, another mobile probe was also created, with the purpose of perforating the floor of the third ventricle. Scarff published a report of a series of 19 patients in 1951, 15 of whom had a good outcome with a permanent reduction in intracranial pressure; 3 did not benefit from the intervention and 1 patient died [4, 22, 23].

Fig. 2.8
Tracy Jackson Putnam (1894–1975)

Fig. 2.9
John Edwin Scarff (1898–1978)
2.1.2 Ostracism of Neuroendoscopy
The decades of the 1950s and 1960s represented a period of ostracism of the practice of neuroendoscopy. This was due to the advent of ventricular shunts, the development of microneurosurgery, and above all, the technological limitations of the time [24]. In 1949, Frank Nulsen and Eugene Spitz performed the first surgery to install a shunt for hydrocephalus [25], and also, in the decade following the introduction of microsurgical techniques, there were extraordinary advances, contributing to the temporary overlooking of neuroendoscopy. The microscope provided the neurosurgeon precisely what neuroendoscopy did not provide: adequate lighting, magnification, and the ability to access deep structures without incurring adjacent brain damage. In the 1960s, despite the stagnation in neuroendoscopy, there were major technological advances that allowed the resurgence of endoscopic techniques [26]. These advances correspond to the invention of a system of lenses with a variable refractive index by Harold Horace Hopkins (1918–1994) (Fig. 2.10), the invention of charged-couple devices (CCDs), and improvements in fiber optic technology [27]. Of these, undoubtedly, the technology of Hopkins deserves to be highlighted; it consisted of a system composed of a glass tube with thin lenses made of air, a rod-lens system (Fig. 2.11) [28]. This system is the basis of current rigid neuroendoscopy, and is the technology purchased by Karl Storz [29]. The Hopkins system, incredibly, increased the field of view, color rendition, and resolution by approximately 10 times compared with previous endoscopes, and it is perhaps for this reason that Hopkins has been nominated twice for the Nobel Prize in Physics [30]. Charged-couple devices (CCD) are small semiconductor sensors that capture images formed by an integrated circuit containing an array of coupled capacitors. Their unique ability to convert optical signals into electrical impulses, approximately 10 times more sensitive to light than photographic film, has made them vital to the miniaturization of the equipment, making it lighter to handle [27, 31]. Also, advances in fiber optic technology allowed for the use of powerful light sources that could be placed distally and transmitted with increasing efficiency to the structures of interest. These extraordinary advances in optics, which minimized the loss of reflected light and improved video capture methods, have led to a revival of neuroendoscopy and a great interest in the method in the past 20 years [24].

Fig. 2.10
Harold Horace Hopkins (1918–1994)

Fig. 2.11
Standard endoscope (above) and Hopkins rod-lens system (below). The glass rods in the Hopkins telescope provide a larger image, greater light transmission, and clarity of vision improved by approximately 10 times compared with previous endoscopes (Image courtesy of Karl Storz, Tuttlingen, Germany)
2.1.3 Rebirth
The revival of neuroendoscopy occurred only thanks to technological development. In this phase, the contribution of Gerard Guiot (1912–1998), from Paris, is primarily highlighted [32, 33]. His contribution was made thanks to the innovation introduced by Fourestier and Vulmière, from the Optical Institute of Paris. The innovation consisted of the installation of the light source not at the distal end of the endoscope, but at the proximal end, on the outside, in a separate optical apparatus. Thus, it was possible to regulate the light intensity from the outside. With this innovation, the light intensity was improved, allowing photos and video recordings, and above all, safer handling of instruments in the ventricular environment [2]. This innovation was first used by Guiot in 1962, at the Foch Hospital in Paris, in a patient with a cystic tumor bilaterally occluding the foramen of Monro. The endoscopic procedure was performed with a tumor puncture and a third ventriculostomy. Two months after the endoscopic procedure, the tumor was completely removed under a microsurgical approach, and it was possible to show the third ventricle floor with the patent ventriculostomy. The patient had a favorable progression, and returned to work 2 months after the microsurgical procedure. A second neuroendoscopic procedure was also carried out in 1962, on a 3-month-old child with obstructive hydrocephalus; a third ventriculostomy was performed, with good patient progression [2]. Another great achievement of this revival period occurred in 1973. Takanori Fukushima, from Tokyo, was the first to use a flexible endoscope. This “ventriculofiberscope” had a diameter of 4 mm, a flexible end, and a working channel. With this equipment 37 procedures were performed, which included biopsies, septostomies, and ventriculostomies [34, 35]. Other achievements also in this phase included the development of the first endoscopy system based on the Hopkins optic system, by Griffith, from Bristol in the United Kingdom. This device had an OD sheath of 4.5 mm, and plexectomies, aqueductoplasties, and biopsies were performed with it [36, 37]. Also, in 1977, Apuzzo and colleagues, from Los Angeles, published a pioneer work on the application of the Hopkins system to several neurosurgical procedures. As an innovation, the work introduced optics with angles from 70° to 120° in cranial, spinal, and transfenoidal surgeries [28].
2.2 Basic Techniques for Ventricular Approaches
Neuroendoscopy has attracted great attention in the past two decades due to the technological development of optical systems, cameras, and monitors, providing great safety and elegance for the procedures. With such advances, the endoscope has been definitively added to the modern equipment arsenal of the neurosurgeon. The neuroendoscope, when compared with the surgical microscope, provides a completely different view, with advantages and disadvantages (Fig. 2.12). Current systems of rigid endoscopy with the Hopkins rod-lens system (Karl Storz, Tuttlingen, Germany), those most used worldwide, provide a field of view resulting in an excellent panoramic vision (wide angle), and even lesions not located directly in front of the endoscope can be easily recognized. There are also flexible endoscopy systems, based on a bundle of optical fibers which – despite allowing for broad angulation for viewing inside the ventricle – do not have the same image resolution, which is lower than that of rigid endoscopy [38, 39]. As a result of the aspect of a panoramic view and greater ease in identifying intraventricular structures, the orientation during endoscopic navigation is extremely safe. This feature is important, because in neuroendoscopy, the surgeon works in a narrow and deep corridor. Endoscopes with angled optics permit “looking around the corner” or behind vascular structures, which is a very positive feature of microsurgeries that are controlled and assisted by neuroendoscopy [38, 39]. The vast majority of ventricular endoscopic procedures may be performed with non-angled systems. The use of endoscopes allows viewing of the neurosurgical target with impeccable lighting, even in a very deep field. Another important advantage of the endoscope over the microscope is the excellent depth of field, so there is no need to adjust the focus during the procedure, a feature which is required with the use of the microscope, particularly at high magnifications. Obviously, the endoscope also has its limitations. The most striking is the lack of stereoscopic vision. The view provided by the endoscope is a “fish eye” view, which is a kind of pseudo three-dimensional (3D) view. This aspect is partly influenced by a phenomenon called parallax, a term derived from astronomy, which is the impression that objects displayed closer to the endoscope move more than more distant objects, and this contributes to the pseudo 3D effect [38, 39]. Intraventricular navigation in the absence of a stereoscopic view is compensated by training. Training should be started mainly on the simplest cases, using non-angled optics. The lack of image resolution is another disadvantage of the endoscope compared with the microscope, which has objective lenses with a larger diameter. Another reason for the good resolution of the microscope is that the surgeon is looking directly through the lens system and the human retina is the sensor that captures the images. With the endoscope, on the other hand, this sensor is the CCD located on the head of the camera coupled to the optics. Even with the recent introduction of high-definition cameras, which generate images of 1080 lines and 2 million pixels, this image is still not comparable to the power of resolution of the human retina [39]. Currently, cerebral endoscopy can be classified in terms of endoscopic neurosurgery, endoscope-controlled microneurosurgery, and endoscope-assisted microneurosurgery [38, 40]. Endoscopic neurosurgery or ventricular neuroendoscopy (“channel endoscopy”) basically consists of the use of the neuroendoscope in the ventricular cavity, with the instruments being employed through a working channel in the endoscope itself. The neuroendoscope is used to navigate in the ventricular liquid medium, so the blood is the worst enemy, given the difficulty in viewing the structures. Because of this, continuous ventricular irrigation with warmed saline through a channel of the endoscope is mandatory. Endoscope-controlled microneurosurgery basically consists of the resection of hypophyseal tumors, skull base tumors, and tumors in other cranial locations done exclusively with the endoscope. Endoscope-assisted microneurosurgery consists of the use of the neuroendoscope alongside the surgical microscope, in the same operation, whether for the removal of tumors or in vascular surgeries [38–40]. The general indications for endoscopic neurosurgery are obstructions in cerebrospinal fluid (CSF) circulation pathways, arachnoid and intraparenchymal cysts, and intraventricular lesions [41–44]. The most common techniques for restoring CSF movement include: endoscopic third ventriculostomy (ETV), septostomy, foraminoplasty, aqueductoplasty with or without a stent, cyst fenestrations in general, and tumor removals [45–51]. The most common cranial point for ventricular neuroendoscopy is Kocher’s point, located in the frontal region, about 2 cm anterior to the coronal suture and 2 cm lateral to the midline. All the images for this atlas were obtained from this point, with small variations, in centimeters, according to the presence or absence of a patent fontanelle (in children), the ventricular anatomy, and the type of disease being treated. Other points of ventricular access are described both for endoscopic procedures themselves, and for ventricular punctures in general [52] (Figs. 2.13, 2.14, and 2.15). In adults and older children, the skull opening is done through a burr hole, and in newborns, the fontanelle can be used as a natural portal for the introduction of the endoscope (Figs. 2.16, 2.17, 2.18, 2.19 and 2.20). In newborns, the approach can also be performed by a minicraniotomy [53] (Fig. 2.21). Before the beginning of the surgery itself it is very important to check the position of the monitor for good visualization and for the comfort of the surgeon, mainly in more time-consuming procedures (Figs. 2.22 and 2.23). In adults and older children the opening of the skull can be performed with a high-rotation drill followed by a cross fashion dural opening (Figs. 2.24 and 2.25). In newborns the fontanelle is a physiological way and the dura is opened in linear fashion, allowing watertight closure at the end (Figs. 2.26 and 2.27). In endoscopic neurosurgery, the endoscope itself is the only ventricular visualization tool, and the tools are introduced into the surgical field through working channels that are part of each system. Currently several types of neuroendoscopes are available. Compact and small-size systems, such as the Oi HandyPro and Gaab systems (Karl Storz, Tuttlingen, Germany) allow more free movements inside the ventricle, as well as providing excellent image quality (Figs. 2.28 and 2.29). The endoscope can be inserted into the ventricular system through a trocar inside a sheath (Fig. 2.30), but some surgeons prefer a peel-away sheath. After the ventricular puncture is done, the trocar is removed, and in its place the optic is inserted, which is nothing more than the endoscope itself. At this point, it is possible to navigate the ventricle by maneuvering the optic with the dominant hand and holding the sheath at its entry into the skull with the non-dominant hand, in order to avoid inadvertent introduction of the system into the ventricle (Fig. 2.31). Some neuroendoscopists prefer to make use of an articulated arm in order to keep the endoscope in place and have both hands free to manipulate instruments through the working channels. Another alternative is that the neuroendoscopist can perform the navigation with both hands, as described above, and a second neuroendoscopist can manipulate the instruments through the working channel without concern for the navigation [54]. In this case, some systems allow the use of instruments in two work channels simultaneously, resulting in a bimanual dissection, although with coaxial movement, and obviously not as elegant as a microsurgical dissection [55]. A third alternative, which is the preference of this author, is a frameless free-hand technique [56], wherein the ventricular navigation through the endoscope is performed with the non-dominant hand and instruments are used through the working channel with the dominant hand. Among the various types of rigid endoscopy systems applicable to ventricular procedures, the first in which this technique has been described is the Oi HandyPro [56, 57] (Fig. 2.32). The Gaab system, although it was not designed specifically for this technique, allows the use of the free-hand technique due to its compact size (Figs. 2.33, 2.34, and 2.35). All images in this book were obtained with these two systems. It is possible for the neuroendoscopist to perform the procedures in a sitting or standing position, depending on the duration of the surgery (Figs. 2.36 and 2.37). At the end of the procedure, in adults and older children, biological glue is sufficient to seal the corticotomy, and closure of the scalp with planes is sufficient. On the other hand, in newborns, dural watertight closure (polypropylene or silk) and biological glue is mandatory (Figs. 2.38, 2.39, 2.40, and 2.41). When performing minicraniotomy [53], dural closure is also necessary, as well as bone fixation (Fig. 2.42). The scalp is closed layer by layer, in watertight fashion. In adults, the skin is closed with mononylon in separate points (Fig. 2.43), with the same skin closure used for children, although in babies an absorbable suture can be a good option for the skin. The ability to capture and archive neuroendoscopic videos, images, and patient data easily and securely during procedures ensures that detailed and accurate records of the diagnosis and treatment are achieved. These records also provide a valuable knowledge base for training, education, publication, and sharing scientific information with colleagues. The AIDA (Advanced Image and Data Acquisition) system (Karl Storz, Tuttlingen, Germany) offers a solution for the recording of patient data and full high-definition (HD) videos and still images. An intuitive interface and user-friendly front panel supports fast and uncomplicated handling (Fig. 2.44).

Fig. 2.12
Comparison of microscopic view (a) and non-angled (b) and angled (c) endoscopic views (Reprinted from Schroeder [38], with permission)

Fig. 2.13
Schematic illustration of all the extra-calvarial ventricular access points: Keen (1), Kocher (2), Dandy (3), Frazier (4), Kaufman (5), and Tubbs (6) (Reprinted from Mortazavi et al. [52], with permission)

Fig. 2.14
Kocher’s point in an adult

Fig. 2.15
Kocher’s point in a 2-year-old child

Fig. 2.16
Three-dimensional (3D) computerized tomography (CT) scan depicting the burr hole at Kocher’s point in an adult

Fig. 2.17
3D CT scan depicting the burr hole more anterior to the traditional reference of Kocher’s point, in a 2-year-old child with reduced fontanelle

Fig. 2.18
Detail of the transfontanelle approach

Fig. 2.19
Detail of the transfontanelle approach in a patient with complex hydrocephalus, after several external ventricular drainages

Fig. 2.20
3D CT scan showing detail of the postoperative linear incision in the transfontanelle approach

Fig. 2.21
Approach by minicraniotomy (Reprinted from Costa Val [53], with permission)

Fig. 2.22
Position of the neuroendoscopy tower

Fig. 2.23
Position of the neuroendoscopy tower and ready surgical field. Because of the continuous irrigation a waterproof bag is very useful

Fig. 2.24
Skull opening with a high-rotation drill in an adult

Fig. 2.25
Bipolar coagulation before the dural opening, performed in cross fashion, in an adult

Fig. 2.26
Dural opening performed in a linear fashion in a newborn

Fig. 2.27
Corticotomy with bipolar coagulation avoiding the dural edge to allow closure after the procedure

Fig. 2.28
Oi HandyPro system, with removable handle, including operating sheath handle (three work channels), mandrel, and wide-angle straightforward telescope 0° (enlarged view, diameter 2 mm, length 26 cm). Instruments (diameter 1.3 mm, working length 30 cm), including scissors (single-action jaws), biopsy forceps (double-action jaws), grasping forceps (double-action jaws), unipolar coagulation electrode, and bipolar coagulation electrode (Images courtesy of Karl Storz, Tuttlingen, Germany)

Fig. 2.29
Gaab system, including wide-angle straightforward telescope 6°, with working channel diameter 3 mm, length 15 cm. Operating sheath, graduated, outer diameter 6.5 mm, working length 13 cm, with lateral stopcock and catheter port and obturator. Instruments, including grasping forceps (single-action jaws, diameter 2.7 mm, working length 30 cm), biopsy forceps (single-action jaws, diameter 2.7 mm, working length 30 cm), scissors (pointed, single-action jaws, diameter 2.7 mm, working length 30 cm), scissors (pointed, slightly curved, double-action jaws, diameter 1.7 mm, working length 30 cm), biopsy forceps (double-action jaws, diameter 1.7 mm, working length 30 cm), forceps for ventriculostomy (diameter 1.7 mm, working length 30 cm), bipolar forceps (with flat jaws, size 2.4 mm, working length 24 cm), bipolar coagulation electrode (diameter 1.7 mm, working length 30 cm), unipolar coagulation electrode (semiflexible, diameter 1.7 mm, working length 30 cm), suction catheter (flexible, for single use, diameter 2.5 mm, working length 45 cm), irrigation tube, and holding system (Images courtesy of Karl Storz, Tuttlingen, Germany)

Fig. 2.30
Ventricular puncture under irrigation with Gaab system operation sheath. During the procedure it is very important to maintain continuous irrigation to avoid ventricular collapse. It is mandatory to have the passive exit always open to avoid intraventricular hypertension

Fig. 2.31
Ventricular navigation and inspection with the Gaab system

Fig. 2.32
Oi HandyPro frameless free-hand system

Fig. 2.33
Free-hand technique with the Gaab system, holding the neuroendoscope with the non-dominant hand and handling the instrument (grasping forceps) with the dominant hand

Fig. 2.34
Free-hand technique with the Gaab system, holding the neuroendoscope with the non-dominant hand and handling the instrument (scissors) with the dominant hand

Fig. 2.35
Free-hand technique with the Gaab system, holding the neuroendoscope with the non-dominant hand and handling the instrument (bipolar coagulation electrode) with the dominant hand

Fig. 2.36
Performing the surgery in the sitting position

Fig. 2.37
Performing the surgery in the standing position

Fig. 2.38
Aspect of dural opening at the end of the procedure. There is evident brain shift

Fig. 2.39
Polypropylene watertight dural closure

Fig. 2.40
Silk watertight dural closure

Fig. 2.41
Biological glue over suture

Fig. 2.42
After a minicraniotomy, the osteoplastic flap returns to its place, attached with the pericranium aid, after watertight dural closure (Reprinted from Costa Val [53], with permission)

Fig. 2.43
Mononylon separate point skin closure

Fig. 2.44
AIDA (Advanced Image and Data Acquisition) system. A solution for the recording of patient data, full high-definition (HD) videos, and images (Image courtesy of Karl Storz, Tuttlingen, Germany)
2.3 Main Ventricular Procedures and their Indications
2.3.1 Endoscopic Third Ventriculostomy (ETV)
ETV is the most often performed endoscopic procedure, and therefore it is the one most studied in the neuroendoscopic literature worldwide. The technique is based on the creation of an alternative exit route for draining CSF from the ventricular system into the subarachnoid space. In this technique a fenestration is performed in the third ventricle floor and in the membrane of Liliequist just below it, allowing the CSF to flow out to the interpeduncular and prepontine cisterns. The main indication for ETV is for obstructive hydrocephalus, and such obstruction may be located anywhere from the middle and posterior portion of the third ventricle to the median and lateral openings of the fourth ventricle. Moreover, patency of the subarachnoid space and preservation of CSF absorption at the arachnoid granulations level are necessary conditions. Magnetic resonance imaging (MRI) is the gold standard for the indication of this procedure, because it allows the topographic identification of the obstruction. The sagittal T2-weighted sequence is the most suitable for such analysis. On the other hand, there is no additional examination able to accurately assess the cisternal circulation and CSF absorption. Therefore, if, in association with the intraventricular obstruction mechanism, there is some pathological condition interfering with CSF circulation in the subarachnoid space or CSF absorption, the successful outcome of ETV can be questioned [48]. Other relevant information provided by MRI is the space below the third ventricle floor, particularly the gap between the clivus and the basilar artery in the interpeduncular cistern (Figs. 2.45 and 2.46). This data allows the third ventricle floor to be opened safely [58]. The lack of space is not an absolute contraindication to carrying out fenestration, but doing so requires a learning curve. The ETV technique involves inserting the endoscope through the frontal approach at Kocher’s point, typically situated 2 cm in front of the coronal suture and 2 cm lateral to the midline. This is not an absolute point, because small variations can be made, especially in the pediatric age group, taking into account the position of the fontanelle, which is a physiological portal, and ventricular size, always avoiding penetration in eloquent areas [59]. The right side is usually chosen, but the side on which the frontal horn is larger may be used. Penetrating the lateral ventricle, access to the third ventricle is achieved through the foramen of Monro. Soon after, the structures of the floor of the third ventricle can be identified; arranged in the anteroposterior direction, these are: optic chiasm, tuber cinereum with the infundibular recess, and the mammillary bodies. Fenestration in the tuber cinereum is done at the midpoint between the infundibulum and the mammillary bodies and may vary in some cases depending on the position of the bifurcation of the basilar artery, sometimes seen due to transparency through the tuber cinereum. This fenestration is usually performed with bipolar coagulation, or sometimes by using the tip of a non-activated bipolar electrode, serving as a blunt instrument. For such fenestration at an experimental level, a type of water jet has been conceived [60], and also laser can be an option [61]. After fenestration, the stoma is generally expanded by using a 3F or 4F Fogarty balloon catheter, or any other suitable instrument. Opening of the membrane of Liliequist, located just below the third ventricle floor, is of great importance. In cases of delayed closure, repeating ETV before considering a shunt implant is recommended [62]. After the fenestration, the endoscope can be inserted inside the interpeduncular cistern to expand the stoma and see if the subarachnoid space is patent. Technical details may vary between neuroendoscopists, from using a Fogarty catheter balloon, to grasping forceps, to using a double balloon catheter in the form of an eight, especially developed for ETV [63]. The overall success rate of the ETV procedure is around 75%, but this depends on the etiology of the hydrocephalus and, above all, on the age of the patient [64]. In adults with obstructive hydrocephalus, the chance of success is very high. Also, in normal-pressure hydrocephalus when there is an obstructive component present, the results are favorable [65]. In children, taking into consideration the etiology, the best results are seen in hydrocephalus secondary to aqueductal stenosis, and in tumors in the posterior portion of the third ventricle or in the posterior fossa. Spennato et al. reviewed the literature and found an average success rate of 68% in patients with aqueduct stenosis [66]. Sainte-Rose et al. carried out a comparative study of the performance of ETV in children with posterior fossa tumors. When the endoscopic procedure was performed before the approach to the tumor, the chance of long-term development of hydrocephalus was only 6.6% [67]. On the other hand, in cases in which external ventricular drainage or simple resection of the tumor was done in attempts to restore the CSF circulation, the rate of hydrocephalus requiring further treatment was 26.8%. Although this was not a prospective, randomized study, the authors concluded that ETV was superior to any other approach in the management of hydrocephalus secondary to posterior fossa tumors [67]. O’Brien et al. [68] analyzed 170 cases of primary pediatric ETV; that is, ETV used as the first treatment for hydrocephalus of different etiologies. These authors reported a success rate of around 70%. However, when they analyzed patients with hydrocephalus secondary to intraventricular hemorrhage or meningitis, the success rate was just 27% or 0%, respectively, once again confirming the significance of the obstructive etiology. These authors also analyzed 63 cases where ETV was performed in the presence of dysfunction (mechanical or infectious) of a ventriculoperitoneal shunt. The success rate was 78%, showing that although the shunt was present and there was possible lower CSF circulation in the subarachnoid space previously, these factors would not be a hindrance for an ETV operation [68]. Concerning etiology, pediatric ETV has a high failure rate in children with previous shunts and in those with postinfectious hydrocephalus [69]. If, on one hand, the etiology in children is well defined as a prognostic factor for the success of ETV, the age is also an important factor to be considered. Today it is known that the earlier the procedure is performed, the greater the chance of failure, due to the immaturity of the ventricular system and also due to the existence of alternative pathways for different CSF drainage compared with those in adults. It has been considered that age of less than 2 years would be a factor for poor prognosis. Later, studies emerged that advocated avoiding ETV alone in children under 1 year of age, which seems to be the most reasonable recommendation in the current literature [70]. In a study by Koch-Wiewrodt and Wagner [71], in a series of 28 children who underwent ETV before 1 year of age, when comparing the average age of those with a successful procedure with the age of those whose procedure failed, there was no statistically significant difference. However, when considering the chance of failure month by month, these authors noticed an increasing trend in the success rate after age 2–4 months. This observation was also noted in patients with aqueduct stenosis in the cases found in the literature of the time [71]. Other small series are in favor of ETV at any age [72, 73]. Given this controversy, there is now the possibility of predicting the success of ETV in pediatric patients using a scale, the ETV Success Score (ETVSS). The scale takes into account the age, etiology, and the presence or absence of a previous shunt, and the points assigned to each parameter are summed. The higher the sum, the greater the chance of success [74, 75]. Another way to predict success in pediatric patients is through artificial neural networks (ANN) [76]. An association between ETV and choroid plexus coagulation seems to be a promising technique for patients under 1 year of age, as discussed in Sect. 2.3.2 (Choroid Plexus Coagulation [CPC]). Like any surgical procedure, ETV is not without complications. A literature review shows the overall complication rate is between 5% and 15%, the permanent morbidity is less than 3%, and mortality is less than 1% [77]. Of course, when analyzing the number of complications in the literature, one should take into account the limitations and the learning curve of each individual neurosurgeon. To assess patient outcomes, apart from clinical evolution, postoperative imaging such as CT or MRI is important to evaluate the success of the ETV (Figs. 2.47, 2.48, 2.49, 2.50, and 2.51).

Full access? Get Clinical Tree
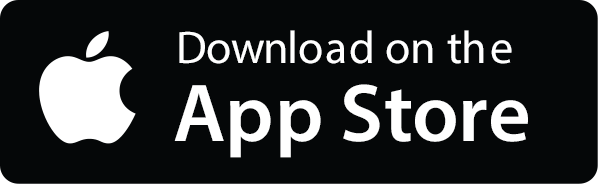
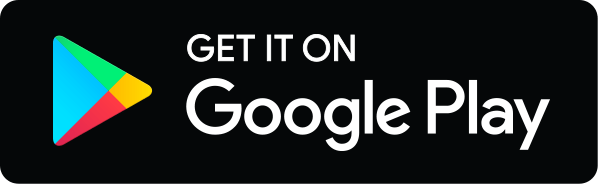