Background
Despite trimodal therapy consisting of maximal safe resection and adjuvant partial radiotherapy with concurrent and subsequent temozolomide, glioblastoma (GBM) has a dismal prognosis, with a median survival of 14.6 months and an overall survival of only 9.8% at 5 years.
Further contributing to therapeutic challenge is the intertumoral and intratumoral heterogeneity of GBM. The strongest predictor for response to temozolomide according to Stupp and colleagues was methylation of the MGMT (O6-alkylguanine DNA alkyltransferase) promotor. However, intratumoral heterogeneity in MGMT methylation status has been noted, perhaps explaining the positive response to temozolomide in some patients with unmethylated GBMs. Furthermore, a small population of glioma cancer cells, postulated to be stemlike cells that are able to self-renew and produce diverse daughter cells, have been shown to be chemoresistant and radioresistant, leading to inevitable tumor recurrence.
In this context, there has been considerable interest in an alternate approach to cancer therapy to further prolong survival and perhaps even offer a cure, namely by harnessing the unique specificity of the immune system. The central nervous system (CNS) has classically been considered immunologically less active, based on early experiments showing prolonged survival of skin grafts within the CNS compared with other sites. This notion has historically been furthered by reports of isolation from the immune system imposed by the blood-brain barrier (BBB), absence of CNS lymphatic drainage, and immune incompetence of native antigen-presenting cells (APCs). In contrast, active immune responses in the CNS are common clinical entities, such as in multiple sclerosis or Alzheimer disease.
Recent developments argue against the dogma of the CNS as an immunologically isolated site. The BBB is disrupted by tissue injury and inflammation, notably in the setting of malignant gliomas, allowing entry of immune cells into the CNS. Even in the absence of BBB disruption, peripheral immune cells can circumvent the BBB using trafficking signals. Moreover, CNS T cells and antigens drain into cervical lymphatics via the subarachnoid space, along the olfactory nerve, and across the cribriform plate. In addition, the resident macrophages of the CNS, microglia, can express major histocompatibility complex (MHC) class II antigens and induce differentiation of naive T cells.
The association between infection and remission of cancer (the infection-remission coincidence) has been noted in the literature for more than a hundred years. More recently, a retrospective, single-center, cohort study has reported a 2-fold survival benefit in patients with GBM who developed postoperative bacterial infections. In addition, an inverse relationship between atopic disease and gliomas has also been observed in numerous reports (as reviewed in Ref. ). The US Food and Drug Administration (FDA) approval of the antigen-specific agent sipuleucel-T for prostate cancer in 2010, and an immune checkpoint inhibitor ipilimumab for metastatic melanoma in 2011, among others, has led to the emergence of cancer immunotherapy into the clinical mainstream. These developments, in addition to the dismal prognosis of patients with GBM with standard therapy, have sparked interest in the potential of harnessed immunotherapy for brain tumors.
Cancer immunotherapy can be broadly defined as therapy based on the immune system’s ability to target and kill tumor cells. It can be classified by mechanism into immunomodulatory, passive, and active immunotherapy. Immunomodulatory therapy involves administration of interleukins, cytokines, and chemokines to enhance the antitumor activity of native effector cells. A phase III trial in recurrent GBMs is currently underway that involves inactivation of checkpoint mediators CTLA-4 (cytotoxic T-lymphocyte-associated protein 4) and PD-1 (programmed cell death protein 1). Passive immunotherapy historically refers to administration of antibodies to target tumor antigens (eg, antibodies against the HER2 [human epidermal growth factor receptor 2]/neu receptor in breast cancer), and is currently undergoing resurgence in the form of transfer of ex vivo expanded effectors cells. In addition, active immunotherapy depends on activation of the patient’s immune system with so-called tumor vaccines, a term that encompasses a variety of products. Examples of phase III trials of active immunotherapy in GBM include one based on dendritic cells (DCs) ( NCT00045968 ), and another based on peptides ( NCT01480479 ), the latter of which has recently been discontinued.
Peptide targeting
Considerable effort has been invested in the peptide vaccine rindopepimut (also called cdx-110) targeting the tumor antigen epidermal growth factor receptor variant III (EGFRvIII). Rindopepimut is a peptide that spans the mutation site of EGFRvIII, conjugated to the immunogenic carrier protein keyhole limpet hemocyanin. Peptide vaccines have the advantage of being simple to manufacture compared with vaccines that require harvesting tumor or serum samples from patients. Three phase II trials of rindopepimut conducted on patients with newly diagnosed, EGFRvIII-positive GBM have shown promising results, with reports of overall survival of 24 to 26 months ( Fig. 19.1 ). Most tumors showing progression no longer expressed EGFRvIII. According to the immunoediting hypothesis, this finding suggests that rindopepimut was effective in eradicating its target cell population via immunologic pressure. A phase II trial in recurrent GBM showed a survival advantage of 11.6 versus 9.3 months with the addition of rindopepimut to bevacizumab. In spite of these early successes, a phase III trial of rindopepimut for newly diagnosed GBM has recently been discontinued with an interim analysis showing equivalent overall survival in the treatment group (20.4 months) versus control (21.1 months). These results emphasize that further work is needed to refine the peptide vaccine approach for EGFRvIII.

EGFRvIII is expressed on the cell surfaces in approximately 30% of all human GBMs, representing the most common epidermal growth factor receptor (EGFR) mutation. More recently, this has been confirmed by custom next-generation sequencing–based assay on RNA from tissue specimens. EGFRvIII is known to enhance tumor invasiveness, cell motility, and chemoradioresistance, and is independently associated with a poorer prognosis. Because this mutation promotes tumorigenesis, it likely represents a driver mutation unique to cancer cells, and indeed it has shown specificity for tumor cells. Moreover, EGFRvIII has also been shown to be expressed in a subpopulation of GBM cells that share properties of cancer stem cells.
Peptides have also been targeted through the use of tumor-derived heat shock proteins (HSP). HSPs function in antigen carriage and in the targeting and activation of APCs, including DCs, which then leads to priming of the immune system’s effector cells. This family of proteins is upregulated by cellular stressors; for example, heat, hypoxia, infection, and malignant transformation. Only HSPs derived from tumor cells, and when complexed to tumor antigens, are capable of inducing antitumor immunity. Hence, HSP vaccines require HSPs complexed to antigen peptides (HSP-protein complexes), procured from the patient’s tumor.
Most HSP vaccine trials have been based on prophages (also called HSP-96 protein complex), using a tumor-lysate approach. HSP-96 has notable substrates implicated in tumorigenesis, including EGFRvIII, platelet-derived growth factor receptor (PDGFR), FAK, AKT, p53, and phosphatidylinositol 3 kinase (PI3K). In a phase II trial for recurrent GBM, median overall survival was 90.2% at 6 months, and 29.3% at 12 months. Another trial in newly diagnosed GBMs showed a median overall survival of 23.8 months. A phase II trial of prophages in combination with bevacizumab for recurrent GBMs is currently underway ( NCT01814813 ).
Viral vaccines
Tumor vaccines based on viruses have also been widely studied, in which viruses are used as vectors for either in vitro or in vivo gene transfer, with the goal of expressing a protein that kills cells directly (so-called suicide gene therapy) or activating the immune system to kill the target cells ( Fig. 19.2 ). Viruses are particularly effective vectors because they are often tropic to certain cells. The most investigated suicide gene is that encoding type I herpes simplex virus thymidine kinase (HSV1-TK), which monophosphorylates the antiviral drug ganciclovir. The monophosphorylated ganciclovir is then converted by the host cell into triphosphate ganciclovir, and is subsequently used in DNA synthesis. Triphosphate ganciclovir blocks chain elongation, halting cell division.

Initially, retroviral vectors were used to transduce the HSV1-TK system into brain tumors. Because retroviral RNA must be converted into DNA before replication in the host cell, this vector was specific to proliferating cells actively synthesizing DNA. However, because most tumor cells are mitotically arrested, most cells were not transduced by retroviruses during HSV1-TK/ganciclovir therapy. Subsequent studies used adenoviral vectors, which allow transfer of genes into both dividing and nondividing cells, but this enhanced efficacy came at the expense of tumor specificity, and hence safety.
Oncolytic viral therapy
Oncolytic viral therapy enables tumor-selective conditional viral replication, leading to local self-amplification in which lytic cell destruction and release of viral progeny maintain propagation of viral attacks throughout the tumor. The herpes simplex virus (HSV) shows natural neurotropism, and is clinically known to cause necrotizing encephalitis. Genetically engineered HSV, in which the machinery for DNA viral replication has been attenuated, allowed the conditional replication of viruses selectively in dividing cells.
Adenoviruses have also been used in oncolytic therapy. Adenoviral gene products of E1A and E1B normally interact with host cellular control, allowing viral replication in host cells. Proteins from the E1A gene trigger cells to enter the S phase by inhibiting cellular retinoblastoma (pRb); E1B-encoded proteins suppress apoptosis by inactivating cellular p53.
Conditional replication in tumor cells has been made possible by deletion of these genes, as these tumor suppressor proteins are often dysregulated in malignant gliomas. The E1B-deleted adenovirus (ONYX-015) has been studied in a phase I trial. Although there were no significant toxicities, it did not have therapeutic efficacy. Notably, the ONYX-015 trial involved peritumoral injection of the oncolytic virus into brain tissue following fresh resection. The E2B-deleted adenovirus (Delta-24) has shown tumor selectivity, and has been further engineered to target tumor-associated receptors (eg, integrins) on the surface of cancer cells (Delta-24-RGD; also called DNX-2401). A phase I study of intratumoral and peritumoral injections of Delta-24-RGD for recurrent GBMs is currently underway.
Polioviruses, clinically known to target motor neurons that can lead to poliomyelitis, show natural tropism to CE155 receptors overexpressed on tumor cell surfaces, and have shown lytic growth in glioma cells. Neurotoxicity of the poliovirus is mediated by its internal ribosome entry gene site. An attenuated polio recombinant was engineered by replacing this element with its counterpart from the rhinovirus. A phase I trial is currently underway for recurrent GBMs, using a single intratumoral convection-enhanced delivery of the engineered poliovirus before tumor resection.
Oncolytic viral therapy has the potential to combine multiple modes of action, including infection of endothelial cells leading to vascular collapse, direct viral destruction of infected tumor cells, and induction of innate and adaptive immune responses leading to destruction of both infected and neighboring tumor cells.
Dendritic cell vaccines
A widely studied form of vaccine therapy is based on DCs. In contrast with tumor cells, which are poor APCs, DCs are considered professional APCs. Administration of tumor antigen–loaded DCs therefore represents an attractive strategy to generate tumor-specific effector cells. The first DC vaccine introduced was sipuleucel-T for prostate cancer, which was approved by the FDA in 2010. DC vaccines are developed from the patient’s peripheral blood monocytes, which are differentiated into DCs in vitro, then pulsed with antigens ( Fig. 19.3 ). Note that DC vaccines rely on endogenous T cells, which may limit its treatment effect because of the immunosuppressive nature of GBMs.

DC vaccines have been developed targeting EGFRvIII. In a phase I trial for newly diagnosed GBMs, immune response was confirmed with proliferation of antigen-specific T cells in vitro, and with delayed-type hypersensitivity responses in vivo. There were no significant adverse effects.
Polyvalent DC vaccines, in which numerous tumor antigen epitopes are targeted, have also been reported to be safe in numerous studies, in spite of the theoretically increased risk of selection for autoreactive T cells. The ICT-107 vaccine was developed with autologous DCs pulsed with 6 tumor antigens overexpressed specifically in the cancer stem cell population (MAGE-1 [melanoma antigen-1], HER-2 [human epidermal growth factor receptor 2], AIM-2 [immunoselected melanoma-2], TRP-2 [tyrosinase-related protein-2], gp100 [glycoprotein 100], interleukin [IL]-13Rα2). Median overall survival was promising at 38.4 months. A randomized phase II trial is currently under analysis.
In another polyvalent DC vaccine, DC-Vax-L, DCs were pulsed with tumor lysates prepared from resection specimens. Adverse effects were likewise not significant. Median overall survival rates in patients with either newly diagnosed or recurrent GBMs were also favorable relative to historical controls, at 31.4 months since the date of initial surgical diagnosis. A phase III trial of DC-Vax-L is currently underway.
Adoptive cellular therapy and chimeric antigen receptor T cells
More recently there has been much interest in adoptive cellular therapy (ACT), which entails the administration of autologous or allogeneic antitumor lymphocytes. It is typically preceded by lymphodepletion in order to eliminate T-regulatory cells and native lymphocytes that compete for homeostatic cytokines, thereby providing an optimal environment for the transferred cells. Lymphocytes with the desired antitumor properties are selected and expanded ex vivo, and then infused into patients ( Fig. 19.4 ).

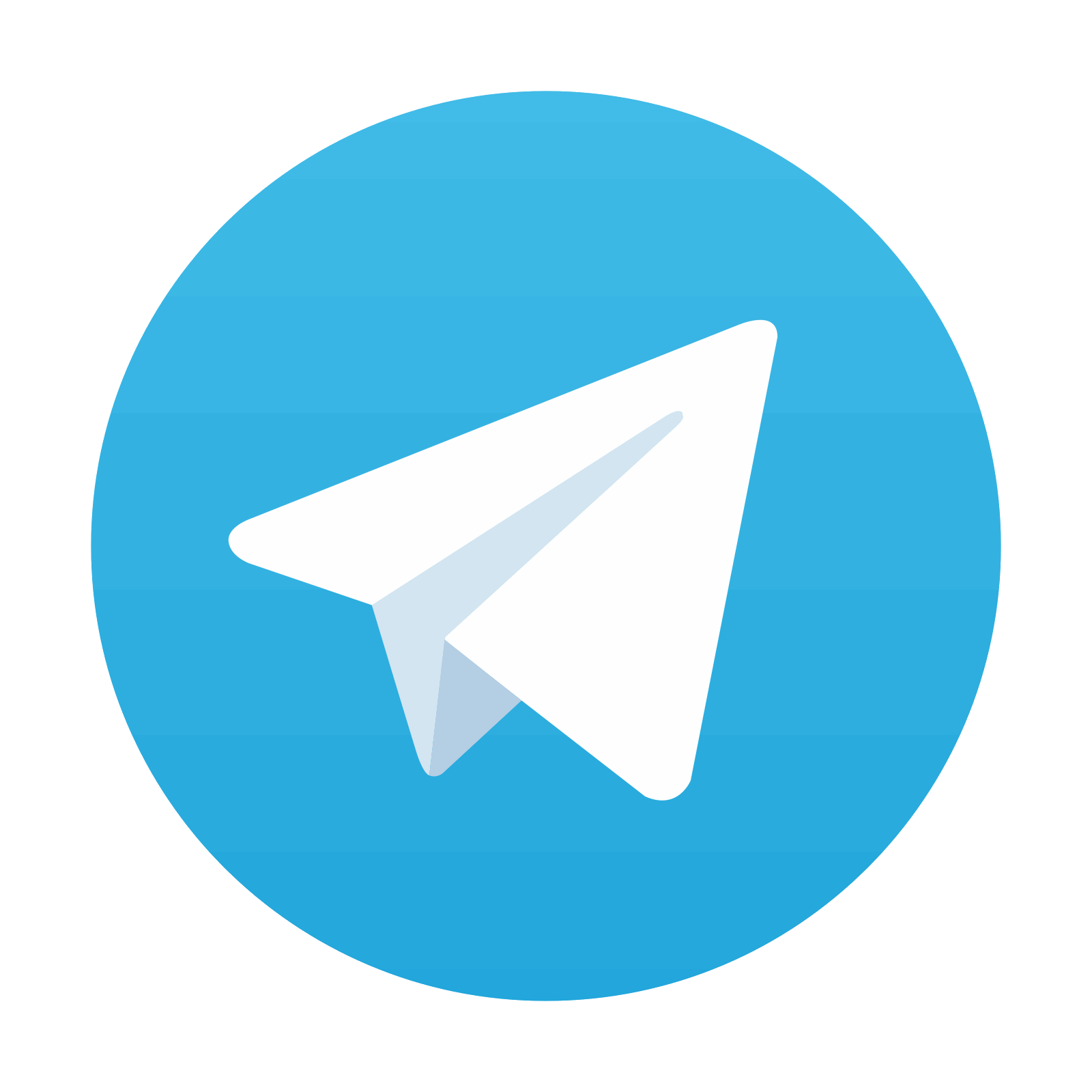
Stay updated, free articles. Join our Telegram channel

Full access? Get Clinical Tree
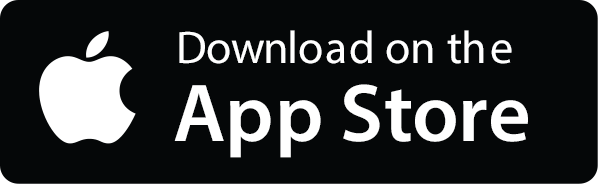
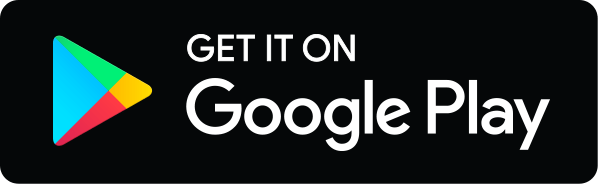
