INTRODUCTION: LIVING LONGER VERSUS CHEATING DEATH
That there may be specific biological processes determining life span is an intriguing concept, and one which has attracted interest from both the academic and commercial sectors. Such interest has often focused on the simple numerical indicator of life span but this can often be at odds with the clinical perspective that centres on quality of life for the elderly. In this chapter we will review the latest findings and current ideas in the fields studying the biological process of ageing, its evolutionary underpinning and the genetic factors intertwined with environmental exposure that determine our life span. There is also a semantic issue of whether a discussion of ageing should include factors contributing to longevity if they merely afford protection against the dominant degenerative disorders or serious illnesses of the elderly or only concentrate on mechanisms that actively delay the ageing process1. Plainly, these two aspects of ageing are inextricably linked but from a research point of view, and in the context of genetic models, only the latter offers fundamental insights into the process.
AGEING GENETICS, HERITABILITY AND EVOLUTIONARY INFLUENCES
Danish epidemiological studies have reported that the heritability of human longevity is approximately 25%2,3: a figure similar to that for lower organisms. Thus there exists scope for the genetic dissection of the phenotype and a substrate for the processes of evolution to act on4. In its purest form, evolution requires that advantageous genetic variation is favoured and increases its frequency in the population through the process of natural selection. This is mediated through its ability to increase a carrier individual organism’s ‘fitness’ and thus its ability to reproduce at a greater than average level. It can be appreciated that this raises fundamental conceptual issues in the context of the genetics of longevity. Female reproductive fitness, in particular, occupies only a proportion of the modern human life span and so it could be hypothesized that gene variants influencing life span in the age range from 55-85 years would be under minimal selection compared to those dictating survival within the 15-45-year age range. It has also been argued that, for some species, individuals living beyond reproductive age are counterproductive to species survival – they are a drain on limited resources and thus compete with actively reproducing individuals. Selection pressure for a biologically programmed ‘cap’ on life span might avoid this conflict. A second argument against a clear genetic contribution to human longevity would be the vast period of human existence on Earth for which 45 years was ‘old age’. In the case of humans, long life spans are a modern phenomenon – average life expectancy dropped in the transition from ‘hunter-gatherer’ (Mesolithic Stone Age) to agrarian (Neolithic Stone Age) lifestyles5 and did not significantly exceed the median life span of approximately 30-35 years until recent centuries. The implications are that not only does a long life harm fellow members of one’s species, it also has no evolutionary outlet in the form of increased reproductive fitness. The genetics of ageing is left evolutionarily ‘rudderless’ in these circumstances.
However, two hypotheses offer an alternative view, supporting an evolutionarily ‘visible’ role for ageing. Firstly, the adoption of agriculture inevitably led to community living and increased social complexity. These social organization developments might be manifest in the altruistic role of the elderly in supporting the community – perhaps through leadership, childcare or other division of labour favourable for group survival. The impact of cultural effects (a form of environment) on ageing can never be entirely separated from genetics in the study of ageing in particular and will be covered in more detail below. Secondly, and perhaps more biologically tractable, is the argument that the genetic variants altering later life survival also play a role in earlier life fitness and are thus relevant to reproductive potential.
Finally, it is also worth considering the corollary of the sudden upturn in life span in recent human history – it has exposed humans to the consequences of genotypes that were never previously expressed for so long. For example, gene variants increasing susceptibility to Alzheimer’s disease might be the result of random mutational drift or positive selection for their benefit in the young, but it is only now that they have been exposed to the environment of the ageing brain and consequently deemed pathological.
AGEING BIOLOGY: CLUES FROM HUMAN SYNDROMES
The fact that well-characterized human disorders of premature ageing (progeria) exist suggests that there are real biological processes at work, and ones which are tractable to genetics research. Individuals with progeroid disorders present with a spectrum of phenotypes which closely resemble natural ageing but these occur in young adulthood or even childhood. A multitude of mutations in two particular genes, WRN and LMNA, account for the majority of these cases. Werner syndrome (WS) is perhaps the best studied of these and is caused by mutations in the WRN gene which encodes a heli-case/exonuclease protein that functions during the critical processes of active nuclear DNA functioning such as replication, recombination and repair – when potentially pathological errors can be introduced into our DNA. Thus, the consequences to genomic integrity in WS patients are an accelerated version of those hypothesized to occur in normal individuals as a result of a lifetime of environmental exposure and mutation accumulation. The phenotypes are similar too, ranging from osteoporosis, cataracts, type II diabetes, atherosclerosis and cancers (particularly mesenchymal sarcomas) such that WS patients tend to die in their 50s as a result of complications arising from these conditions. Interestingly, the phenotypic spectrum of WS doesn’t seem to include the neurodegenerative conditions such as Alzheimer’s disease. It is tempting to speculate that these particular disorders are due to the accumulation of protein aggregates (plaques and tangles), i.e., protein attrition rather than genomic DNA attrition.
Mutations in the lamin A gene, LMNA, encoding a nuclear intermediate filament protein involved in the packaging of nuclear chromatin, cause ‘atypical’ WS, or in extreme cases involving a particular LMNA splice mutation, the severe and very early-onset Hutchinson-Gilford progeria syndrome (HGPS). A number of other rare progeroid syndromes exist and, of these, Cockayne syndrome type 1 (CS) is the only other one discussed here. This is caused by mutations in the transcription-coupled nucleotide excision repair genes, CSA and CSB, which also contribute to the cell’s battle against genomic damage.
The genes emerging from these human conditions unequivocally point to the maintenance of genomic integrity as a vital process in ageing, involving the concerted action of several different systems to achieve it. Mutations in these systems seem to result in either progerias or cancer syndromes, or occasionally both (e.g. WS). It has been suggested that these alternative outcomes result from the specific responses to DNA damage initiated by the various systems: repair, apoptosis or senescence6.
AGEING BIOLOGY: CLUES FROM LOWER ORGANISMS
The validity of certain mouse models of ageing has sometimes been questioned for the very reasons discussed previously – distinguishing genuine progeroid features from a numerically shorter life span due to pathological state7. Therefore, the presence of features such as kyphosis, as an indicator of an osteoporotic state, stereotypical fat deposition and atrophy of the epidermis are often employed as benchmarks of true ageing models. The progeroid conditions in humans point towards accumulating DNA damage as a mechanism of ageing and the increased life span of mice over-expressing the antioxidizing protein thioredoxin seems to confirm the importance of this process. However, other transgenic mouse models with modulated life spans suggest that additional contributory mechanisms are at play. Historically, it has been suggested that cellular, organ and organismal ageing are affected by the rate of cell proliferation and apoptosis. This has manifest itself in such concepts as the Hayflick limit8
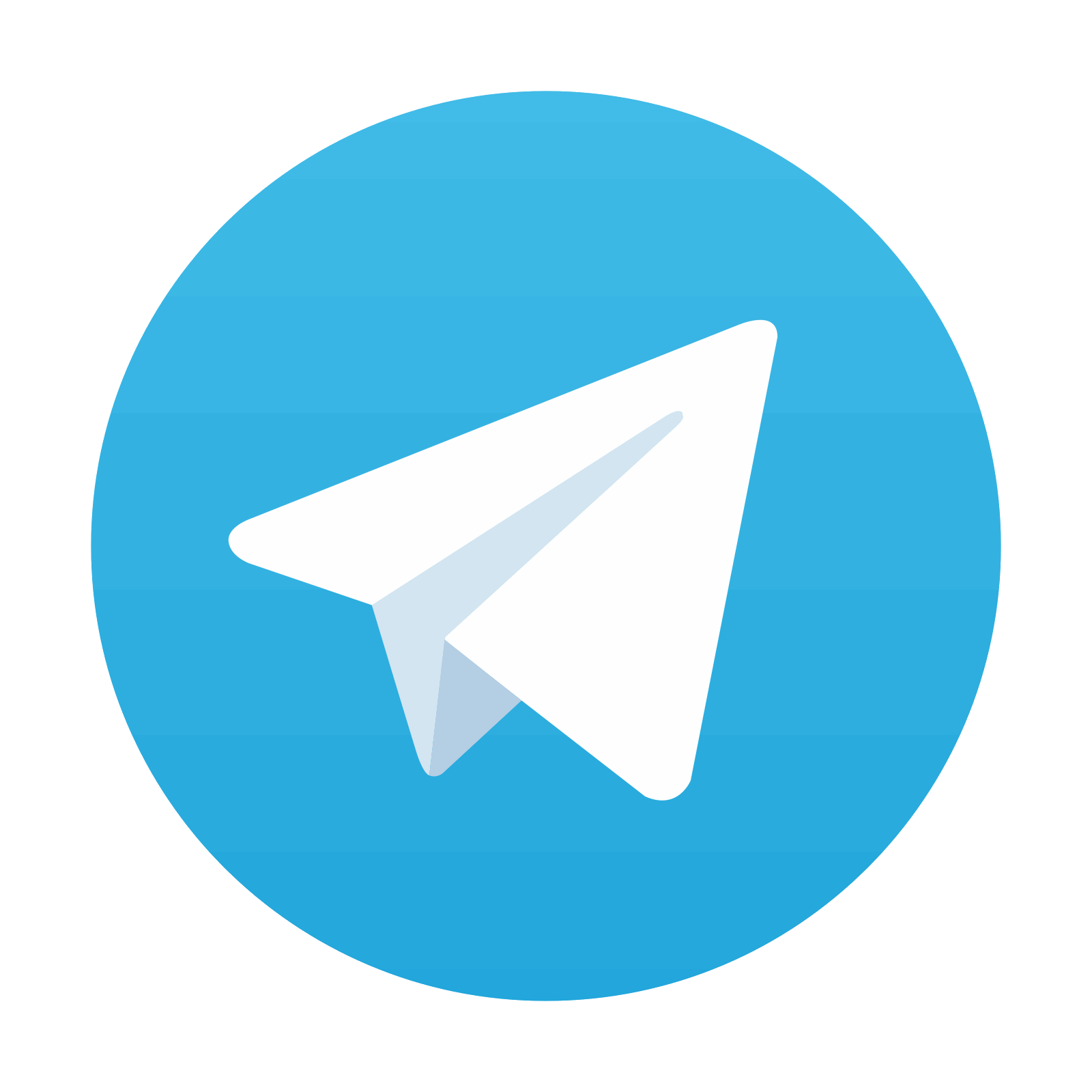
Stay updated, free articles. Join our Telegram channel

Full access? Get Clinical Tree
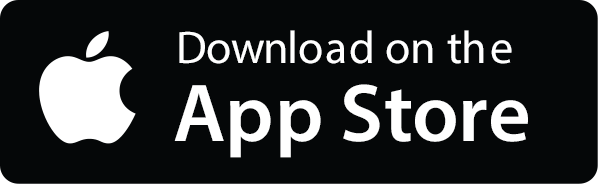
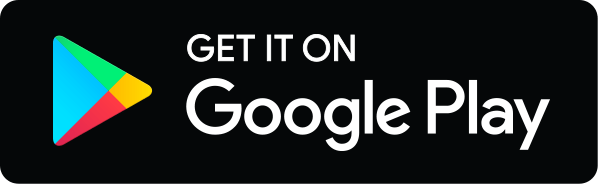