Mutations Without Loss of Gene Function
Another technique involves the use of “knock-in” mice. Unlike knockout mice, these mouse models are used to address more specific and detailed functions of the target gene in the presence of the gene’s function. That is, in knock-in mice the target gene is replaced with a mutated but functional form to determine the effects of subtle changes in protein structure or function (21).
Recently, many laboratories have developed a variety of methods to affect gene expression in mice using RNA interference (RNAi). In the RNAi process, small double-strand RNAs are used to specifically bind to and promote the degradation of target mRNA (22, 23). This leads to a decrease of synthesis of the target protein to 10–40% of its normal level but does not eliminate expression of the target protein entirely (24). Manipulating RNAi is complementary to studying gene function, especially when gene knockouts are not useful because they result in embryonic or early postnatal death. There are also other transgenic techniques that allow for the modification of genes in such a way that they act only at specific times or are expressed only in specific tissues or cell types (25).
Polygenic Models
In quantitative trait locus (QTL) studies, investigators screen the genome to locate regions contributing to variance (Box 5-1) in obesity traits. In the process, statistical association is assessed between traits being studied and genetic markers—segments of DNA with an identifiable physical location on a chromosome and for which inheritance can be followed. Such an association suggests that a gene in the area of the marker plays a role in the development of the disease. These studies provide an indirect way of determining the approximate location and inheritance pattern of genes that are near the genetic marker and affect a given disease or phenotype. Several species of animals have been used in QTL studies, including chickens (26), pigs (27), rats (28), fruit flies (29), bats (30), seals (31), rhesus monkeys (32, 33), hamsters (34), and mice (35).
Box 5-1 Glossary of Genetics Terms
Variance: a measure of statistical dispersion indicating how far values typically are from the distribution’s mean.
Phenotypic variance: the total variance among phenotypes over the range of environments.
Environmental or error variance: any non-genetic source of phenotypic variation among individuals.
Genetic variance: variation in a trait within populations due to genetic differences among individuals; includes additive variance, dominance variance, and epistatic variance.
Additive variance: variance of a quantitative trait due to the single effects of alleles at the loci that influence the trait.
Dominance variance: variance of a quantitative trait due to the interaction of the two alleles at a locus.
Epistatic variance: variance due to interaction among alleles at different loci.
Heritability: the proportion of population variance in a trait attributable to segregation of a gene or genes.
a) broad-sense heritability: the ratio of total genetic variance to total phenotypic variance.
b) narrow-sense heritability: the ratio of additive variance to the total phenotypic variance.
Population stratification: the existence of groups of individuals within a population that have some degree of reproductive isolation from the rest of the population and for which allele frequencies are likely to be different from the population as a whole.
Gene–gene interaction: when two or more DNA variations interact either directly (DNA–DNA or DNA–mRNA interactions), to change transcription or translation levels, or indirectly by way of their protein products, to alter disease risk separate from their independent effects.
Gene–environment interaction: when a DNA variation interacts with an environmental factor, such that their combined effect is distinct from their independent effects.
Phenocopy: the presence of a disease phenotype that has a non-genetic (random or environmental) basis.
Trait heterogeneity: when a trait has been defined with insufficient specificity such that it represents at least two distinct underlying traits.
Genetic heterogeneity: the production of the same or similar phenotypes (observed biochemical, physiological, and morphological characteristics of a person determined by his/her genotype) by different underlying genetic mechanisms.
a) Locus heterogeneity: different genetic loci leading to clinically similar phenotypes.
b) Allelic heterogeneity: different alleles at a single locus leading to clinically similar phenotypes.
Selective breeding: deliberate mating of individuals so that offspring will have a desired trait.
Human Studies
Whereas animal genetic studies are mainly experimental, human genetic studies are mainly observational, i.e., there is no manipulation of the genome or other key, putatively causal, factors. There are two general strategies used to study the role of genes in obesity phenotypes in humans (36). The first, the measured genotype approach, is based on direct measurement of genetic variation at the protein or DNA levels in an effort to assess the effect of differences in alleles on phenotypic differences. The second, the unmeasured genotype approach, attempts to use differences in disease expression, referred to as phenotypic variance, to estimate the contribution of genetic variance and to find quantitative evidence for single genes with detectable effects on the disease. The contribution of genes to the disease is studied by examining the phenotype or the extent to which the disease is manifested. In humans, several experimental designs, including family, twin, and adoption studies (36), as well as analytic genetic approaches, such as linkage analysis, heritability estimation, and others, have been used to study the genetics of obesity. Some are discussed below.
Designs of Human Studies
Family Studies
Family studies can address whether a disease aggregates in families (37). Such studies typically compare the prevalence of the disease among first-degree relatives of affected cases to the prevalence in the population or among relatives of unaffected controls. A higher risk among relatives of cases indicates that the disease may be familial, but it does not necessarily mean that genes are involved; a disease may run in families because of a shared environment. Thus, family studies cannot establish the role of genes or estimate the magnitude of their influence (37).
There are two types of “natural experiments” that enable us to estimate the extent to which complex traits are familial because of shared genes, shared environment, or both: twin studies and adoption studies (38–39).
Twin Studies
Twin studies compare the concordance of disease rates between identical/monozygotic (MZ) twins (who share identical sequences over the entire genome) and fraternal/dizygotic (DZ) twins (who share identical sequences over half of their genes on average) (40). If we assume that shared environment affects MZ twins and DZ twins to the same extent (the equal environments assumption), then significantly higher concordance in MZ than in DZ twins (i.e., disease rates are more similar in MZ twins) reflects the action of genes. If only one twin has the disease (concordance rate <100%), then environmental factors must be influencing disease expression (40).
The simplest method to analyze twin data for continuously distributed traits such as BMI is the mean absolute intrapair difference (41). For example, we might compute the mean difference of a trait, such as BMI, for pairs of monozygotic twins and for pairs of dizygotic twins. If variation in a trait is a result of genetic differences between individuals, then the mean difference in trait values between MZ twins should be less than that of the difference for DZ twins. However, mean intrapair differences and concordance comparisons determine only whether MZ twins are more likely than DZ twins to have the same disease. These analyses do not allow quantification of the heritability of the trait or estimation of the proportion of the variance that is due to genetic differences between individuals.
Adoption Studies
The assumption of adoption studies is that resemblance in the trait between the adoptee and members of the adoptee’s biological family is due to shared genes, whereas resemblance between the adoptee and members of the adoptive family is due to shared family environment (42). Adoption studies can help distinguish genetic and environmental influences on family resemblance by comparing rates of a disease in children to rates in their biological vs. adopted parents (37, 40). If genes influence the risk of a disorder, biological family members (genetically related) should resemble each other more than do adoptive family members (environmentally related).
Admixture Studies
It is well known that disease does not affect all populations equally. Assessing variation in the rates of disease according to demographic factors, such as sex and race or ethnicity, is a common component of epidemiologic research and the information gained in this way affects clinical and public health practice (43). In association mapping (which is similar to linkage analysis, but rather than relying on transmission of alleles within families, relies on correlating allele frequencies to disease status in large populations), variation in risk factors due to systematic ancestry differences between cases of a disease and controls without the disease, sometimes loosely termed population stratification, may cause confounding unless properly controlled. That is, failure to account for population stratification may lead to errors in inferring association between genetic variants and disease status.
Admixture occurs when two or more genetically diverse populations merge to form a new population. Localizing disease genes using an admixed population is called admixture mapping. In human admixture studies, researchers combine information about known population history with information from individuals’ measured genotypes to address questions such as: “Do persons who inherited more of their DNA from ancestors from such-and-such region of the world tend to have higher values of a trait (e.g., body weight) than do persons who inherited less of their DNA from that region of the world?” or “Are there particular regions of the genome at which inheriting DNA from ancestors from such-and-such region of the world predisposes one to particular diseases?”
Studies consistently show that obesity is more common in people of West African ancestry than in people of European ancestry (44, 45). The African American population is an admixed human population for whom about 20% of the genetic material traces to European ancestry (46). The association between increased obesity risk and African ancestry and the admixed nature of the African American population (46) suggests that admixture mapping (47) might be an important obesity gene-finding strategy to study genetically heterogeneous populations of African American descent.
Findings for the Genetic Component of Obesity
In the 1950s, weight loss induced via temporary dietary restriction in obese subjects was found to be short-lived (48). Furthermore, the metabolic state of these dieting individuals seemed to resemble that of a starving person in some ways. These observations led to the conjecture that there was a genetic component to obesity. Later studies on adoptees (49) and twins (50) showed convincingly that roughly 70% of within-population variation in BMI may be attributable to genetic variations among individuals. Although some family and adoption studies provide lower estimates than this 70% estimate of heritability (51), these studies are not well suited to capture the complex and non-additive nature of the genetic influences on obesity (52). Twin studies, which are far better suited to this purpose, have shown that genetic sources explain 50–90% of variation in BMI (53). In a study of same-age, unrelated siblings reared together since infancy (i.e., at least one sibling was adopted), 61% of the variance was genetic, mostly non-additive, 25% of the variance was due to a common or shared environment, and 14% of the variance was due to unique environment (54). Thus, heritability estimates in the range of 60–70% seem to be the most accurate for BMI.
Many people who wish to make beneficial changes to human health by altering the environment seem to see these figures as threatening or disheartening. There is in truth no need for this reaction. Heritability estimates of 60–70% imply that environmental factors explain the remaining 30–40% of the variance in BMI, which is a substantial proportion of the total variance. It implies, for example, that moving people merely one standard deviation down in environmental risk (i.e., making a moderate change to the environment) could decrease average BMI by two units (55). This would correspond to roughly a 10% reduction in relative weight, roughly the amount that can be achieved with the best available pharmaceuticals today, illustrating the power of the environment even in the face of strong heritability.
Contributions from the Environment
Obesity rates in the US have risen dramatically during the past 50 years. Since this is too short a period for most allele frequencies to change significantly, changes in the environment are important, though genetic factors that increase susceptibility to environmental influences are also important (5, 56–58). Factors such as the physical environment, the social environment, and the economic environment have all presumably contributed to an increase in the prevalence of obesity (5). The physical environment includes factors such as pollutants and increased reliance on automobiles. The social environment includes recreational eating, continuous advertising of unhealthy food products, and the availability of larger portion sizes. These factors as well as others may be involved (5, 58–60). The association with obesity of putatively influential environment factors has been demonstrated in studies of twins with a high genetic predisposition to obesity. The more active twin of a twin pair is generally less obese (61). Further evidence of environmental effects has come from studies showing that immigrants to the US usually show marked differences in the incidence of obesity compared to their counterparts who remain in their native countries. These observations also show the relationship between our genes and the environment (57, 62).
According to the thrifty genotype hypothesis, genotypes promoting the storage of carbohydrate as fat during times of plentiful food were once advantageous because the stored fat provided energy during times of food scarcity (8). In the modern era of chronically plentiful food, these same genetic variants may lead to obesity, diabetes mellitus, and insulin resistance. The concept of thriftiness was modified to consider effects on the fetus, whereby the fetus adapts to an adverse (nutrient-poor) intrauterine environment by optimizing nutrient use (9). This adaptation results in the development of some fetal organs at the expense of others, which may predispose to adult disease (9). Evidence supporting thriftiness and the identification of thrifty genes have both proven to be elusive (10–12).
Several authors recently reviewed evidence that intrauterine and maternal effects can predispose offspring to obesity. Maternal pregravid weight, maternal weight at delivery, maternal weight gain during gestation, parity, maternal insulin sensitivity, infant birth weight, and postnatal infant growth have all been proposed to affect obesity risk (63). Current research is exploring the idea that events that occur during critical developmental periods early in life (prenatal, perinatal, and postnatal) may influence an individual’s risk of disease later in life, a concept called “metabolic programming” (64–65) or “metabolic imprinting” (66). Even paternal preconceptional influences have been posited (67).
Another recently developed idea is that environmentally acquired alterations in adiposity may be transmitted to generations beyond the parents’ immediate offspring, due to what are termed epigenetic factors (58, 60, 68). This proposes that environmental signals may be passed to grandchildren and subsequent generations through effects on the genome, even if those generations did not experience the original signal (69, 70).
Specific Genes
Obesity is a consequence of a chronic disruption of energy balance. When energy intake exceeds energy expenditure, and when nutrient partitioning favors lipid storage and carbohydrate oxidation, the resulting imbalance causes expansion of existing fat cells and proliferation of new fat cells (71). Hereditary factors involved in obesity are associated with three types of biochemical processes: 1) the food intake biochemical pathway, which determines the sensations of satiety and hunger, and hence the amount of food eaten; 2) the energy metabolism process, which controls the degree to which energy from food is used or stored; and 3) the adipogenesis process, which creates adipocytes, cells specialized in fat storage (72). Obesity can be artificially categorized into monogenic obesity and polygenic obesity, based on the number of genes believed to be involved. A comprehensive listing of genes related to obesity can be found at the Obesity Gene Map Database (73).
Monogenic Obesity
Monogenic forms of obesity are rare, severe, and start in childhood. These single-gene disorders include cases in which obesity is one of many clinical symptoms (74–75). More recently, several monogenic disorders (discussed below) resulting from the disruption of metabolic pathways have been identified.
Syndromic Obesity or Mendelian Disorders
Although some of the syndromic obesity genes have been molecularly characterized, the pathophysiological links between their protein products and the development of obesity and other related clinical traits are still unclear. Examples of Syndromic Obesity Disorders are described in more detail in Box 5.2.
Box 5-2 Some Syndromic Obesity Disorders
Prader-Willi Syndrome (PWS): PWS is perhaps the most common of the obesity syndromes (prevalence 1/25,000 births). PWS is an autosomal dominant disorder characterized by obesity, reduced fetal activity, muscular hypotonia at birth, short stature, small hands and feet, mental retardation, hypogonadism, and hyperphagia. This disorder is caused by the lack of the paternal chromosomal segment 15q11–q13, either through deletion of the region or loss of the entire paternal chromosome 15. Several candidate genes in the deleted region have been studied but none has been implicated in the syndrome with certainty (75).
Bardet-Biedl Syndrome (BBS): BBS is a rare autosomal recessive syndrome (prevalence <1/100,000 births) characterized by obesity, mental retardation, pigmentary retinopathy, polydactyly, hypogonadism, and renal abnormalities. Although initially described as a recessive disorder, recent data have unmasked a mode of disease transmission in which mutations in at least eight different genetic loci can interact in some families to cause and/or modify the phenotype (76).
Albright Hereditary Osteodystrophy (AHO): AHO is an autosomal dominant disorder characterized by obesity, short stature, round face, brachydactyly, bone formation in non-bone tissue (ectopic tissue ossification), mental retardation, and low blood calcium (hypocalcaemia). The prevalence of AHO is 1/139,000 in Japan. AHO is due to parental imprinting of mutations in a specific gene (see Epigenetics, below, for a description of imprinting), the GNAS1 (guanine nucleotide binding protein α-stimulating activity polypeptide 1) gene, resulting in reduced expression or altered function of its protein product (77).
Cohen Syndrome: Cohen syndrome is an autosomal recessive disorder due to a gene that was mapped to chromosome 8q in several Finnish families. Besides obesity, Cohen syndrome is characterized by mental retardation, microcephaly, characteristic facial features, and myopia. The COH1 gene was shown to carry mutations in many Cohen syndrome patients from different ethnic groups (78).
Alström Syndrome: Alström syndrome is a homogeneous autosomal disease of childhood characterized by obesity, retinal cone dystrophy, dilated cardiomyopathy, type 2 diabetes, and other clinical traits of variable severity, such as hypothyroidism, small size, hypogonadism, renal abnormalities, and some mental retardation. Mutations in a single gene, ALSM1, have been associated with all cases of Alström syndrome (79).
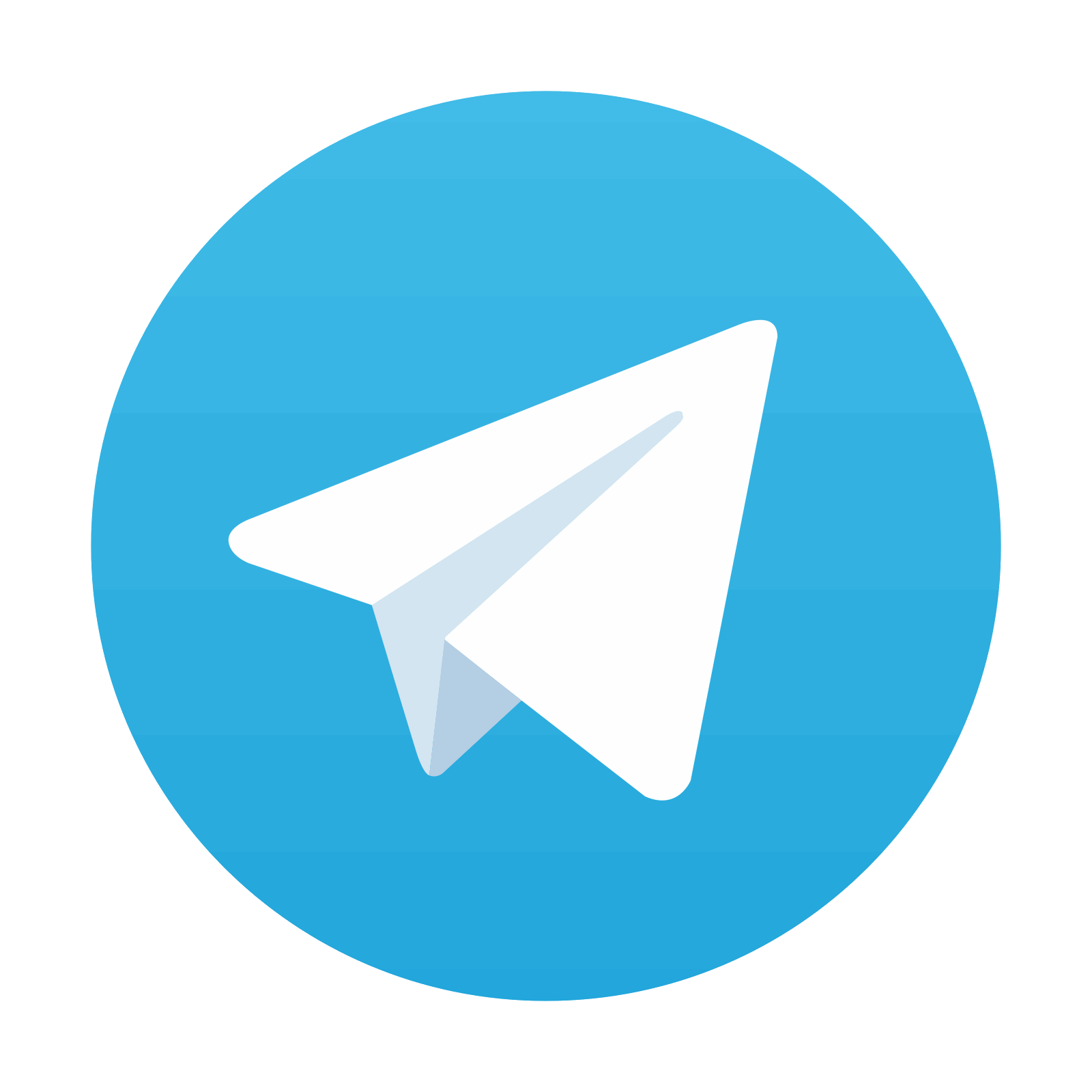