Fig. 13.1
Neuropathological hallmarks of pantothenate kinase-associated neurodegeneration (PKAN), representative of NBIA. (a) Typical Perl’s stained iron deposits in the globus pallidus of a PANK2-positive case. Scale: 200 μm, low magnification. (b) Typical presence of smaller neuroaxonal spheroids (arrow) and large degenerating neurons (arrowhead) in the globus pallidus of a PANK2-positive case. Hemosiderin in the background, scale: 50 μm (a, b, reproduced with permission from Kruer et al. [7], by permission of Oxford University Press). (c, d) Section through the basal ganglia in PANK2-positive case, hematoxylin and eosin (H&E) stain with pale area representing neuronal loss (c) and typical Perl’ s stain with blue representing excess iron (d) (c, d, Images courtesy of Prof. T. Revesz and Dr. A. Li, Queen Square Brain Bank, UCL)
In compliance with its heterogeneous nature, alpha-synuclein and Lewy body pathology [11, 13, 14] and TDP-43 and tau pathology [15–18] have additionally been observed for some subtypes of NBIA (for summary and overview, see Table 13.1).
Table 13.1
Overview of NBIA conditions, genes, MRI findings, and neuropathology in order of appearance throughout the chapter
Condition (acronym) | Synonym(s) | Gene | Cytogenetic band (Ensembl) | MRI features and areas of highest iron density | Inclusion type additional to iron and predominant region | Additional neuropathological findings |
---|---|---|---|---|---|---|
PKAN | NBIA1 | PANK2 | 20p13 | Iron deposits with highest density in GP, “eye-of-the-tiger” sign | Tau or none | Spheroid bodies throughout CNS, focussed in GP,variable involvement of putamen and internal capsule. Tau pathology possible. Only occasional peripheral manifestation |
PLAN | NBIA2, PARK14 | PLA2G6 | 22q13.1 | Area of highest iron in GP, milder SN involvement. Cerebral and cerebellar atrophy possible | Alpha-synucleinopathy with cortically widespread Lewy bodies, additional tau pathology | Central and peripheral neuroaxonal spheroids. Widespread cortical alpha-synuclein-positive stainings, Lewy body pathology. Additional presence of tau. Neurodegeneration in brainstem, cerebellum, optic pathway, and spinal cord |
MPAN | NBIA4, SPG43 | C19orf12 | 19q12 | Iron deposits in GP and SN | Alpha-synucleinopathy with cortically widespread Lewy bodies, additional tau pathology | Neuronal loss, axonal spheroids, iron deposits in globus pallidus and substantia nigra, Lewy bodies, Lewy neurites in globus pallidus, neurofibrillatory tangles, and tau-positive inclusions. Loss of myelin: pyramidal tracts, optic nerve |
BPAN | NBIA5, formerly: SENDA syndrome | WDR45 | Xp11.23 | Specific substantia nigra hyperintensity with central hypointensity on T1, iron accumulation in GP and SN, mild cerebellar atrophy and white matter changes possible | Tau (personal communication) | Widespread tangles and threads, distribution and neuronal autophagy-associated markers under current observation |
FAHN | SPG35 | FA2H | 16q23.1 | Iron deposits with focus in GP. White matter changes. Thin corpus callosum | Not known yet | No human brain data. Peripheral nerve biopsy: decrease of myelinated nerve fibers. Animal models with cerebellar abnormalities, demyelination, profound axonal loss throughout CNS |
Kufor-Rakeb disease | PARK9 | ATP13A2 | 1p36.13 | Atrophy of GP and pyramids. More generalized brain atrophy in later disease course. Highest iron content mostly in putamen and caudate | Not known yet – clinically and genetically significant overlap with lipofuscinoses | Widespread neuronal and glial lipofuscinosis sparing white matter, whorled lamellar inclusions in electron microscopy in one ATP13A2-positive NCL case. Peripheral nerve biopsy: cytoplasmic inclusion bodies |
Neuroferritinopathy | NBIA3, hereditary ferritinopathy, FTL | FTL | 19q13.33 | Iron deposits in SN > caudate, GP, red nuclei, and putamen. Cystic necrosis in basal ganglia and bilateral pallidum | Tau | Spherical inclusions positive for ferritin staining in cerebellum, posterior putamen, and other iron-rich areas; spheroids and neurofilaments immunoreactive to ubiquitin and tau. Additional hepatic iron deposits possible |
Aceruloplasminemia | – | CP | 3q25.1 | T2-weighted MRI with marked hypointensity of thalami, basal ganglia-nuclei, dentate. Most prominent iron deposits in basal ganglia, dentate nuclei > thalami, cerebral and cerebellar cortices | No alpha-synucleinopathy. Additional iron deposits in internal organs (mainly pancreas/liver). | Purkinje cell loss in cerebellum. Globular structures in astrocytes > neurons. No alpha-synuclein reactivity. Positivity to anti-4-hydroxynonenal and anti-ubiquitin antibodies |
CoPAN | NBIA6 | COASY | 17q21.2 | “Eye-of-the-tiger”-like MRI in 1 subject, 2nd subject: isolated involvement of neostriatum preceded evidence of typical pallida iron content increase | Not known yet | Not known yet |
Despite ongoing advances in elucidating its various pathophysiologies, the treatment of NBIA disorders to date essentially remains symptomatic. NBIA disorders can be inherited in autosomal dominant, autosomal recessive, and x-linked fashion. Up to now, at least ten genes are known to be associated with neurodegeneration with brain iron accumulation, nine of which will be discussed in more detail in separate sections below. The genetic underpinnings of the disease which are in the focus of this review are as heterogeneous as its clinical phenotypes [19].
Firstly, NBIA disorders can be caused by mutations in genes with a direct link to iron metabolism. The most famous examples thereof are mutations in the ferroxidase encoding gene ceruloplasmin (CP) leading to aceruloplasminemia [20, 21] or pathogenic mutations in the gene ferritin light polypeptide (FTL) that lead to hereditary ferritinopathies as this gene encodes the light subunit of the ferritin protein responsible for iron storage and release [22–25]. Secondly, most of the disease-associated mutations in NBIA impair genes in phospho- and sphingolipid metabolic pathways [26], mitochondrial or lysosomal activity [27, 28], and the mechanisms linking those to iron metabolism are less clear. Finally, the ultimate hope in studying NBIA disorders is to use this presence of overlapping clinical features and molecular/biological pathways in order to identify new therapeutic alleys, not only for these rare disorders but also for more common disorders as Parkinson’s disease (PD) and dystonia that share elements of pathophysiology.
This review gives an overview about the distinct genetic defects that lead to the different, yet overlapping, phenotypes of NBIA disorders, highlights important phenotypic presentations that direct genetic testing where possible, provides information about treatment options when present, and discusses remaining controversies of this highly dynamic field of neurodegeneration.
Pantothenate Kinase-Associated Neurodegeneration (PKAN)
PKAN, also recognized under the acronym NBIA1, accounts for about 35–50 % of NBIA cases [29]. However, there is a possibility of overestimation depending on the cases screened and possibly confounding selection bias. It has an estimated worldwide prevalence of about 1:1,000,000 with regional higher prevalences (e.g. Dominican Republic) due to founder effects. It was formerly assessed under the name Hallervorden-Spatz syndrome (HSS) – a terminology that has been abandoned due to the unethical implications of these researchers under the Nazi regime but can still be found sporadically for historic reasons [30].
Clinically, PKAN can be divided into two categories, atypical and typical PKAN. Typical PKAN patients usually become symptomatic with gait and postural abnormalities around the age of 3–4 [29, 31]. Their mutational spectrum tends to show variations in PANK2 that lead to protein truncation more often than mutations in atypical, late-onset PKAN cases which tend to be amino acid changes without consequent truncation effects [29]. Typical PKAN patients present with a more rapid but nonlinear progression to a full clinical spectrum of severe (in its early manifestation predominantly oromandibular and nonaxial) dystonia [29, 32], spasticity and hyperreflexia, parkinsonism, chorea, impaired saccadic pursuits as well as decelerated and hypometric vertical saccades, mental decline, and behavioral abnormalities [29, 33]. Pigmentary retinopathy occurs in up to 40 % of typical PKAN cases, whereas optic atrophy is infrequent [29, 34]. Given the disease progression with rapid deterioration intertwined with phases of stability, the majority of patients are nonambulatory within at most 15 years into the disease [29].
Atypical PKAN cases tend to be significantly older when the first obvious symptoms appear (age of onset at 13.7 ± 5.9 years [range, 1–28] in atypical vs. 3.4 ± 3.0 years [range, 0.5–12] in typical cases as reported in the original paper by Hayflick et al., 2003) [29]. Their pyramidal and extrapyramidal manifestations are generally milder and progress less rapidly; however, most atypical PKAN patients eventually lose their ambulatory independency [29]. Furthermore, speech defects like dysarthria or palilalia as well as psychiatric features like cognitive decline seem to be more prevalent in atypical cases [29].
In terms of radiographic findings, the eye-of-the-tiger sign, a T2-weighted hypointense signal in the globus pallidus (GP) with a central region of hyperintensity, has lost its previously acclaimed pathognomonic status for PANK2 [29, 35]. Patients with other genetic forms of NBIA or with a different neurodegenerative disease have been found to hold this sign [8, 36] as well as PANK2-positive patients lacking it [37]. The eye-of-the-tiger sign can even be present in individuals without any clinical symptoms [38]. Nonetheless it remains an important flag in clinical diagnostics pointing towards the group of NBIA disorders.
The human gene which is associated with this condition is PANK2. PANK2 finds itself on chromosome 20, p13 [39]. It encodes the only human protein from the pantothenate kinase family that is thought to be expressed in mitochondria and therefore seems to be responsible for the intramitochondrial de novo synthesis of coenzyme A [39]. Pantothenate kinase is the key regulatory enzyme in coenzyme A synthesis and catalyzes the first step from pantothenate/vitamin B to 4’phosphopantothenate in this universally important pathway. Given its role in fatty acid metabolism, amino acid synthesis, and Krebs cycle, CoA is vital for the basic key metabolism of the cell [40].
PKAN is inherited in autosomal recessive manner. Its mutational spectrum includes mostly missense and nonsense mutations, which – depending on the location of the mutation and the corresponding conservation throughout species – result in reduced or no activity of pantothenate kinase in mitochondria. Additionally, deletions up to 6 kb, whole exon deletions, and splice site mutations and duplications have been observed, and all 7 exons of the gene can be affected [41]. A screening study of 2006 enrolled 72 patients with a clinical diagnosis of NBIA and could identify both mutated PANK2 alleles in 48 patients. Thirty-three different mutations – mostly missense and nonsense – were reported; deletions of the gene were found in around 4 % of the mutated PANK2 alleles [41]. The most frequent mutations in their study were c.1583C > T/p.Thr528Met (n = 11), c.573delC/p.S191RfsX13 (n = 10), and c.1561G > A/p.Gly521Arg (n = 10). Over the years, authors used different transcripts to refer to the same common mutations in PANK2, while in effect c.1561G > A is found to be equal to c.1231G > A and c.1583C > T to c.1253C > T. In order to avoid confusion, we will only refer to the amino acid change in the following although even a certain amount of different annotations can be found throughout the literature. With increasing evidence, p.Gly521Arg (allele frequency ~ 25 %), p.Thr528Met (~8 %), and p.Arg451Ter (~3 %) are found to be the most common pathogenic variants [42, 43], while the residual spectrum of PANK2 mutations includes mainly private mutations [29]. Two thirds of PKAN patients are compound heterozygotes [43]. As per example, one of the most common point mutations, leading to amino acid change p.Gly521Arg, has been shown to result in reduced production of the mature isoform of the PANK2 enzyme [40]. Regarding the molecular pathology of PKAN, it has therefore been suggested that mutations in PANK2 lead to reduced PANK2 protein levels, impaired catalytic activity of PANK2, and consecutively altered neuronal mitochondrial lipid formation and metabolism [40]. However, it has to be noted that not all disease-associated point mutations result in significantly reduced catalytic activity of the enzyme [40]. The final transformation into clinical symptoms still remains equally elusive, and few attempts have been made to combine the mutational location and corresponding clinical features: As one example, Hartig et al. introduced an activity score to take into account the severity of the identified mutations and the predicted rest activity of the enzyme. They found the activity score of the mutations to be correlated with the age of onset of the disease, but not the loss of ambulation in their PANK2-positive cases [41]. In a different attempt, a correlation between the location of missense mutations and their biochemical properties with the disease features found mutations located in the dimer interface, the ATP binding site, and the interior of the protein to negatively impact the enzyme activity and to be mostly associated with classic, young-onset, and rapidly progressing disease. Mutations located on the surface that lead to slight instability, but seemed to preserve most of the enzyme’ s catalytic activity, were associated with milder symptom presentation of PKAN [44]. Even though genotype-phenotype correlations in PKAN remain controversial and difficult, it is these arduous and small advances in trying to correlate the specific location of a mutation with functional and phenotypical properties that will shed further light on the gap between the genetic underlying causes and their transformation into clinical manifestations and will ultimately facilitate genetic counseling. However, one important restriction in our field of extremely rare conditions will always be the inherently small amount of cases available.
Despite the remaining gaps, as of current state of knowledge, some conclusions can be retained: As shown in the original work by Hayflick and colleagues, patients homozygous for the common p.Gly521Arg amino acid change have classic disease [29]. Furthermore, two null mutations seem to result in rapidly progressive, early-onset PKAN, while missense mutations with diminished but preserved enzyme function are likely to lead to atypical PKAN with later onset and slower progression. In our own clinical experience, we have now seen several compound heterozygous cases that seem to lead to atypical PKAN: Late disease onset in these cases goes along with rather preserved cognition, slow progression, and longer lifespan.
Neuropathologically, PKAN is part of the neuroaxonal dystrophy spectrum with two main classes of spheroids observed: large, granular bodies representing degenerating neurons and smaller eosinophilic spheroid-like structures indicative of dystrophic neurites [7]. Prior to gene identification, numerous cases of heterogeneous etiology under the former umbrella term “Hallervorden-Spatz syndrome” have been reported to show Lewy body pathology [13, 45–47]. These have been genetically unconfirmed cases which renders the interpretation of this histopathological finding uncertain and less trustworthy as retrospectively these cases are most likely to be PANK2-negative cases with possibly heterogeneous but finally undetermined underlying genetic defects. However, a recent neuropathological investigation strictly including only PANK2-positive patients observed no alpha-synuclein positivity, but some tau-positive inclusions, and concluded that PKAN is not a synucleinopathy [16] which neuropathologically distinguishes it from other NBIA disorders (PLAN, MPAN) where alpha-synuclein accumulation can occur.
Furthermore, the disease has been shown to be allelic to HARP syndrome, depicting hypoprebetalipoproteinemia, acanthocytosis, retinitis pigmentosa, and pallidal degeneration [48] which is now likewise considered to be part of the PKAN- continuum. PKAN is the only NBIA disorder where iron-mediating medical treatment has systematically been tested, and while treatment with the iron chelator deferiprone leads to a median reduction of 30 % of pallidal iron content, clinical benefits unfortunately remained nonsignificant in the initial report [49], while the recent four-year follow-up report has shown clinical stabilization upon treatment in 5/6 patients [50]. Furthermore, it is the only NBIA subgroup where preliminary data about the use of deep brain stimulation (DBS) of globus pallidus internus bilaterally in the treatment of dystonia are available [51, 52] showing that it may produce clinical benefit for the postoperational time observed but does not stop the ongoing neuronal degeneration.
PLA2G6-Associated Neurodegeneration (PLAN)
PLA2G6-associated neurodegeneration (PLAN) accounts for approximately 20 % and thereby for the largest proportion of NBIA disorders after PKAN [53]. Three main phenotypes have been described within the spectrum of PLA2G6-associated disease that is a striking example of allelic heterogeneity: classic infantile neuroaxonal dystrophy (INAD) [54], childhood-onset atypical neuroaxonal dystrophy (NAD) [14, 55], and adult-onset dystonia-parkinsonism [5]. Their unifying features are progressive cognitive decline and rapid deterioration of motor skills, but the three syndromes can be distinguished via the following:
INAD is attended by a disease onset between 6 and 36 months and usually manifests with initial hypotonia, developmental regression, progressive psychomotor delay, cerebellar features, bulbar dysfunction, and progressive spastic tetraparesis [55–58]. MRI findings include cerebellar atrophy, and electromyography typically shows denervation atrophy [59, 60]. Optic atrophy, fast rhythms on electroencephalogram (EEG), and seizures may be present. Prior to gene identification, the definitive diagnosis was confirmed on sural biopsy, depicting typical dystrophic axons in the peripheral nerves [57]. Most INAD patients die within the first decade of their life; isolated evidence suggests some surviving up to their early twenties [42].
Atypical NAD is less common than INAD and usually manifests between the first and eighth year of life, while a delayed onset in the late twenties is possible [58]. The disease course is less aggressive with a slower progression rate. Prominent symptoms are ataxia, spastic tetraparesis, dystonia, speech delay, and behavioral abnormalities ranging from impulsivity, poor attention span, and emotional lability to autistic behavior [14, 55]. Seizures, optic atrophy, and nystagmus seem to occur in NAD as frequently as in INAD, while fast rhythms, truncal hypotonia, and strabism are predominantly part of INAD [14].
The third phenotype, PLA2G6-related adult-onset dystonia-parkinsonism, is characterized by a later (second to third decade), subacute onset of dystonia-parkinsonism with rapid decline and initial dramatic response to levodopa. Pyramidal involvement, eye movement abnormalities, dystonia, dysarthria, psychiatric features, cognitive decline, and gait abnormalities were further common findings. Brain iron deposits were absent on MR imaging of these patients, whereas cerebral and cerebellar atrophy was prominent [5].
Phospholipase A2-associated neurodegeneration is inherited in autosomal recessive manner and caused by mutations in the widespread expressed gene PLA2G6 [61]. PLA2G6 is located on chromosome 22q and comprises 17 exons which underlie alternative splicing and result in the production of different isoforms [61]. The gene was discovered in 2006 following a linkage approach in families with INAD and NBIA [54] and was subsequently found to be mutated in the related Karak syndrome [62] which is now recognized to be part of atypical NAD. Prior to this discovery, the diagnosis was solely to be made clinically or histopathologically, and confirming the diagnosis via molecular genetic testing has since proven useful to reduce invasive tissue biopsies and alleviate genetic counseling.
In the initial study, the majority of 44 identified unique mutations were homozygous missense mutations [33], followed by frameshift-inducing deletions [5], nonsense mutations [3], deletions without subsequent frameshift [1], a splice site mutation, and a large deletion. Most mutations are private mutations. This frequency spectrum has since been reproduced in a slightly larger cohort [14]. So far, homozygous null mutations were only found in patients with INAD and not in individuals of the broader clinical category with iron dyshomeostasis without axonal spheroids [54] suggesting that mutations resulting in absent protein seem to be associated with severe phenotypes. As in PANK2, compound heterozygous missense mutations suggestive of remaining protein function correlate with the atypical, milder NAD phenotype [14].
The 85 kDa calcium-independent group 6 phospholipase A2 (iPLA2-VI) is the encoded protein which plays an important role in the maintenance of cell membrane homeostasis, regulation of apoptosis, leukotriene and prostaglandin synthesis, catalysis of glycerophospholipid hydrolysis, and phospholipid remodeling [5, 14, 42, 53, 61, 63, 64]. Multiple isoforms are encoded by the PLA2G6 transcript variants, whereof two are enzymatically active [61]. A subset of the reported mutations in the literature was found to alter the shorter enzymatically inactive isoforms and when incorporated into the tetramer iPLA2-VI acts as dominant-negative inhibitors [61, 63]. Therefore, defects in iPLA2-VI result in impaired phospholipid remodeling which is an essential component of physiological membrane repair and homeostasis [65]. Additionally, iPLA2-VI can initiate apoptosis when exposed to oxidative stress [66], and deficiency in these vital cell protection and repair mechanisms results in neuronal damage and degeneration.
Histopathologically, the hallmark finding in PLAN is the presence of neuroaxonal spheroids which is thought to represent the degeneration of the inner mitochondrial membrane [67, 68]. This neuroaxonal degeneration with distended axons (spheroid bodies) is mainly present in the CNS but has been reported in peripheral nerves as well [57]. Furthermore, Lewy body pathology alongside tau-positive inclusions has been observed [69]. A second common finding is progressive brain iron deposition that can be detected neuropathologically as well as neuroradiologically with some time into the disease. Typical iron deposits in the globus pallidus can be missing in the initial radiographic brain imaging of INAD cases but usually develop within a certain course of the disease [54, 59]. Further common neuroradiographic features in PLAN are cerebellar hypoplasia or atrophy and T2-weighted high signal in the cerebellum, hypoplastic optic tracts/chiasm, and an elongated, vertically orientated splenium [59].
Mitochondrial Membrane Protein-Associated Neurodegeneration (MPAN)
Mitochondrial membrane protein-associated neurodegeneration (MPAN), also known as neurodegeneration associated with C19orf12 mutations, accounts for 6–10 % of NBIA disorders and is the third most prevalent group after PKAN and PLAN [53, 70, 71]. Disease onset usually is in childhood to early adulthood. Common features are speech and gait difficulties, cognitive decline resulting in dementia, spasticity, dysarthria, optic atrophy, dystonia, parkinsonism, psychiatric features, and motor neuronopathy with early signs of upper motor neuron dysfunction, followed by affection of the second motor neuron later. Its natural history is slowly progressive; survival into adulthood is not uncommon. MPAN is more than other NBIA disorders associated with rapid progressive cognitive decline [53, 70, 71].
Neuroimaging shows iron accumulation in the globus pallidus and substantia nigra (SN) as well as cortical and cerebellar atrophy. Hyperintense streaking of the medial medullary lamina between globus pallidus internus and externus on T2-weighted images can be additionally present and is sometimes mistaken for an eye-of-the-tiger sign [71].
MPAN is caused by mutations in the orphan gene C19orf12 and is inherited in autosomal recessive fashion. Homozygosity mapping followed by candidate gene sequencing identified the common founder mutation in C19orf12 NM_001031726.3:c.204_214del11/NP_001026896.2:p.Gly69ArgfsTer10 in a Polish family in 2009 [70]. Subsequent screening in a Polish cohort of additional 23 index patients with the above described phenotype and negative for mutations in PANK2, PLA2G6, FTL, and CP revealed homozygous mutations in C19orf12 in 18 of them. 12 carried the homozygous founder mutation which is thought to have arisen in a common founder at least 50–100 generations ago. Three remaining cases carried the deletion in combination with different missense mutations (p.Gly65Glu, p.Gly53Arg, and p.Thr11Met) in the compound heterozygous state. One remaining case carried two missense mutations, p.Gly69Arg and p.Lys142Glu, and two cases with homozygous missense mutations (p.Tyr11Met and p.Gly69Arg) were identified.
One additional compound heterozygous missense mutation (p.Gly69Arg and p.Lys142Glu) in C19orf12 was identified in a patient with a late-onset (49 years) predominantly parkinsonian phenotype and in one young Polish patient with a mild impairment of fine motor skills and is therefore thought to result in milder phenotypes. A follow-up screening study on a larger and ethnically more diverse cohort of 161 idiopathic NBIA cases was published in 2013. It identified two mutated alleles in 23 cases and established MPAN as the third most common subform of NBIA to date. In addition to the common founder mutation, a variety of different, mostly “private” mutations (nonsense, frameshift, and missense) were identified throughout C19orf12. There was no evidence for large deletions and duplications. However, the occurrence of a single heterozygous mutant allele in three cases suggests the presence of occult mutations in intronic and regulatory sequences [71]. Furthermore, in their study, one single-mutation case had a family history compatible with autosomal dominant inheritance backed up by histological findings in the deceased father of the family. The identified single unique frameshift mutation induces a series of 32 amino acid substitutions and ultimately results in a premature stop codon 2 amino acids away from the physiological termination. Therefore, the authors discuss the possibility of this aberrant product escaping physiological nonsense-mediated mRNA decay and thereby presumably exerting dominant-negative effects on the normal protein. However, further studies are awaited to elucidate the pathogenesis of this single mutation and finally clarify the possibility of additional autosomal dominant inheritance in C19orf12 [71].
Most evidence in C19orf12 pathogenesis has been collected regarding the common founder mutation NM_001031726.3:c.204_214del11/NP_001026896.2:p.Gly69ArgfsTer10. This homozygous 11-bp deletion results in a frameshift that introduces a premature stop codon and is predicted to cause early truncation of the protein. Even though the deletion did not alter C19orf12-RNA levels in blood, the protein was undetectable with immunoblot analysis in patients’ fibroblasts [70]. The missense mutation p.Lys142Glu changes a highly conserved positive lysine to a negatively charged glutamate. Three different missense mutations p.Gly53Arg, p.Gly65Glu, and p.Gly69Arg replace highly conserved glycines in the transmembrane region of C19orf12 with a charged amino acid and therefore are likely to alter its function.
The exact gene function of C19orf12 is unknown, but functional expression analyses suggest its mitochondrial location [70]. Analysis of respiratory chain complexes and mitochondrial morphology in fibroblasts of C19orf12-positive patients could not detect any significant abnormalities. However, analysis of C19orf12-coregulated genes in whole blood interestingly revealed strongest coregulation with genes involved in valine, leucine, and isoleucine degradation as well as fatty acid biogenesis. These processes are related to coenzyme A metabolism in mitochondria and therefore link MPAN to previous NBIA disorders with impairment in CoA pathways and lipid homeostasis (PKAN, PLAN, CoPAN (see later)).
Postmortem brain histopathology in two cases showed neuronal loss, iron deposits in the globus pallidus and substantia nigra, Lewy bodies, Lewy neurites in the globus pallidus, axonal spheroids, neurofibrillatory tangles, and tau-positive inclusions. The pyramidal tracts of the spinal cord and optic nerve displayed severe loss of myelin in one of them [70, 71].
Beta-Propeller Protein-Associated Neurodegeneration (BPAN, Formerly SENDA Syndrome)
Beta-propeller protein-associated neurodegeneration (BPAN) is also formerly known as static encephalopathy of childhood with neurodegeneration in adulthood.
(SENDA syndrome) accounts for about 1–2 % of NBIA disorders based on its prevalence in the International Registry for NBIA and Related Disorders from the Hayflick laboratory [53] and ~7 % according to a recent literature review [72].
Affected cases show a distinct phenotype of psychomotor developmental delay (mental retardation and early-onset spasticity of the lower limbs) in early childhood which remains fairly stable until a sudden onset and rapidly progressive complex of symptoms including dystonia, parkinsonism, and spasticity occurs in adulthood. Eye movement disorder, sleep disturbances, dysautonomia, seizures, and frontal release signs can additionally be present, and parkinsonian features usually show good response to l-dopa even though early motor fluctuations and dyskinesias often develop [1, 73]. Brain MRI shows a specific substantia nigra hyperintensity with central hypointensity on T1, iron accumulation in the globus pallidus and SN, and mild cerebellar atrophy along with white matter changes [74]. Preliminary brain pathology showed widespread tangles and threads in a single case (J. Holton, personal communication, 2014), and more BPAN patients will need to be autopsied to complement these observations.
Only recently, exome sequencing identified the underlying previously unknown genetic cause of this disease: De novo mutations in the beta-propeller scaffold protein WDR45 at chromosome Xp11.23 were reported in affected SENDA cases establishing BPAN as the first x-linked dominant NBIA disorder [27]. All reported variants had unique occurrence in the index patient of the sequenced families suggesting de novo events, and loss-of-function mutations account for the majority of so far reported variants, followed by missense mutations in highly conserved residues [27, 73]. The majority of reported WDR45-positive cases are females [73, 75], while phenotypes of affected males do not substantially differ from females [27] which might be due to somatic mosaicism in the few surviving males and somatic and/or germline mutations and skewed X-chromosome inactivation in females.
WDR45 encodes WD repeat domain 45, one of the mammalian homologues of the autophagy-related gene Atg18 in yeast that is thought to be a human core autophagy gene important in the early autophagy pathway [75–77]. In a recent experiment, WDR45-positive patient-derived lymphoblastoid cell lines showed accumulation of aberrant early autophagic bodies and decreased autophagic activity [75]. Together with the original findings [27], this provides further evidence for a strong connection between neurodegeneration and genetically defective autophagy.
Fatty Acid Hydroxylase-Associated Neurodegeneration (FAHN)
Fatty acid hydroxylase-associated neurodegeneration (FAHN) is another rare form of neurodegeneration with brain iron accumulation. It includes dysmyelinating leukodystrophy and spastic paraparesis with or without dystonia/spastic paraplegia 35 [78, 79], which had been recognized as distinct clinical entities previously but have now been included into the spectrum of FAHN [80, 81]. Clinically, FAHN is characterized by a pyramidal-extrapyramidal movement disorder with predominant gait difficulties due to severe spasticity and ataxic and dystonic features alongside to progressive intellectual impairment and the likely occurrence of optic atrophy, oculomotor abnormalities, and seizures. Usually, the disease manifests itself in childhood, but at the latest in the first or second decade of life. Typical neuroimaging features include bilateral GP hypointensity on T2-weighted images, white matter lesions, mild cortical and pronounced pontocerebellar atrophy, and a thin corpus callosum [82]. Thin corpus callosum is not a common feature in the remaining NBIA disorders and should direct genetic testing to mutations in FA2H. However, it can be observed more frequently in other complex hereditary spastic paraplegias, mostly SPG11 and SPG15 [83, 84].
To date, less than 30 patients from different ethnic backgrounds are reported [78–80, 82, 85–87]. Reliable data on prevalence are still missing. Characteristics of the phenotype as well as the natural history of the disease can therefore only be reported with limited certainty and need to await further confirmation with more cases arising [87].
Neuropathological data from postmortem brain analysis have not yet been reported in this disorder. Sural nerve biopsy of one FA2H-positive case revealed a decrease of myelinated nerve fibers with intact myelin compaction and a normal amount of nonmyelinated fibers and normal ratio of large to small fibers [79]. Available muscle biopsy in another case from Dick et al. showed denervation and reinnervation without evidence of mitochondrial disease [78]. However, in another reported case, biopsies of the skin, muscle, and sural nerve were found to be normal [86], and although not mandatory for diagnosis, bone marrow biopsy in FA2H-positive patients may show accumulation of granular histiocytes. In animal models of FA2H, demyelination and profound axonal loss in the CNS as well as cerebellar abnormalities have been observed after 12 months, while at that timepoint peripheral nerves were principally normal in function and structure and only exhibited later-onset peripheral demyelinating and axonal neuropathy [88].
Fatty acid hydroxylase-associated neurodegeneration is inherited in autosomal recessive manner and is caused by mutations in the gene FA2H on chromosome 16q23.1. Human FA2H has seven exons and encodes the fatty acid 2 hydroxylase which alpha-hydroxylates incipient fatty acids enzymatically. The hydroxylation is required for the fatty acids in order to be incorporated into subsequent lipids that make up cell membranes and form myelin structures. All reported sequence variants in FA2H to date are “private” mutations, and it is thought that they exert their effect through a loss-of-function mechanism. Identified mutations lead to premature termination of the protein, and reduced protein levels of FA2H have been detected in D6P2T cells transfected with pcDNA-hFA2H R154C [82]. However, due to its rare occurrence, the functional implications so far remain poorly studied and understood.
Kufor-Rakeb Disease (ATP13A2 Mutations/PARK9)
Kufor-Rakeb disease was originally described in a family with consanguineous background from Kufor-Rakeb, a small village in Jordan in 1994 [89]. The original paper reported juvenile-onset severe parkinsonian features in combination with pyramidal symptoms, dementia, and supranuclear upgaze paresis. Levodopa therapy usually improves the extrapyramidal dysfunction, while levodopa-induced dyskinesias are reported to develop early [90, 91]. MRI findings comprised severe atrophy of the globus pallidus and the pyramids and more generalized brain atrophy in later course of the disease. Later papers describe facial-faucial-finger myoclonus, visual hallucinations, autonomic dysfunction, slowing of saccadic pursuit and horizontal and vertical saccades, and oculogyric dystonic spasms in addition to the juvenile-onset parkinsonism, pyramidal signs, dementia, and supranuclear gaze palsy [90–93] and initially categorized Kufor-Rakeb disease as Parkinson’s disease 9/PARK9. It was another 12 years from the original report in 1994 until the underlying genetic defect, a compound heterozygous loss-of-function mutation (c.3057delC/c.1306 + 5G > A) in a previously uncharacterized neuronal P-type ATPase gene, ATP13A2, was identified in a large nonconsanguineous Chilean sibship in 2006. Subsequent mutation screening in the original Jordanian family revealed a homozygous 22 bp duplication (c.1632_1653dup22) in all affected individuals [28]. Reports on the incidence of brain iron accumulation in the putamen and caudate [92, 94] in a proportion of ATP13A2-positive patients led to the inclusion of this disease entity into the NBIA spectrum [94]. It has been hypothesized that occurrence of iron might be associated exclusively with more severe mutations or may only develop later in the disease course [95].
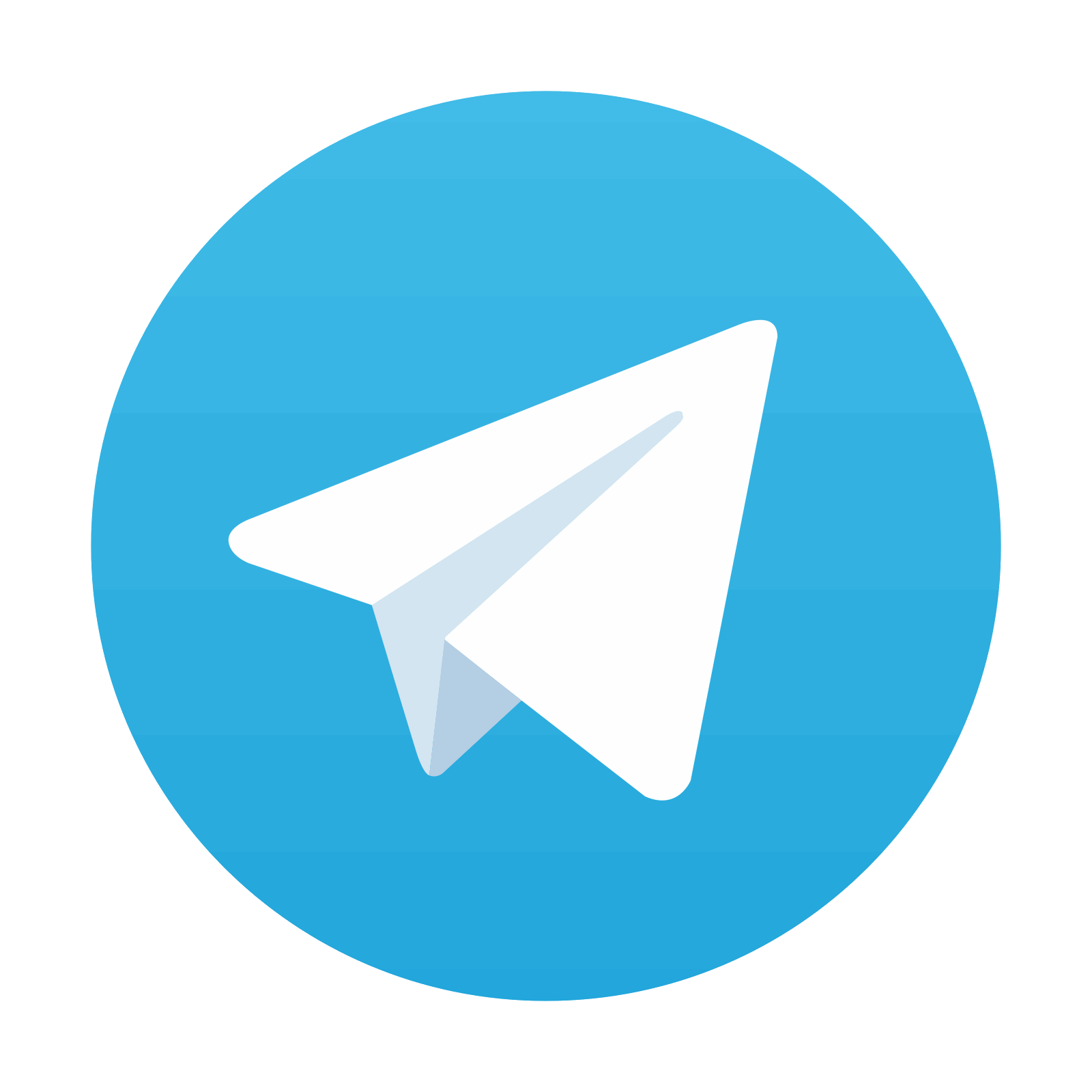
Stay updated, free articles. Join our Telegram channel

Full access? Get Clinical Tree
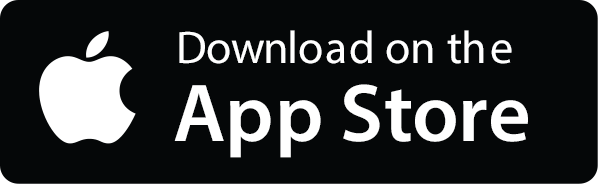
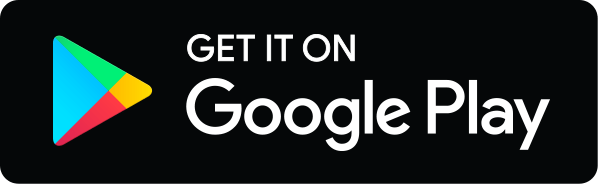