Fig. 9.1
Graphical representation of the WGCNA network. The center of the figure shows the overall network where the nodes (green circles) are modules and the edges are significant correlations (correlation p-value <0.05 and correlation coefficient >0.5) between the first principal component of the gene expression values. Top left: blue module. Top right: magenta module. Bottom right: lightcyan module. Bottom left: turquoise module. Circles: network genes. Large circles: top five genes with highest intra-modular connectivity (hubs). Squares: network genes overlapping with CNV genes. Red: upregulated genes. Blue: downregulated genes. Concentric circle arrangement in the case of the turquoise (bottom left) and magenta (top right) modules reflects intra-modular connectivity: genes closer to the center have higher intra-modular connectivity than genes further away from the center (Reproduced with permission from Lennington et al. [111])
Analysis of the distribution of the differentially expressed genes within the modules revealed a bias toward more central, highly interconnected genes for the magenta (microglia/immune) module and in the opposite direction for the other relevant modules, toward more peripheral, less interconnected genes (Fig. 9.1).
Finally, the authors integrated their network analysis with a list of genes intersecting rare CNVs from one of the studies described above [98], to identify genetic alterations as potential causative factors of the changes observed at transcriptome level. Interestingly, they found significant overlap between the subset of TS-specific genes and the turquoise and light cyan modules, respectively, with more peripheral (i.e., low-connectivity) and more central (i.e., high-connectivity) genes (Fig. 9.1). The lack of overlap between the magenta module (microglia/immune) and the CNV genes was somewhat surprising, given the widespread perturbation in gene expression levels, which also included many hub genes. Taken together with the reported lack of correlation between the magenta and the turquoise and/or light cyan modules, these findings may suggest that the immunological alterations are either not pathophysiologically dependent on the other alterations or that a previous relationship might have existed developmentally, but was lost as a consequence of disease [111].
Summary and Future Directions
As can be said for all genetically complex disorders, the process of gene discovery in TS to date has been challenging. Despite strong evidence that genes contribute to the disorder, there have been a considerable number of gene discovery efforts that have yet to account for a significant proportion of disease etiology. Nevertheless, there is reason for optimism given emerging evidence for the contribution of specific genes and biological pathways from rare variation and gene expression studies in TS (see Table 9.1) and given significant progress being made in other neuropsychiatric and neurodevelopmental disorders, particularly ASD and schizophrenia. Large-scale studies in both of these disorders have resulted in dramatic advances in the understanding of their respective genomic architectures, signaling a good prognosis for the future of TS genetics research.
Table 9.1
Tourette syndrome gene candidates, organized according to neurotransmitter class and functional categories (A-I) Type of study used in reference(s)
Gene | Description | Site | References | A | B | C | D | E | F | G | H | I |
---|---|---|---|---|---|---|---|---|---|---|---|---|
Histamine signaling | [98] | • | ||||||||||
HDC | Histidine decarboxylase | 15q21.2 | [91, 94, 96–99] | • | ||||||||
GABAergic signaling | [98] | • | • | • | ||||||||
GAD1 | Glutamate decarboxylase 1 | 2q31 | [111] | • | ||||||||
GABRA1 | Gamma-aminobutyric acid (GABA) A receptor, alpha 1 | 5q34 | [111] | • | ||||||||
GABRG2 | Gamma-aminobutyric acid (GABA) G receptor, gamma 2 | 5q34 | [111] | • | ||||||||
GABRA3 | Gamma-aminobutyric acid (GABA) A receptor, alpha 3 | Xq28 | [111] | • | ||||||||
NOS1 | Nitric oxide synthase 1 | 12q24.2 | [111] | • | ||||||||
NPY | Neuropeptide Y | 7p15.1 | [111] | • | ||||||||
NPY2R | Neuropeptide Y receptor Y2 | 4q31 | [111] | • | ||||||||
Cholinergic signaling | ||||||||||||
CHAT | Choline acetyltransferase | 10q11.2 | [10, 91, 111] | • | • | |||||||
CHRM2 | Cholinergic receptor, muscarinic 2 | 7q33 | [111] | • | ||||||||
GBX2 | Gastrulation brain homeobox2 | 2q37.2 | [111] | • | ||||||||
NTRK1 | Neurotrophic tyrosine kinase, receptor type 1 | 1q21-22 | [111] | • | ||||||||
SLC18A3 | VACHT; Solute carrier family 18 (vesicular acetylcholine transporter), member 3 | 10q11.23 | [111] | • | ||||||||
SLC5A7 | CHT1; Solute carrier family 5 (sodium/choline cotransporter), member 7 | 2q12 | [111] | • | ||||||||
Monoamine signaling | ||||||||||||
DRD1 | Dopamine receptor D1 | 5q35 | [111]; neg findings [43] | • | ||||||||
DRD2 | Dopamine receptor D2 | 11q23 | [40] | • | ||||||||
DRD4 | Dopamine receptor D4 | 11q15 | [37–39]; neg findings [41, 42] | • | ||||||||
DRD5 | Dopamine receptor D5 | 4p16 | [111] | • | ||||||||
HTR2C | Serotonin receptor 2C | Xq23 | [111] | • | ||||||||
MAOA | Monoamine oxidase A | Xp11.3 | [38] | • | • | |||||||
TPH2 | Tryptophan hydroxylase 2 | 12q21.1 | [50] | • | ||||||||
SLC6A3 | DAT; Solute carrier family 6 (neurotransmitter transporter), member 3 | 5p15.3 | [41, 45] | • | ||||||||
SLC6A4 | SERT; Solute carrier family 6 (neurotransmitter transporter), member 4 | 17q | [51] | • | ||||||||
Other neuronal signaling, axon guidance, etc | ||||||||||||
CNTNAP2 | Contactin-associated protein like-2 | 7q35-36.1 | [29, 36] | • | ||||||||
DNAJC13 | DNAJ homolog, subfamily C, member 12 | 3q21-23 | [108] | • | ||||||||
GPR63 | G protein-coupled receptor 63 | 6q16.1 | [64] | • | ||||||||
NLGN4X | Neuroligin 4, X-linked | Xp22.3 | [69] | • | ||||||||
NTN4 | Netrin 4 | 12q22 | [58] | • | ||||||||
SLITRK1 | SLIT and NTRK-like family, member 1 | 13q31.1 | [59, 83, 84]; neg findings [56, 85–90] | • | ||||||||
SLITRK5 | SLIT and NTRK-like family, member 5 | 13q31.2 | [111] | • | ||||||||
Bioenergetics/metabolism | ||||||||||||
IMMP2L | Mitochondrial inner membrane protease subunit 2 | 17q31.1 | [32, 60, 62, 65, 67, 68, 70] | • | ||||||||
MRPL3 | Mitochondrial ribosomal protein L3 | 3q21-23 | [108] | • | ||||||||
NDUFA4 | NADH dehydrogenase 1 alpha subcomplex, 4, 9kDa | 7p21.3 | [64] | • | ||||||||
Immune-related genes | [111] | • | ||||||||||
IL6 | Interleukin 6 | 7p15-21 | [1, 11, 119] | • | • | |||||||
IL12 | Interleukin 12 | 3q25 | [1, 11, 119] | • | • | |||||||
TNFa | Tumor necrosis factor | 6p21.3 | [1, 11, 119] | • | • | |||||||
Other genes | ||||||||||||
DPP6 | Dipeptidyl aminopeptidase-like protein 6 | 7p36.2 | [66] | • | ||||||||
KLHL32 | Kelch-like family member 32 | 6q16.1 | [64] | • | ||||||||
OFCC1 | Orofacial cleft 1 candidate 1 | 6p24.3 | [108] | • |
Whether the field focuses on the identification of rare mutations, common polymorphisms, gene expression changes, or all three, it is abundantly clear that large patient cohorts will be an essential requirement to identify relevant genes and to minimize the risk for false-positive findings. Over the last decade, the Tourette Syndrome Association International Consortium for Genetics (TSAICG), consisting of 14 different collaborative sites, has been working to collect larger samples of TS subjects. Using this collection and additional samples from other collaborating investigators, the TSAICG completed the first GWAS of TS [56], described above, and has recently undertaken a second TS GWAS in 3,000 cases and 5,000 ancestry-matched controls (http://www.findtsgenes.org/). The analyses of these data are nearing completion and should help identify common and rare genetic variants that increase the risk for TS. Once these genes are characterized, there is optimism that subsequent research will elucidate the biological processes that are important for the development of TS.
In 2011, a second consortium, the Tourette International Collaborative Genetics (TIC Genetics; http://tic-genetics.org/) study, was established, with the aims of collecting clinical data and biomaterials (DNA, transformed cell lines, RNA) from TS patients and their family members. Recruitment is from more than twenty sites in the USA, Europe, and South Korea, and these materials are part of a sharing repository of the National Institute for Mental Health (NIMH) Center for Collaborative Genetic Studies on Mental Disorders. Data and biomaterials will be made available to the widest possible research community to hasten the identification of causal genetic factors and facilitate better understanding and treatment of TS [130]. Both consortia, TSAICG and TIC Genetics, are currently working closely to plan complementary analyses and to combine existing data for analyses of larger TS data sets.
Finally, a large consortium of TS investigators in the European Union is conducting a parallel, longitudinal study of 1,000 TS subjects, with a plan to complete a third GWAS in the near future (http://www.emtics.eu/).
There is great hope that the efforts of these groups and their research will elucidate novel cellular and molecular mechanisms that may not have been previously hypothesized to be important for the etiology of TS. Recent discovery of rare mutations in the histamine neurotransmission pathway led to a current clinical trial of an H3-modulating compound, serving as one example of how such a process might proceed from gene discovery to the development of new intervention strategies. In the end, the continued generosity of patients and their families participating in research, the sharing of biomaterials across the scientific community, and the effective collaboration among scientists, patients, families, and research-funding institutions will all be critical to success. There is reason for great optimism that the pace of genetic discovery in TS will accelerate and inform intervention strategies that will alleviate suffering endured by those with TS and related disorders.
Acknowledgments
This work is supported by the Brain and Behavior Research Foundation (NARSAD) to GC, by the National Institute of Mental Health (NIMH) T32 Fellowship for postdoctoral training in childhood neuropsychiatric disorders, Award Number MH018268 to JBL, and by NIMH Award Number K08MH099424 to TVF.
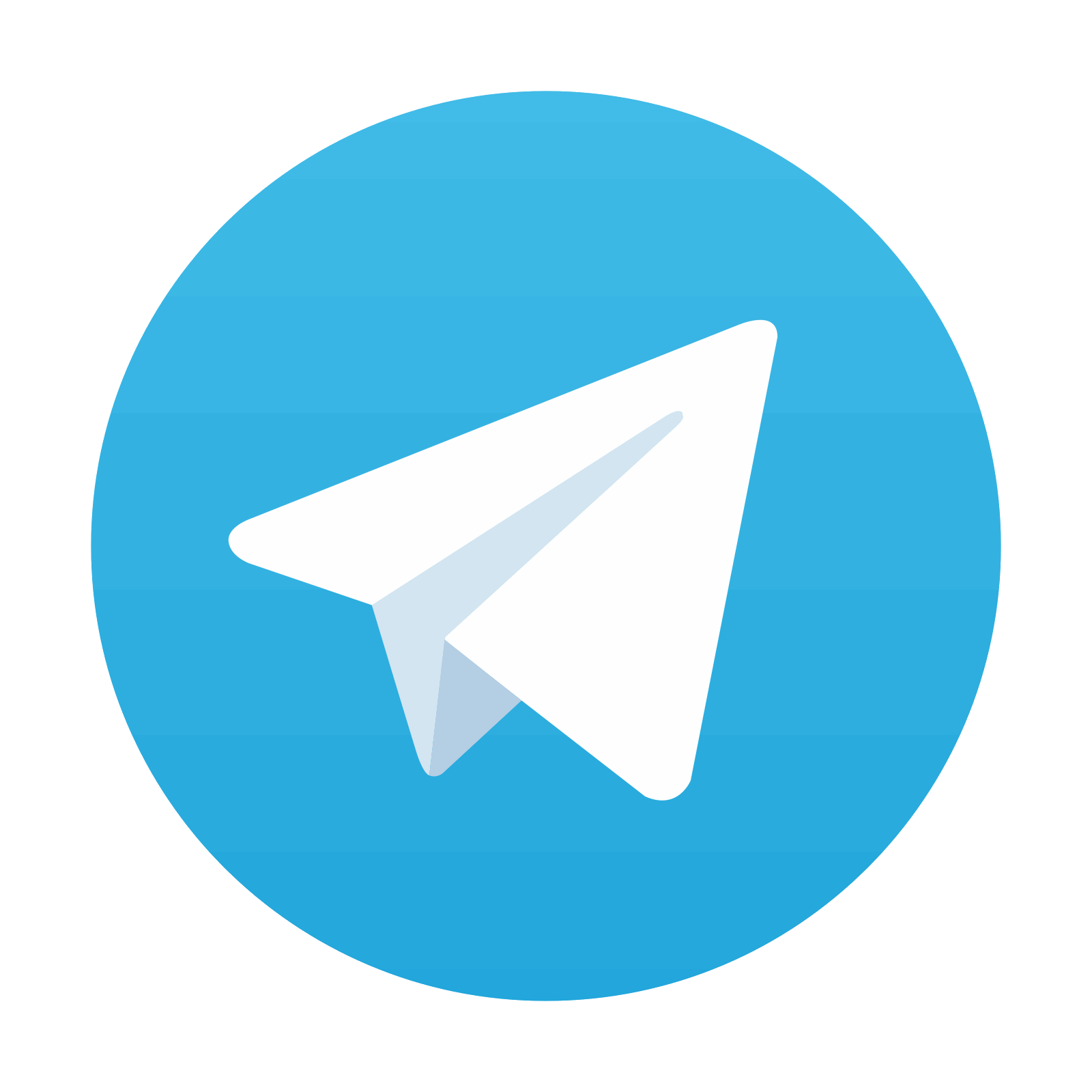
Stay updated, free articles. Join our Telegram channel

Full access? Get Clinical Tree
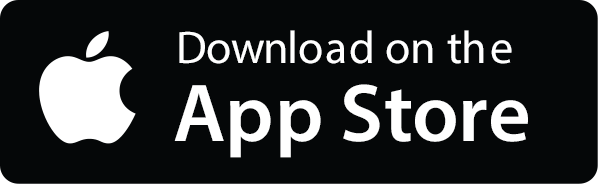
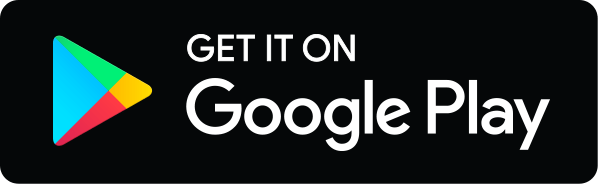