inheritance of nonspecific predisposing factors may act by increasing the chances that acquired epileptogenic factors will cause seizures or epilepsy even in the relatives of patients with acquired lesions, though this occurs to a much lesser degree than that observed in the relatives of patients with idiopathic epilepsy.
TABLE 20.1. Main mendelian disorders and chromosomal abnormalities associated with epilepsy | ||||||||||||||||||||||||||||||||||||||||||||||||||||||||||||||||||||||||||||||||||||||||||||||||
---|---|---|---|---|---|---|---|---|---|---|---|---|---|---|---|---|---|---|---|---|---|---|---|---|---|---|---|---|---|---|---|---|---|---|---|---|---|---|---|---|---|---|---|---|---|---|---|---|---|---|---|---|---|---|---|---|---|---|---|---|---|---|---|---|---|---|---|---|---|---|---|---|---|---|---|---|---|---|---|---|---|---|---|---|---|---|---|---|---|---|---|---|---|---|---|---|
|
epilepsy were also found to carry a higher risk than the offspring of parents with other types, not only for absence seizures but also for other seizure types in the study of Ottman et al. (1989).
TABLE 20.2. Genetic epilepsy syndromes with simple inheritance (gene unknown) | ||||||||||||||||||||||||||||||||||||||||||||||||||||||||||
---|---|---|---|---|---|---|---|---|---|---|---|---|---|---|---|---|---|---|---|---|---|---|---|---|---|---|---|---|---|---|---|---|---|---|---|---|---|---|---|---|---|---|---|---|---|---|---|---|---|---|---|---|---|---|---|---|---|---|
|
same genetic mechanisms of channel dysfunction known to be operative in the single gene epilepsies may be part of the cause in these cases as well.
TABLE 20.3. Known idiopathic epilepsy genes in 2003 | ||||||||||||||||||||||||||||||||||||||||||||||||||||||||||||||||||||||||||||||||||||||||||||||||||||||||||||||||||||||||||||||||||||||
---|---|---|---|---|---|---|---|---|---|---|---|---|---|---|---|---|---|---|---|---|---|---|---|---|---|---|---|---|---|---|---|---|---|---|---|---|---|---|---|---|---|---|---|---|---|---|---|---|---|---|---|---|---|---|---|---|---|---|---|---|---|---|---|---|---|---|---|---|---|---|---|---|---|---|---|---|---|---|---|---|---|---|---|---|---|---|---|---|---|---|---|---|---|---|---|---|---|---|---|---|---|---|---|---|---|---|---|---|---|---|---|---|---|---|---|---|---|---|---|---|---|---|---|---|---|---|---|---|---|---|---|---|---|---|
|
while loss-of-function mutations of one of these genes (SCN1A) usually causes a more severe phenotype. However, the phenotypes associated with missense mutations of these genes may sometimes be severe, including some cases with Dravet syndrome or a picture reminiscent of MAE. Genetic heterogeneity may result from either variable expression of some genes or allelic heterogeneity (Meisler et al., 2001).
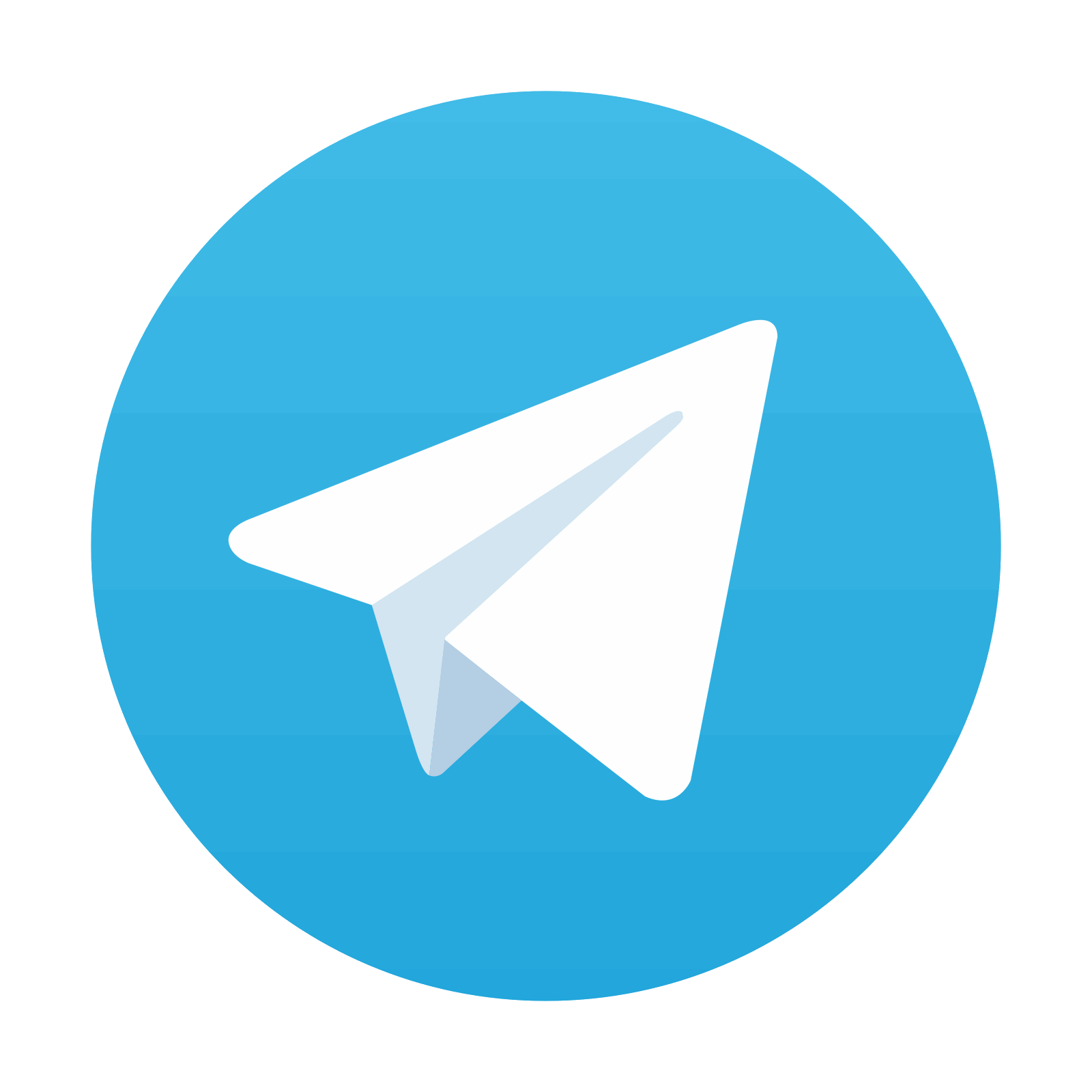
Stay updated, free articles. Join our Telegram channel

Full access? Get Clinical Tree
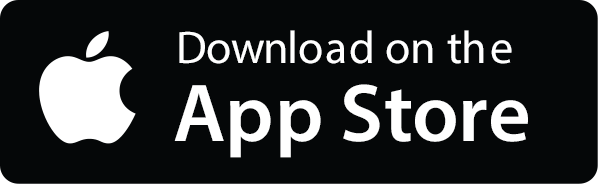
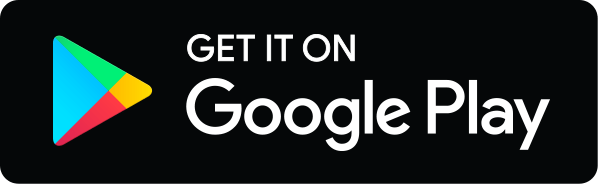