Glioma, especially high-grade glioblastoma multiforme (GBM), is the most common and aggressive type of brain tumor, accounting for about half of all the primary brain tumors. Despite continued advances in surgery, chemotherapy, and radiotherapy, the clinical outcomes remain dismal. The 2-year survival rate of GBM is less than 30%. Better understanding of GBM biology is needed to develop novel therapies. Recent studies have demonstrated the existence of a small subpopulation of cells with stemlike features called cancer stem cells (CSCs). These GBM CSCs are self renewable and highly tumorigenic. They not only are chemo-radio resistant but also often contain multidrug resistance genes and drug transporter genes. These characteristics enable GBM CSCs to survive standard cytotoxic therapies. Among GBM CSCs, CD133 + cells are a well-defined population and are prospectively isolated by their cell-surface marker. Increasing data show that the presence of CD133 + CSCs highly correlates with patient survival, making these cells an ideal immunotherapy target population. The authors have reviewed recent studies related with GBM CSCs (particularly CD133 + CSCs) and the novel therapeutic strategies targeting these cells.
Human brain tumors are a diverse group of diseases characterized by the abnormal growth of brain cells contained within the skull, afflicting adults and children. According to the National Cancer Institute data, there are about 20,000 new cases of brain tumor and 13,000 deaths each year in the United States. In children, brain tumors are the leading cause of solid tumor cancer death; all forms of glioma make up about one-fifth of all childhood cancers ( www.cancer.gov ). In adults, the most common malignant brain tumor, glioblastoma multiforme (GBM), is also the most malignant primary tumor of the brain associated with one of the worst 5-year survival rates among all human cancers. The median survival time is 14.6 months after first diagnosis. Despite the advances in conventional treatments, composed of surgical resection, local radiotherapy, and systemic chemotherapy, the incidence and mortality rates for gliomas have changed little in the past decade. With greater understanding of the cellular and molecular mechanisms of cancer initiation and propagation, the cancer stem cell (CSC) hypothesis presents new insights for developing novel treatments that target this group of cells. In this article, the authors discuss the CSC hypothesis and its application to develop treatments for glioma.
CSC brain tumor stem cell and CD133 CSCs
The first conclusive evidence for CSCs came from the studies of acute myeloid leukemia (AML).
Bonnet and Dick isolated a subpopulation of AML cells that were capable of initiating AML in immunodeficient NOD/SCID (nonobese diabetic/severe combined immunodeficient) mice. These leukemia cells (leukemia stem cells [LSCs]) express cell-surface markers that are similar to normal hematopoietic stem cells (HSCs). The AML that is established from these LSCs recapitulates the morphologic and immunophenotypic heterogeneity of the original tumor. These seminal studies opened the door for CSC study. Besides the properties shared with normal stem cells (self-renewal and the ability to differentiate into other cells), candidate cells must present the following properties to be considered as CSCs: (1) the unique ability to engraft, (2) the ability to recapitulate the tumor of origin morphologically and immunophenotypically in xenografts, and (3) the ability to be serially transplanted. These criteria are the standard to identify other CSCs not only in hematopoietic tumors but also in solid tumors.
The first solid tumor CSCs were identified from breast cancer by isolating CD44 + /CD24 −/low cells from primary tumor cells. The isolated CSCs can recapitulate the original breast cancer with the same morphologic and immunophenotypic features. CSCs could be isolated from these grafts and serially transplanted. For gliomas, several groups isolated brain tumor stem cells (BTSCs) from primary tumors based on the criteria mentioned earlier and the ability to form neurospheres as normal neural stem cells (NSCs) do. In the authors’ study, as few as 100 of these BTSCs could recapitulate the heterogeneity of GBM in immunocompromised rodents. In addition to primary gliomas, the authors also isolated cancer stemlike cells from the commercial rat gliosarcoma cell line, 9L. This cell line has been cultured in the laboratory over a long period under neurosphere conditions used for NSC expansion. Similar results were reported by Kondo and colleagues for the rat GBM cell line, C6. These data indicate that glioma cell lines may retain the capacity for a stemlike phenotype even after years of in vitro culture. CSCs have also been identified in various other malignant primary tumors and cancer cell lines by using different cell-surface markers ( Table 1 ).
Tumor | Type | Isolation Markers | References |
---|---|---|---|
AML | Primary tumors | CD34 + CD38 – | |
Breast | Primary tumors | CD44 + CD24 −/LOW | |
Brain | Primary tumors | CD133+ | |
Cell lines | CD133+ /sphere formation | ||
Cell lines | Side population (SP) | ||
Colon | Primary tumors | CD133+ | |
Primary tumors | CD133+ CD44+ | ||
Cell lines | CD133+ | ||
Laryngeal | Cell lines | CD133+ | |
Leukemia | Primary tumors | CD34+CD10- | |
Liver | Primary tumors/cell line/blood | CD90+CD44+ | |
Cell lines | CD133+ | ||
Lung | Primary tumors | ALDH1 | |
Primary tumors | CD133+ | ||
Melanoma | Primary tumors | ABCB5+ | |
Primary tumors | CD133+ ABCG2+ | ||
Ovarian | Primary tumors | CD133+ | |
Pancreas | Primary tumors | CD133+ | |
Cell lines | CD133+ | ||
Prostate | Primary tumors | CD133+ |
Among the CSC-associated markers, CD133 (prominin-1) is one of the most important and well studied. It is a 120 kDa, 5-transmembrane-domain glycoprotein, with 2 cytoplasmic loops, 2 glycosylated extracellular domains, and a cytoplasmic C-terminal domain. Despite mounting evidence that CD133 is an important marker for somatic stem cells and CSCs, its physiologic function is not known. Some studies suggested that CD133 is involved in neural-retinal development and phototransduction. Due to its interaction with plasma membrane cholesterol and enrichment in cholesterol-based membrane microdomains, it may play some role in membrane topology. Barcelos and colleagues also demonstrated that CD133 + progenitor cells could promote the healing of diabetic ischemic ulcer through stimulating angiogenesis and activating the Wnt pathway. This observation may suggest a role for CD133 + CSCs in tumor angiogenesis and in related signaling pathways.
Glioma CSCs and clinical treatment
CSCs are often resistant to conventional chemotherapy and radiation therapy. Glioma CSCs are resistant to radiotherapy and chemotherapy. CD133 + glioma CSCs could preferentially activate the DNA damage checkpoint response under irradiation. The activation is Chk1 and Chk2 checkpoint kinase dependent. Blazek and colleagues also confirmed that CD133 + glioma cells are more radiation resistant than CD133 − cells. This study also reported that CD133 expression is upregulated 1.6 fold under 2% O 2 (hypoxic conditions). Similar results had been reported by other groups also. Because hypoxic conditions exist in most solid tumors, including gliomas, this upregulation of CD133 expression provides enhancement for specific targeting of glioma CSCs rather than NSCs.
An in vitro study showed that CD133 + glioblastoma CSCs are more resistant to multiple chemotherapeutic agents than their CD133 − counterparts. The authors demonstrated that CD133 + glioma CSCs express higher levels of the drug transporter gene, BCRP , combined with upregulation of the DNA repair protein, methylguanine DNA methyltransferase (MGMT) mRNA, and mRNAs of other genes that inhibit apoptosis, including FLIP, Bcl-2, Bcl-X , and some IAP family genes. These cells were significantly resistant to chemotherapeutic agents when compared with autologous CD133 − cells.
Glioma CSCs possess migration as an additional property to escape from conventional therapies. The authors and others reported that overexpression of chemokine receptors, such as CXCR4, is a common mechanism related to CSC migration. As reviewed by Lefranc and colleagues, glioma cell migration is a complex combination of multiple molecular processes, including the alteration of tumor cell adhesion to a modified extracellular matrix, the secretion of proteases by the cells, and modifications to the actin cytoskeleton. Intracellular signaling pathways involved in the acquisition of resistance to apoptosis by migrating glioma cells include PI3K, Akt, mTOR, NF-κB, and autophagy (programmed cell death type II).
Glioma CSCs and clinical treatment
CSCs are often resistant to conventional chemotherapy and radiation therapy. Glioma CSCs are resistant to radiotherapy and chemotherapy. CD133 + glioma CSCs could preferentially activate the DNA damage checkpoint response under irradiation. The activation is Chk1 and Chk2 checkpoint kinase dependent. Blazek and colleagues also confirmed that CD133 + glioma cells are more radiation resistant than CD133 − cells. This study also reported that CD133 expression is upregulated 1.6 fold under 2% O 2 (hypoxic conditions). Similar results had been reported by other groups also. Because hypoxic conditions exist in most solid tumors, including gliomas, this upregulation of CD133 expression provides enhancement for specific targeting of glioma CSCs rather than NSCs.
An in vitro study showed that CD133 + glioblastoma CSCs are more resistant to multiple chemotherapeutic agents than their CD133 − counterparts. The authors demonstrated that CD133 + glioma CSCs express higher levels of the drug transporter gene, BCRP , combined with upregulation of the DNA repair protein, methylguanine DNA methyltransferase (MGMT) mRNA, and mRNAs of other genes that inhibit apoptosis, including FLIP, Bcl-2, Bcl-X , and some IAP family genes. These cells were significantly resistant to chemotherapeutic agents when compared with autologous CD133 − cells.
Glioma CSCs possess migration as an additional property to escape from conventional therapies. The authors and others reported that overexpression of chemokine receptors, such as CXCR4, is a common mechanism related to CSC migration. As reviewed by Lefranc and colleagues, glioma cell migration is a complex combination of multiple molecular processes, including the alteration of tumor cell adhesion to a modified extracellular matrix, the secretion of proteases by the cells, and modifications to the actin cytoskeleton. Intracellular signaling pathways involved in the acquisition of resistance to apoptosis by migrating glioma cells include PI3K, Akt, mTOR, NF-κB, and autophagy (programmed cell death type II).
Targeting signaling pathways in CSCs
Signaling pathways, including Wnt, hedgehog, Notch, HOX family members, Bmi-1, phosphatase and tensin (PTEN) homolog, telomerase, and efflux transporters, are involved in balancing self-renewal and differentiation of NSCs and CSCs. Newer studies also show that Notch, hedgehog, and bone morphogenic protein (BMP) pathways are involved in controlling CD133 + CSC functions in glioma. Bao and colleagues showed that glioma CSCs generate vascular tumors through overexpression of vascular endothelial growth factor (VEGF). Because VEGF is a validated therapeutic target for glioma therapy, this finding may indicate more favorable targeting of CSCs in glioma therapy.
Due to the common pathways and cell-surface markers shared by NSCs and CSCs, it is important to develop CSC-specific therapies that avoid potential toxicities to NSCs. Selective targeting of AML CSCs by Guzman and colleagues demonstrated the possibility of such selectivity. They showed that LSCs, but not normal HSCs, were susceptible to the apoptotic effects of the proteasome inhibitor MG-132 combined with the anthracycline idarubicin through NF-κB activity. NF-κB inhibitors could induce LSC apoptosis but spare normal HSCs. In a subsequent study, the same group also showed that 4-benzyl-2-methyl-1,2,4-thiadiazolidine-3,5-dione (TDZD-8) treatment could induce oxidative stress and selectively kill LSCs in vitro but not HSCs. Other studies demonstrated that AML is PTEN pathway dependent. Rapamycin, a PI3K/PTEN signaling pathway inhibitor, could dramatically decrease leukemia burden. In addition, this treatment appeared to be specific for the LSCs because normal HSCs were unaffected.
When selective targeting of CSCs becomes possible, another strategy to target CSCs is by forcing them to differentiate and become more sensitive to conventional chemo-radiotherapies. Differentiation therapy is based on this concept, and several agents had been tested in recent years. All-trans retinoic acid (ATRA) is the most studied differentiation therapy molecule. Sell reported that about 90% of newly diagnosed patients with acute promyelocytic leukemia achieved complete remission and more than 70% were cured by ATRA therapy. Differentiation with ATRA was also reported in early-stage mouse embryonic stem cells, rat C6 glioma cells, and human embryonic NSCs. These studies opened up the possibility of using ATRA to induce differentiation of glioma CSCs as a therapeutic technique. Besides ATRA, other agents have also been tested for this approach of differentiation therapy. Piccirillo and colleagues have shown that treating CSCs with differentiation factors can effectively deplete CSCs in human glioma. In this study, researchers reported that BMPs, especially BMP4, activate BMP receptors and trigger the Smad signaling cascade in cells isolated from human glioblastomas. This activated signaling pathway leads to a reduction in proliferation and increased expression of differentiated neural markers in both CD133 + CSCs and normal glioma cells. When xenotransplanted BMP4–pretreated glioma CSCs were transplanted into mice, invasive glioma was not detected. These data provided evidence that differentiation therapy is a promising noncytotoxic strategy to deplete CSCs.
Targeting CSCs using passive immunotherapy
Antibody therapy (passive immunotherapy) directed against CSCs has resulted in several experimental therapeutic successes. Schatton and colleagues identified melanoma CSCs with the expression of the chemoresistance mediator ABCB5+ (ATP binding cassette B5+). Treatment with anti-ABCB5 antibody for xenografted melanomas resulted in significant reduction of tumor size. Moreover, this direct targeting of the CSC antigen induced tumor cell death through antibody-dependent cell-mediated cytotoxicity. Another encouraging result of antibody therapy was reported by Jin and colleagues. In their study, CD44 had been identified as an AML CSC surface marker. Although the same marker is expressed on normal bone marrow HSCs at a lower level, treatment with anti-CD44 antibody before transplant can selectively block engraftment of AML LSCs but not normal HSCs. Treatment of previously engrafted AML LSCs with the same antibody led to a significant reduction in disease burden by 83% to 100%. In vivo treated AML CSCs resulted in lower engraftment, suggesting that anti-CD44 antibody treatment directly altered the fate of CSCs either by inducing differentiation or by inhibiting their repopulation ability. This study provided evidence that passive immunotherapy with antibodies targeting CSC antigens could be effective even when the same antigen is shared with NSCs. Krause and colleagues also reported that the expression of CD44 is required on leukemic cells that initiate chronic myeloid leukemia (CML). Anti-CD44 antibody treatment attenuated the induction of CML-like leukemia in recipients, suggesting that CD44 blockade may be beneficial in autologous transplantation in CML.
Passive immunotherapy targeting solid tumor CSCs has also been reported. Smith and colleagues demonstrated that antibody-drug conjugates (ADCs) could be used for both hepatocellular and gastric cancers. When an anti-CD133 antibody was conjugated to a potent cytotoxic drug, monomethyl auristatin F, this conjugate effectively inhibited the growth of Hep3B hepatocellular and KATO III gastric cancer cells in vitro by inducing apoptosis in CD133 + CSCs. In vivo administration of this ADC also resulted in significant delay of tumor growth in SCID mice.
In addition to directly targeting CSC surface antigens, antibody therapy has also been used as sensitizing agents combined with chemotherapy. Todaro and colleagues showed that treatment of CD133 + colon CSCs with anti-IL-4 antibody before treatment with oxaliplatin, 5-FU (fluorouracil), or TRAIL (tumor necrosis factor–related apoptosis-inducing ligand) resulted in increased cell death. In vivo injection of IL-4 neutralizing antibodies followed by oxiplatin effectively reduced tumor burden.
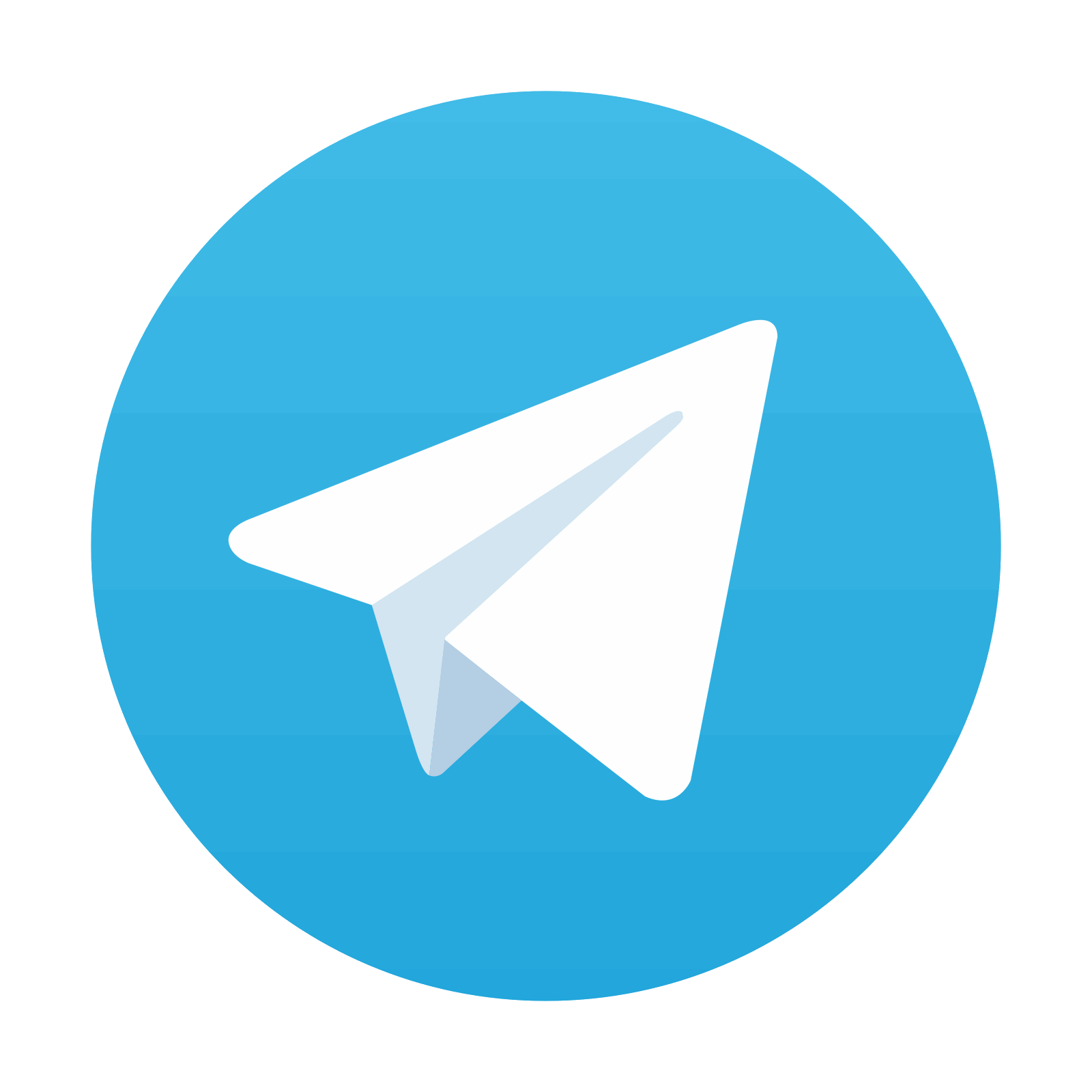
Stay updated, free articles. Join our Telegram channel

Full access? Get Clinical Tree
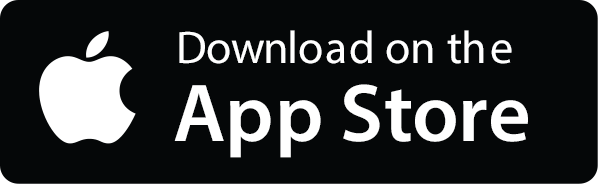
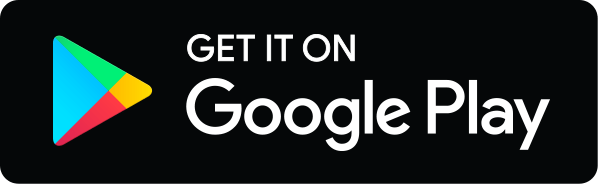