Where Me is a divalent metal, XO4 a trivalent anion and Y a monovalent anion. Stoichiometric hydroxyapatite is therefore represented by the formula Ca10(PO4)6(OH)2. The stoichiometric HA is characterized by its Ca/P ratio of 1.67. Its infrared spectroscopic analysis shows characteristic bands allocated to OH− ions and phosphate group; and its X-ray diffraction diagram of X corresponds to a hexagonal structure (P63/m group) with the lattice parameters: a = 0.9422 nm and c = 0.688 nm [48].
The mesh contains ten calcium atoms and six PO4 3− tetrahedra and two hydroxyl groups. The assembly of PO4 3− ions in the form of honeycomb which constitutes the backbone of the network and provides a great stability to the structure of hydroxyapatite. There in the house of the mesh type two tunnel, one with a diameter of about 2.5 Å and the other between 3 Å and 4.5A. These tunnels confer hydroxyapatite ion exchange properties and acceptor small molecules (O2, H2O, glycine …).
The synthesis of hydroxyapatite can be done in two ways, either by precipitation in an aqueous medium in the presence of ammonia to adjust pH or by thermal reaction at high temperature.
β -tricalcium phosphate (β-TCP )
β -tricalcium phosphate has the chemical formula Ca3(PO4)2. It is characterized by a Ca / P ratio of 1.5. It is rhombohedral structure (R3c group) and has the lattice parameters a = b = 1.0439 nm and c = 3.7375 (for multiple hexagonal mesh).
Its preparation is made by sintering at high temperature deficient apatite calcium Ca/P ratio of 1.5 or by solid-solid reaction at high temperatures. Sintering temperatures are usually between 1000 and 1250 ° C [49].
There is another way of implementing the TCP wet. The principle is to obtain a precipitate poorly crystallized using a phosphate source and a source of calcium while controlling the temperature and pH. The whole is then calcined at high temperature.
Forming and Shaping
In order to fabricate bioceramics in progressively complex shapes, scientists are investigating the use of both old and new manufacturing techniques. These techniques range from an adaptation of the age-old pottery techniques to the newest manufacturing methods for high-temperature ceramic parts for airplane engines. Namely, reverse engineering [50, 51] and rapid prototyping [52–54] technologies have revolutionized a generation of physical models, allowing the engineers to efficiently and accurately produce physical models and customized implants with high levels of geometric intricacy. Combined with the computer-aided design and manufacturing (CAD/CAM), complex physical objects of the anatomical structure can be fabricated in a variety of shapes and sizes. In a typical application, an image of a bone defect in a patient can be taken and used to develop a three-dimensional (3D) CAD computer model [55–58]. Then a computer can reduce the model to slices or layers. Afterwards, 3D objects and coatings are constructed layer-by-layer using rapid prototyping techniques. A custom-made implant of actual dimensions would reduce the time it takes to perform the medical implantation procedure and subsequently lower the risk to the patient. Another advantage of a pre-fabricated, exact-fitting implant is that it can be used more effectively and applied directly to the damaged site rather than a replacement, which is formulated during surgery from a paste or granular material (Fig. 15.1).
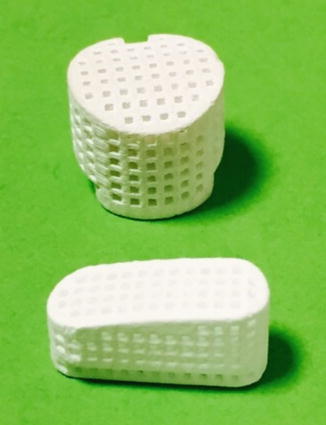
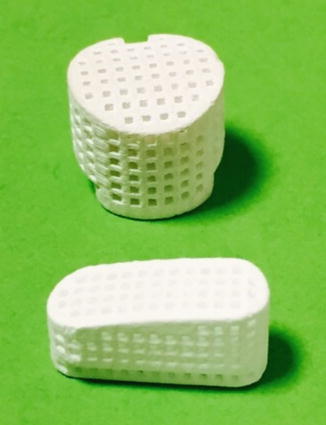
Fig. 15.1
Example of calcium phosphate ceramic obtains by rapid prototype technique
In addition to the aforementioned modern techniques, classical forming and shaping approaches are still widely used. The selection of the desired technique depends greatly on the ultimate application of the bioceramic device, e.g., whether it is for a hard-tissue replacement or an integration of the device within the surrounding tissues. In general, three types of the processing technologies might be used:
Employment of a lubricant and a liquid binder with ceramic powders for shaping by impregnation of polymeric foam and subsequent firing;
Application of self-setting and self-hardening properties of water-wet molded powders with or without porogen agent;
Materials are melted to form a liquid and are shaped during cooling and solidification.
Sintering and Firing
A sintering (or firing ) procedure appears to be of a great importance to manufacture bulk bioceramics with the required mechanical properties. Usually, this stage is carried out according to controlled temperature programs of electric furnaces in air. The firing step can include temporary holds at intermediate temperatures to burn out organic binders [59]. The heating rate, sintering temperature and holding time depend on the starting materials. For example, in the case of HA, these values are in the ranges of 0.5–3 °C/min, 1000–1250 °C and 2–5 h, respectively [60]. In the majority cases, sintering allows a structure to retain its shape. However, this process might be accompanied by a considerable degree of shrinkage, which must be accommodated in the fabrication process.
In general, sintering occurs only when the driving force is sufficiently high, while the latter relates to the decrease in surface and interfacial energies of the system by matter (molecules, atoms or ions) transport, which can proceed by solid, liquid or gaseous phase diffusion. Namely, when solids are heated to high temperatures, their constituents are driven to move to fill up pores and open channels between the grains of powders, as well as to compensate for the surface energy differences among their convex and concave surfaces (matter moves from convex to concave). At the initial stages, bottlenecks are formed and grow among the particles. Existing vacancies tend to flow away from the surfaces of sharply curved necks; this is an equivalent of a material flow towards the necks, which grow as the voids shrink. Small contact areas among the particles expand and, at the same time, a density of the compact increases and the total void volume decreases. As the pores and open channels are closed during a heat treatment, the particles become tightly bonded together and density, strength and fatigue resistance of the sintered object improve greatly. Grain-boundary diffusion was identified as the dominant mechanism for densification. Furthermore, strong chemical bonds are formed among the particles and loosely compacted green bodies are hardened to denser materials.
In the case of calcium orthophosphates, the earliest paper on their sintering was published in 1971 [61]. Since then, numerous papers on this subject were published and several specific processes were found to occur during calcium orthophosphate sintering. Firstly, moisture, carbonates and all other volatile chemicals remaining from the synthesis stage, such as ammonia, nitrates and any organic compounds, are removed as gaseous products. Secondly, unless powders are sintered, the removal of these gases facilitates production of denser ceramics with subsequent shrinkage of the samples. Thirdly, all chemical changes are accompanied by a concurrent increase in crystal size and a decrease in the specific surface area. Fourthly, a chemical decomposition of all acidic orthophosphates and their transformation into other phosphates (e.g., 2HPO4 2− → P2O7 4− + H2O↑) takes place. Besides, sintering causes toughening, densification, partial dehydroxylation (in the case of HA) [32], grain growth, as well as it increases the mechanical strength.
Bone Substitute Characteristics
For their use in clinical, bone substitutes must meet certain criteria. These characteristics define the efficiency of filling material.
Biocompatibility
The biocompatibility of substitute employees is very important. These materials and their degradation products must not, in fact, present cytotoxicity or be accompanied by a strong inflammatory reaction.
By their nature, calcium phosphate materials and their degradation products (calcium phosphate) are perfectly assimilated by the body and even participate in bone regeneration. Either in vitro or in vivo, most of these materials exhibit a very good biocompatibility. The implantation of HA ceramic, of β-TCP or BCP in animals is accompanied by a good bone apposition to the surface of the materials, without the presence of inflammatory response [62]. In rare cases, excessive dissolution of materials is accompanied by cytotoxicity and consequently a lack of efficacy. Such observations have been made for ceramic of α-TCP [63].
Osteoconduction
The osteoconduction is currently defined as the ability of a material to permit bone growth when in contact or near a bone [62]. The material must be able to accommodate the osteoblast precursors that migrate from the bone marrow and ensure their proliferation and differentiation into osteoblasts.
Calcium phosphate ceramics have very good osteoconductive properties and their capacity is determined by characteristics including their chemical composition and macroporosity. Implantation in BCP ceramic dog femurs (HA / β-TCP: 60/40) shows an earlier osseointegration and a quantity of new bone than all time of the study compared to samples HA (similar porosity) [64].
Wilson et al. [65] have also studied in combination the effects of composition and the macroporosity of the osteoconductive potential of calcium phosphate ceramics. They compared bone formation after implantation in the lumbar spine of samples goat β-TCP, HA and BCP (HA / β-TCP: 80/20) which were sintered at different temperatures, and therefore having different macropores. They then classified the samples to their potential osteoconductive: BCP sintered at low and medium temperature (rough and smooth) = β-TCP > HA sintered at low temperature > PCO sintered at high temperature (rough and smooth) > HA sintered high temperature. In view of the results, they indicate that the parameter that determines the potential bioactivity would osteoconductive ceramics related to their dissolution rate.
In their study, Habibovic et al. [66] have obtained comparable results after implantation site in orthotopic various calcium phosphates: HA, carbonated apatite ceramics and BCP lacking macropores induce little bone formation while it is very important for BCP macroporous samples.
Bioactivity
Bioactive materials have the unique property to create a direct contact with the bone without fibrous cap formation thereby increasing their potential for integration. This ability is the ability for these biomaterials to precipitate to their surface a nanocrystalline apatite similar to bone mineral, in contact with biological fluids [67]. The formation of the apatite phase is done in several steps: (1) existence of a supersaturated environment in calcium and phosphate ions; (2) precipitation in the material surface of a nanocrystalline carbonated apatite; (3) organization and incorporation of this phase with the organic matrix of the newly formed bone. The first step is carried out naturally and biological fluids in contact with an implant are supersaturated with respect to apatite nanocrystalline likely to form. It is considered that the ionic product is close to the serum phosphate solubility product octacalcium (OCP) [68]. The second step depends on the surface nucleating properties, it is particularly important for hydroxyapatite and other calcium phosphates may promote the epitaxial growth of apatite crystals. However, the ability of a surface to promote the germination of an apatite phase may be enhanced by the release of inorganic ions of the biomaterial itself.
Two other ions may contribute to this effect, phosphates and OH− ions by their effect on the local pH, and some biomaterials also use these properties. The third step is more complex and involves many physicochemical and biological parameters. Among the physical-chemical parameters, it should be mentioned the speed of crystal growth, the inhibition of growth and germination by proteins or ions (Mg, carbonates, pyrophosphates, citrates …), the distribution of these groups to the mineralization front.
The biological parameters concern, including adhesion, proliferation, differentiation and mineralization of osteoblasts, which depend strongly on the surface of the biomaterial and certain mineral ions in solution (particularly calcium and phosphate). As bone mineral, apatite nanocrystalline new formed phase is very reactive and it has significant capacity ion exchange and adsorption of proteins. It has also been suggested that proteins from surrounding fluids may be co-precipitated and thus included in this apatite layer during its formation. The materials of bioactivity was shown for the first time by Hench et al. [69] who noticed the formation of the apatite layer on their bioglass.
The presence of nucleation sites and an increase of the supersaturation of calcium and / or phosphate on the surface of the material are two mechanisms to induce the formation of the apatite similar to bone mineral [70]. Most ceramics based calcium phosphates are bioactive in varying degrees. The macroporosity of these materials appears to play an important role in this phenomenon, this macroporosity increases the surface area of the samples and therefore the number of nucleation sites. It has, on the other hand, been suggested that it may allow to obtain a micro environment for ion supersaturation and calcium phosphate [70].
Biodegradation
Shortly after implantation, a healing process is initiated by compositional changes of the surrounding bio-fluids and adsorption of biomolecules. Following this, various types of cells reach the bioceramic surface and the adsorbed layer dictates the ways the cells respond. Further, a biodegradation of the implanted bioceramics begins. This process can occur by either physicochemical dissolution with a possibility of phase transformations or cellular activity (so called, bioresorption). More likely, a combination of both processes takes place in vivo. Since the existing calcium orthophosphates are differentiated by Ca/P ratio, basicity/acidity and solubility their degradation kinetics and mechanism depend on the chosen type of calcium orthophosphate [71, 72]. Since dissolution is a physical chemistry process, it is controlled by some factors, such as solubility, surface area to volume ratio, local acidity, fluid convection and temperature.
With a few exceptions, dissolution rates of calcium orthophosphates are inversely proportional to the Ca/P ratio (except of TTCP), phase purity and crystalline size, as well as it is directly related to both the porosity and the surface area. Bioresorption is a biological process mediated by cells (mainly, osteoclasts and, in a lesser extent, macrophages). It depends on the response of cells to their environment. Osteoclasts attach firmly to the implant and dissolve calcium orthophosphates by secreting an enzyme carbonic anhydrase or any other acid, leading to a local pH drop to ~4–5 [73]. In any case, in vivo biodegradation of calcium orthophosphates is a complicated combination of various non-equilibrium processes, occurring simultaneously and/or in competition with each other (Fig. 15.2).
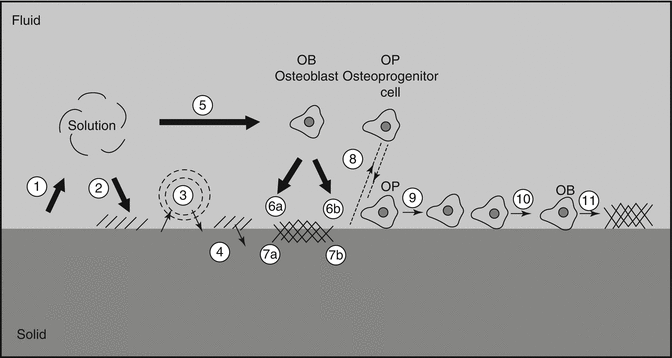
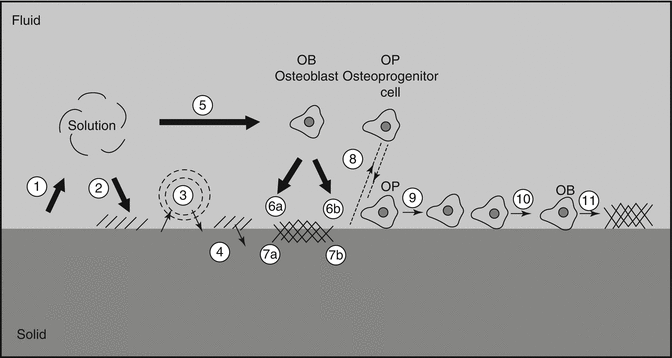
Fig. 15.2
A schematic diagram representing the events, which take place at the interface between bioceramics and the surrounding biological environment: (1) dissolution of bioceramics; (2) precipitation from solution onto bioceramics; (3) ion exchange and structural rearrangement at the bioceramic/tissue interface; (4) interdiffusion from the surface boundary layer into the bioceramics; (5) solution-mediated effects on cellular activity; (6) deposition of either the mineral phase (a) or the organic phase (b) without integration into the bioceramic surface; (7) deposition with integration into the bioceramics; (8) chemotaxis to the bioceramic surface; (9) cell attachment and proliferation; (10) cell differentiation; (11) extracellular matrix formation. All phenomena, collectively, lead to the gradual incorporation of a bioceramic implant into developing bone tissue (Reprinted with permission from Ducheyne and Qiu [23])
The experimental results demonstrated that both the dissolution kinetics and in vivo biodegradation of biologically relevant calcium orthophosphates proceed in the following decreasing order: β-TCP > bovine bone apatite (unsintered) > bovine bone apatite (sintered) > coralline HA > HA. In the case of biphasic (HA + TCP), triphasic and multiphasic calcium orthophosphates, the biodegradation kinetics depends on the HA/TCP ratio: the higher the ratio, the lower the degradation rate. Similarly, in vivo degradation rate of biphasic TCP (α-TCP + β-TCP) bioceramics appeared to be lower than that of α-TCP and higher than that of β-TCP bioceramics , respectively (Fig. 15.3).
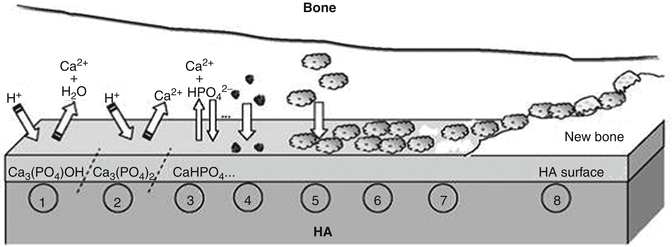
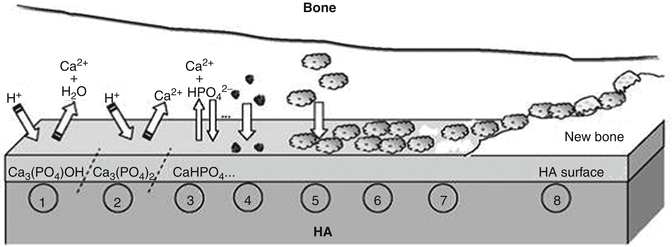
Fig. 15.3
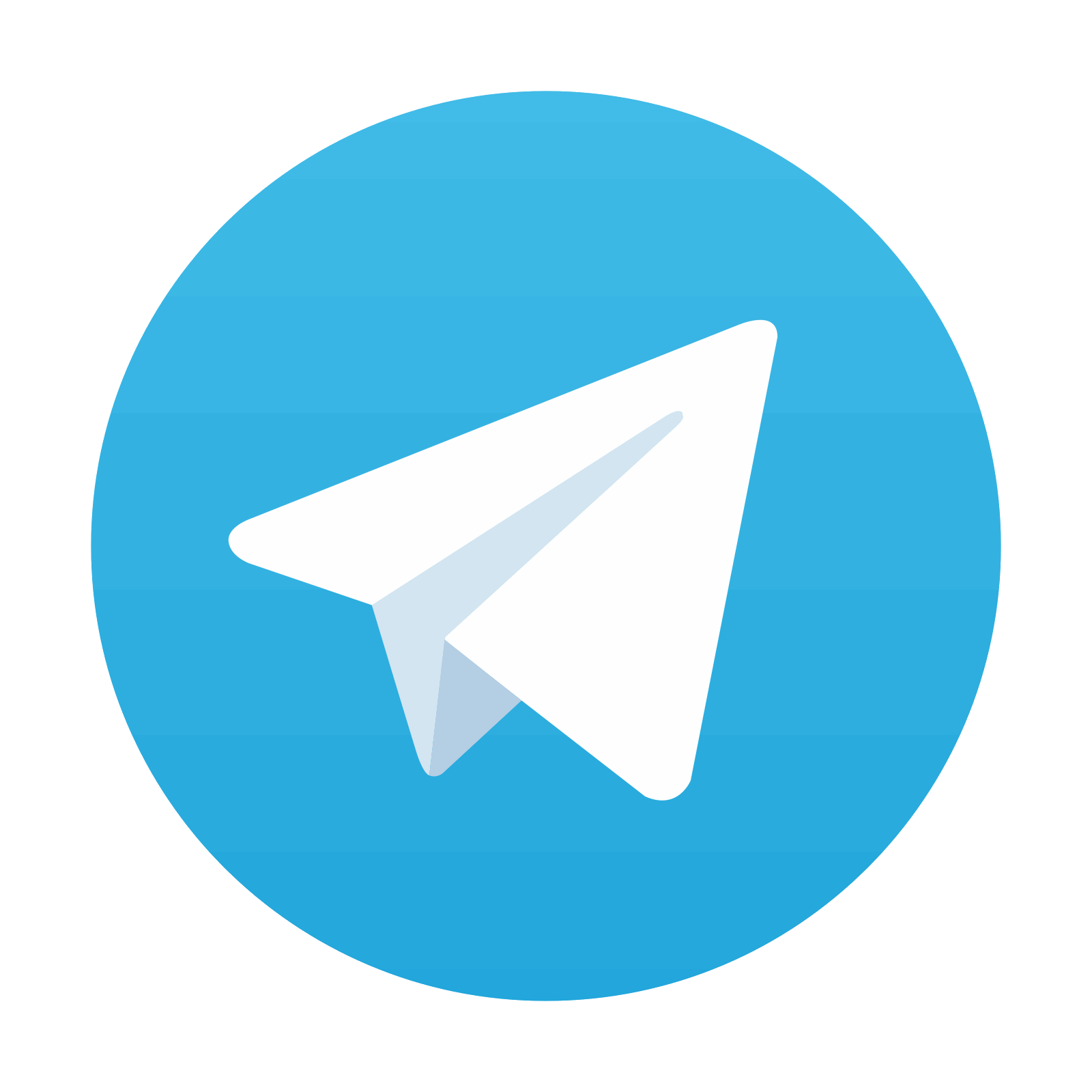
A schematic diagram representing the phenomena that occur on HA surface after implantation: 1 beginning of the implant procedure, where a solubilization of the HA surface starts; 2 continuation of the solubilization of the HA surface; 3 the equilibrium between the physiological solutions and the modified surface of HA has been achieved (changes in the surface composition of HA does not mean that a new phase of DCPA or DCPD forms on the surface); 4 adsorption of proteins and/or other bioorganic compounds; 5 cell adhesion; 6 cell proliferation; 7 beginning of a new bone formation; 8 new bone has been formed (Reprinted with permission from Bertazzo et al. [74])
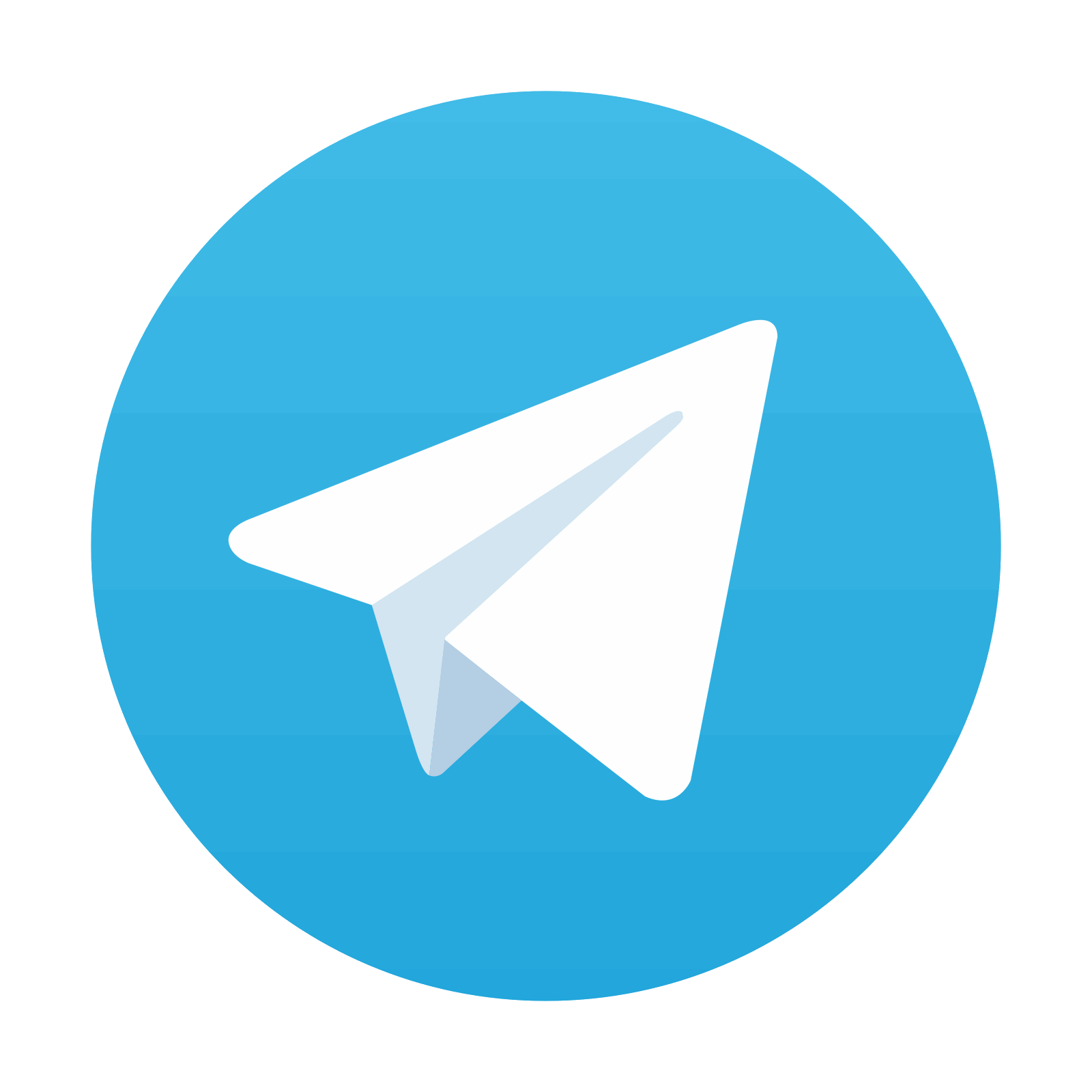
Stay updated, free articles. Join our Telegram channel

Full access? Get Clinical Tree
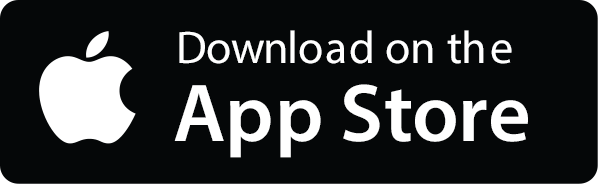
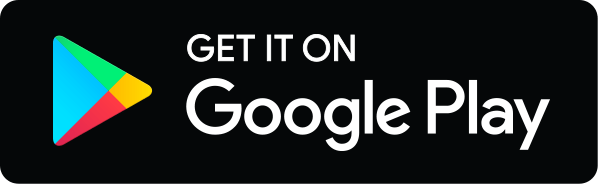
