Fig. 14.1
MIT Manus, one of the earliest robotic upper limb rehabilitation systems (Figure reproduced from [35] under the Creative Commons Attribution License)
One of the earliest robotic upper limb rehabilitation systems developed is the MIT-Manus [14], shown in Fig. 14.1. The MIT-Manus can operate in several planar reaching modes: assisting users, passively sensing motions, or by responding to the user’s motions. The results of several independent clinical trials showed that robotic training with the MIT-Manus helped 372 persons with stroke to improve upper extremity motor function [36]. Similarly, the Assisted Rehabilitation and Measurement Guide is an active-assistive robotic device that also showed the benefit of robotic training for people with a stroke [37]. Force feedback devices have also been used to quantify the performance of a patient for use in measuring improvement and possibly prescribing treatments [10]. However, recent review papers have stated that it is unclear whether robotic methods have the potential to produce greater benefits than conventional techniques when practiced for the same amount of time [12, 13]. The amount of training is one of the most important factors for functional recovery after stroke [1, 38, 39].
Robot guided therapy can either use assistive or resistive methods and it is not currently clear which is more effective. In active assistance training, a therapist or robot assists the patient through the desired motion. The benefits of active assistance include stretching the muscles and connective tissues, reinforcing a normal pattern of motion, and allowing the patient to practice more complex tasks [13]. Active assistance also allows for an increase in the intensity of training, since with assistance, more motions may be completed in less time. However, the “guidance hypothesis” suggests that motor learning could actually be decreased when the individual is physically guided since the individual learns how to interact with the therapist or device and not necessarily how to generally move their arm [13, 40]. It is possible that the patient is subconsciously only performing a portion of the task and not learning the other necessary muscle activations [41].
Resistive training methods work to facilitate rehabilitation by making task completion more difficult during training by applying forces that resist or perturb the motion. Individuals moving in a force field that perturbs their motion will adapt to generate forces that counteract the field, resulting in a normal motion within the field [1]. The adaptation will persist for a short time after the field is removed. This after-effect has led to error enhancement training, in which the errors that an individual makes during a motion are exaggerated. Once the disturbance is removed, the after-effect results in a more correct motion, however the corrected motion typically only persists for a short amount of time [42].
One reason the after-effects only last a short amount of time is the dual-rate learning process [43, 44]. The motor output is the summation of a fast learning process, which adapts quickly to new patterns and also forgets quickly, and a slow learning process which adapts over many repeated interactions, but shows prolonged after-effects. The after-effects generated as a result of resistive training are largely due to the slow learning process, but the quick fall off of the effects is the fast learning process adapting quickly. To modify the generated motions permanently, the slow learning process must be involved repeatedly. However, simply making the training sessions longer on each day does not increase the effectiveness since time is needed between sessions to allow for motor consolidation [45, 46]. Longer sessions several times a week are unlikely to make a significant difference, but shorter sessions practiced everyday are likely to be most effective. However, this has not been confirmed since most of the rehabilitation studies train for only 3 days a week. It is costly and time consuming to bring a person in everyday for the study and for long-term rehabilitation. Thirty minutes performed in the home each day would not be so difficult to perform and would involve less overall time spent training each week, particularly if transportation time is included.
There are several challenges that need to be overcome before these robotic methods can be used in the home. Cost is a significant issue that will be helped partially by economies of scale and decreasing cost of parts, but these costs will only come down so far. The methods need to be reevaluated to determine the most fundamental type of rehabilitation that can be used to generate the result. As opposed to developing more complicated and expensive robots that only incrementally improve the rehabilitation, some of the effort should focus on finding the methods that provide 90 % of the benefit at 10 % of the cost. The less complicated devices could also use less powerful motors, which would inherently provide a safer environment for rehabilitation; safety is critical to a home-based solution.
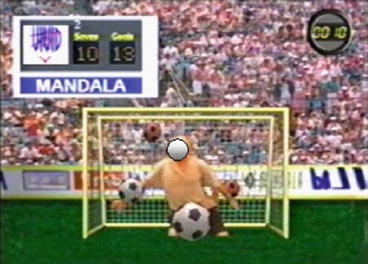
Fig. 14.2
An example of a rehabilitation game to encourage movements that are appropriate for motor relearning (Figure reproduced from [48] under the Creative Commons Attribution License)
14.4 Visual and Haptic Feedback
One major problem of many rehabilitation programs is the transference of learning between tasks, specifically from the rehabilitation therapy to activities of daily living. The use of visual and haptic feedback has been studied to overcome this problem. One study examined the effects of displaying patient motion collected from an electromagnetic motion capture system during training sessions and showed significant gains after 15 one-hour sessions [47], however no control subjects were used for comparison. In video capture virtual reality, the mirror images of patients’ motions have been displayed interacting in a virtual environment. These systems have been tested in a large number of studies, but have focused on presence and enjoyment rather than rehabilitation outcomes [48]. One example is shown in Fig. 14.2. The enjoyment is an important aspect of home-based rehabilitation as it directly relates to motivation and the likelihood that an individual will continue with the training. Virtual and augmented reality for use in rehabilitation has been a popular subject of recent research, however further study is needed to determine the long-term efficacy of virtual reality in rehabilitation [49, 50].
Haptic force feedback through a Phantom Omni [51] and a Rutgers Master II glove [52] have also been shown to help transfer stroke patient rehabilitation improvements to activities of daily living. The use of exoskeletons for force feedback providing a control force to the palm of the user’s hand [53] as well as gravity support exoskeletons [54] have shown significant improvements in clinical measures of stroke patients. Haptic guidance using vibration motors on the arms have recently been shown to be as effective as visual feedback for correcting motions [55]. In another study looking at targeted force-based movement, haptic feedback was shown to lead to improved ease of use and success in accurately completing the virtual finger-pointing task, but the task with haptic feedback was slower [56].
Methods based on simple haptic and visual methods are ideal for home use since they are cheap and easy to use. The downside of them is that they are unable to provide any significant force assistance, so they are not going to be effective for moderate to severely impaired individuals. However, it is unlikely that home-based rehabilitation would ever be appropriate for individuals with severe impairment as they will likely need additional supervision and care.
14.5 Upper Limb Rehabilitation
This section will look specifically at methods for upper-limb rehabilitation and discuss prospects for expanding the effectiveness of home-based methods.
14.5.1 Home Based Rehabilitation Methods
As discussed earlier, the ability to train more often leads to better results in motor relearning [4]. Home-based methods have been shown to help maintain individuals’ ability to perform activities of daily living [5, 6]. There are several methods that have been adapted for home use, but they are limited to individuals with mild impairments. Ideally, moderately impaired individuals would also be able to benefit from home-based rehabilitation.
The SMART system [57] incorporates a motion tracking system to monitor performance of daily tasks and rehabilitation exercises, an online database that allows therapists to monitor patient performance, and a visual feedback system that therapists may use to provide instruction. Java Therapy [58] uses a commercially available force feedback joystick and a suite of online games to provide therapy and evaluation. Another home computer based method is UniTherapy, which uses a force feedback joystick and steering wheel [59, 60] and has been validated in clinical trials [61, 62]. These home-based methods, however, use a home computer with limited accessories that can only provide limited assistance forces and have a limited workspace. These methods are able to provide some benefit, but the rehabilitation effect is limited to people who have relatively high motor function. The challenge is to develop safe and affordable rehabilitation for individuals with moderate impairment.
The commercially available MOTOmed arm cycling training device (Fig. 14.3) targeted towards stroke, multiple sclerosis, hypertension, cerebral paresis and Parkinson’s disease, offers a home-based rehabilitation option. For a relatively low price, patients can perform daily rehabilitative cycling exercises within the comfort of their home. MOTOmed can be customized to be used passively, motor-assisted, or active resistively. Rhythmic Arm cycling has shown to increase upper limb performance [63] and reduces arm spasticity [64, 65].
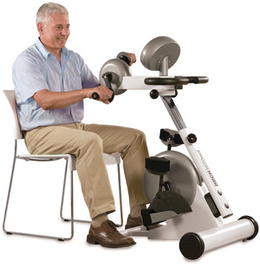
Fig. 14.3
The MOTOmed® is a arm cycling device with passive, motor-assisted, or active resistive options (Figure reproduced with permission)
14.5.2 Bimanual Rehabilitation
Self-rehabilitation using bimanual rehabilitation is ideal for home-based stroke therapy since much of the required force could be provided by the person’s sound limb and minimal, or no, external assistance would be required from a caregiver or a motor. The idea behind bimanual rehabilitation is that an individual assists his own paretic arm with his sound arm through an external physical coupling. Neither a physical therapist nor a robot can determine the exact path a person wants his arm to move as well as the person can. When an individual moves both the paretic and sound arms at the same time, the same signal is sent from the brain to the arms and, since the arms are constrained to move together, the proprioceptive feedback will be similar between the two sides of the brain. Burgar et al. hypothesize that bimanual symmetric exercise will enhance recovery by stimulating the ipsilateralcorticospinal pathways [66], which is similar to the hypothesis by Wolf et al. [67] that bimanual therapies could target the ventromedial brain stem pathways. The fundamental idea is that duplicating the efferent and afferent signals will retrain the motor pathways on the paretic side.
Many devices have implemented bimanual motions in a subset of the coupling modes shown in Fig. 14.4. In Joint Space Symmetry, the joint angles for the left and right arms are identical, resulting in hand motions that are mirrored about the sagital plane. In Visual Symmetry, both hands move in the same absolute direction in the visual reference frame. VS occurs in many daily activities, such as moving a large object with both hands. In Point Mirror Symmetry, the hand motions are mirrored about a point in space, much like turning a steering wheel.

Fig. 14.4
There are three common symmetric motions in which bimanual rehabilitation is typically performed: joint-space symmetry (JSS) where the joint angles are mirrored; visual symmetry (VS) where the hands move through the same visual path; and point mirror symmetry (PMS) where the hand motions are mirrored about a point in space
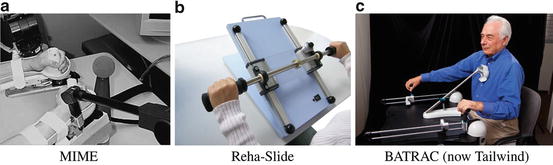
Fig. 14.5
Several of the devices that have been used for bimanual rehabilitation. The general idea of bimanual rehabilitation is that an individual assists his own paretic arm with his sound arm through an external physical coupling (Subfigure (a) reproduced from [48] under the Creative Commons Attribution License. Subfigures (b) and (c) reproduced with permission)
14.5.2.1 Robotic Bimanual Rehabilitation
Robotic bimanual rehabilitation uses a robotic device to assist an individual in making bimanual motions, some of which are shown in Fig. 14.5. The Mirror Image Movement Enabler (MIME) mirrors the position of the sound arm to the paretic arm using a large industrial robot, the PUMA 500 [66], but the study did not clearly identify the effectiveness of bimanual rehabilitation since subjects used a combination of unimanual and bimanual training [1]. Whereas the MIME focuses on mirroring the overall position of the hands, the BiManuTrack mirrors the flexion of the forearm and wrist and has shown positive results similar to the MIME [68] and to unimanual therapy [69].
Although these devices have been shown to be effective, they are limited to hospital and clinical settings. In recent years simpler passive devices have been developed that are well-suited for home-use rehabilitation.
14.5.2.2 Passive Bimanual Rehabilitation
Passive bimanual rehabilitation devices rely solely on the patient to generate motions, and do not provide assistive forces. Some of these devices have no physical coupling and rely entirely on the impaired arm to generate motions, while others physically link the hands, allowing one arm to assist the other.
BATRAC incorporates one such device where individuals independently move their hands along a linear track with in-phase and out-of-phase motions [70]. BATRAC has shown results similar to dose-matched therapeutic exercises [71]. A commercially available version of the BATRAC device, called Tailwind, is available and may be suited to home-use, although home use trials have not been conducted.
The Reha-Slide [72] implements bimanual motions only in the visual reference frame. The hands are linked by a rigid mechanism that allows an adjustable amount of friction. The entire effort to move the handles, including friction, is controlled by the sound side, The Reha-Slide is simple and demonstrated some improvement, but was not as effective as the BiManuTrack.
The ImAble system includes two devices that couple motions in a visually symmetric frame [73]. The Able-B supports the impaired arm against gravity while allowing the unimpaired arm to assist in making horizontal motions. The Able-X consists simply of a rigid handlebar with a motion sensitive game controller. The devices are used to interact with a suite of virtual reality computer games and have shown positive improvements in a pilot study [73]. The Able-X is commercially available for home use, however its home use efficacy has not been studied.
14.5.2.3 Compliant Bimanual Rehabilitation
The preceding devices have shown bimanual rehabilitation to be effective, but each only used one symmetry mode and either a rigid coupling or no coupling. However, for healthy individuals, certain motion types are easier to duplicate in one symmetry mode than another [74, 75]. These results indicate that the coupling symmetry mode and stiffness could affect the effectiveness of bimanual rehabilitation. Healthy individuals could recreate bimanual motions more easily in VS if the task was a rapid, unpredictable motion, but could recreate rhythmic motions more easily in JSS [76].
For bimanual rehabilitation, a completely rigid coupling may cause the individual to entirely rely upon their sound arm for all motions [13, 40] whereas a coupling that is too soft would completely eliminate the effect of the coupling, which would prevent individuals with low motor function from benefitting from this training method. With a flexible coupling, the paretic limb will have some assistance, but will apply at least a minimal amount of force, and the assistance force can gradually decrease throughout training. As discussed in Sect. 14.3, allowing the individual to gradually adapt will likely train the individual better than an all-or-nothing approach.
To directly compare the rehabilitation efficacy of different bimanual symmetry modes and coupling stiffnesses, a Compliant Bimanual Rehabilitation Device (CBRD) has been developed. This device allows the hands to be coupled in JSS, VS, or PMS, with a wide range of coupling stiffnesses, from 100 to 2,000 N/m. Preliminary results have shown that the CBRD can couple the motions of two healthy individuals in a task that simulates hemiparesis [77, 78]. This study also showed that the CBRD may improve bimanual task performance of healthy individuals.
14.6 Lower Limb Rehabilitation
Most survivors of stroke, persons suffering from traumatic brain injury, paraplegia, tetraplegia, multiple sclerosis, cerebral palsy, or hydrocephalus are known to suffer from motor deficits, including hemiparesis. Hemiparesis in the lower limb leads to hemiparetic gait, which is characterized by an asymmetric walking pattern [79, 80]. Hemiparetic gait typically includes asymmetries in walking coordination measures, such as step length and double support. In other words, the placement and timing of each foot are not equal on the two sides. The rehabilitation techniques used for this target population can be categorized as one of the following:
Classic gait Rehabilitation (Neurophysiological and Motor Learning)
Robotic Devices
Functional Electrical Stimulation
There are generally not as many methods available for home-based lower-limb rehabilitation as there are for upper-limb due to the added complications related to balance and stability when standing/walking. Below is a description of existing methods and how they could be adapted for the home.
14.6.1 Classic Gait Rehabilitation for Hemiparesis
Currently, the most common gait rehabilitation techniques are classic methods, but robotic devices are gaining acceptance [81]. Classic gait rehabilitation mainly includes preparatory exercises, such as calisthenics, mild stretching, range of motion exercises [82, 83], and guidance/assistance of the limb position while walking over even ground in conjunction with a physical therapist. Classic gait rehabilitation has two subcategories: neurophysiological techniques and motor learning techniques. In the neurophysiological rehabilitation approach, such as Bobath [22] and the Brunnstrom method [84], the patient is the passive recipient of the physiotherapist’s corrective and assistive movements [85]. Motor learning approaches, such as the Perfetti method [86], are quite the opposite in that they emphasize active patient participation [87]. Although these are two distinct approaches to hemiparetic gait rehabilitation, in practice each method is customized for each specific situation and patient. For these two categories, no method has been explicitly developed for gait rehabilitation [88]. While overground gait training still persists to be the most commonly used method for patients who are unable to walk independently [89], States et al. [90] indicate that there seems to be no distinct benefits caused by this method. It is suggested that a combination of methods, such as the use of body weight supported treadmill training [91], is a more effective approach [92]. An example of a body weight supported training in combination with guided therapy is shown in Fig. 14.6.
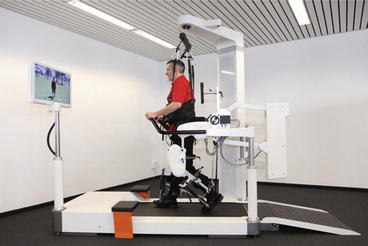
Fig. 14.6
The Lokomat® is a gait-assistive device with a built-in body weight support system (Picture: Hocoma, Switzerland)
Home-based motor imagery exercises for gait rehabilitation can also be an effective tool to improve walking performance in patients. Motor imagery is a cognitive operation that increases brain activity in neuronal cortical networks [93], or in other words, motor imagery is a meditation technique that focuses on the visualization of proper limb movement. Dunsky et al. [94] studied the effects of motor imagery exercises on 17 hemiparesis patients over a period of 3 months. As a result, walking speed increased by 40 % with gains retained at the 3-week follow up. Also, a significant increase in stride length, cadence, and single-support time of the affected limb was reported along with a significant decrease in double-support time. A comprehensive review of imagery exercises for gait rehabilitation [95] reveals similar positive effects, however further clinical studies with strong designs and larger groups are needed for confirmation of these positive findings. If validated, motor imagery would be suitable for home-based therapy that could include a large range of individuals.
14.6.2 Robotic Devices for Hemiparesis Rehabilitation
Classic gait rehabilitation methods alone are unable to restore a normal walking pattern in many stroke patients [96] and are progressively used in conjunction with robotic devices. There are several advantages in the use of robotic devices for gait rehabilitation: reduction of physical assistance and therapy cost, data acquisition, measurement and assessment, and repeatability [3]. Studies indicate that introducing robotic devices into gait rehabilitation results in improved endurance, lower-limb balance, functional balance, gait symmetry, double stance support, and stride length [97–99].
Exoskeleton type robotic devices are commonly used with body weight support systems with an assist-as-needed control law. The Lokmat [100, 101], shown in Fig. 14.6, is an electromechanical exoskeleton that employs a zero-impedance control mode, or path control [102], which allows patients to freely move their limbs while walking. The concept of virtual tunneling, which guides the patient’s motion through a force field, is applied in the lower extremity exoskeleton ALEX [103]. The Lokomat and ALEX both are used with a treadmill, body weight support system, visual feedback, and goal oriented training. However, due to their large size, exoskeletons are not likely candidates for home use.
The robotic platform by Monaco et al. [104, 105] offers an automated lower limb rehabilitation device, named NEUROBike, to patients during the initial acute phase when individuals are not yet able to keep an upright walking posture. The NEUROBike system essentially guides the position and orientation of the individual’s feet in the sagittal plane to mimic normal gait. Although still in its developmental stage, this rehabilitation device can potentially offer a simple home-based system, but more study is necessary.
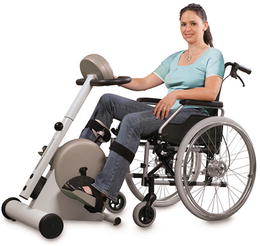
Fig. 14.7
The MOTOmed® is a passive, motor-assisted, or active resistive cycling training device (Figure reproduced with permission)
At a relatively lower cost, a simpler, yet effective approach is presented in the commercially available lower and upper limb rehabilitation device named MOTOmed by Reck, shown in Fig. 14.7. The MOTOmed is a lower (or upper) limb cycling device that can be used in a sitting position, in supine position, or with Functional Electrical Simulation (FES). It is designed to be used in a home environment on a daily basis. Passive, motor-assisted, or active resistive training make the MOTOmed customized to an individual’s rehabilitation needs. Lower limb rehabilitation studies which utilized the MOTOmed five times a week have shown improvement in patient balance, walking distance (or gait), step length, increased muscle tone, and reduction in spasticity [106–108]. Such leg cycling rehabilitation in conjunction with electrical stimulation has also been shown to reduce hypertonia in patients with stroke [108].
Balance control is a concern for individuals with stroke and, for severely impaired individuals, shifting weight so they can take a step is challenging. A recent study examined the effects of robot-assisted balance training in which an external perturbation force field was applied to individuals while leaning only and also when taking a step [109]. The results showed that the stepping group had a larger change in the asymmetry of their gait patterns, which indicates that training needs to occur in a dynamic environment.
Once a hemiparasis patient is able to stand up and walk with appropriate supports, similar corrective forces in the sagittal plane can be applied to the patient’s knee joint through Series Elastic Remote Knee Actuator (SERKA) [110] to achieve correct walking movements. SERKA compensates for the patient’s lack of strength and endurance during knee flexion by applying corrective torques at key instances during the gait cycle.
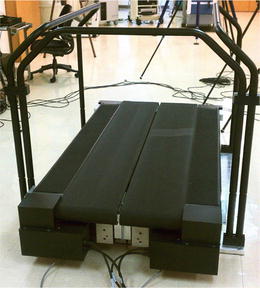
Fig. 14.8
A split-belt treadmill can be used to reduce an asymmetric gait. The downside is that the corrected gait does not efficiently transfer to walking over ground
While treadmills are not generally categorized as robots from an engineering standpoint, in gait rehabilitation they are classified as robotic devices. Lower-limb rehabilitation commonly includes split-belt treadmills [111, 112], as shown in Fig. 14.8. Such systems enable independent control of the two treads that each leg walks on, forcing one leg to move faster through stance than the other. Healthy participants with typical gait will walk symmetrically on the treadmill when the belts are running at the same speed (i.e., speed ratio is 1:1). When the speed ratio is changed to 2:1, these participants develop an asymmetric gait since their feet are moving at different speeds when in contact with the treadmill. After 10–15 min of walking at a 2:1 ratio, these participants adapt the spatial and temporal relationships between their legs to reestablish a symmetric walking pattern. When the belt speeds are returned to normal (1:1 ratio), the modifications made to gait during split-belt walking are temporarily remembered. The modifications result in an asymmetric gait that is opposite to the asymmetry induced initially by the split-belt treadmill, which is an after-effect similar to that discussed in Sect. 14.3. The same method can be used to correct individuals with asymmetric gaits.
Although the split-belt treadmill can change the interlimb coordination while walking on the treadmill, the effect does not completely transfer to over-ground walking. When after-effects are assessed over ground, the magnitude is diminished to 10 % of the magnitude of treadmill after-effects in control participants [113]. For individuals who have suffered a stroke, the transfer to over-ground walking is better at 30–70 %, but the effect is variable and diminishes rapidly following a single training session [114]. It has also been hypothesized that the limited transfer is due to conflicting sensory experiences between the treadmill environment and the over-ground environment [115]. On a treadmill, the scene is not moving, so there are no visual cues reinforcing the forward motion that would be present when walking over ground. Since walking is highly context dependent [113, 115, 116], these cues indicating a different context may prevent the learned patterns on the treadmill from being expressed during over-ground walking. In other words, if the participant is aware that the training conditions are different from the testing conditions, this may limit the transfer. Also, walking on a treadmill limits one’s ability to change velocity whereas, when walking over ground, an individual has complete control over velocity. There are also slight differences in the passive dynamics of walking over ground and walking on a treadmill [117].
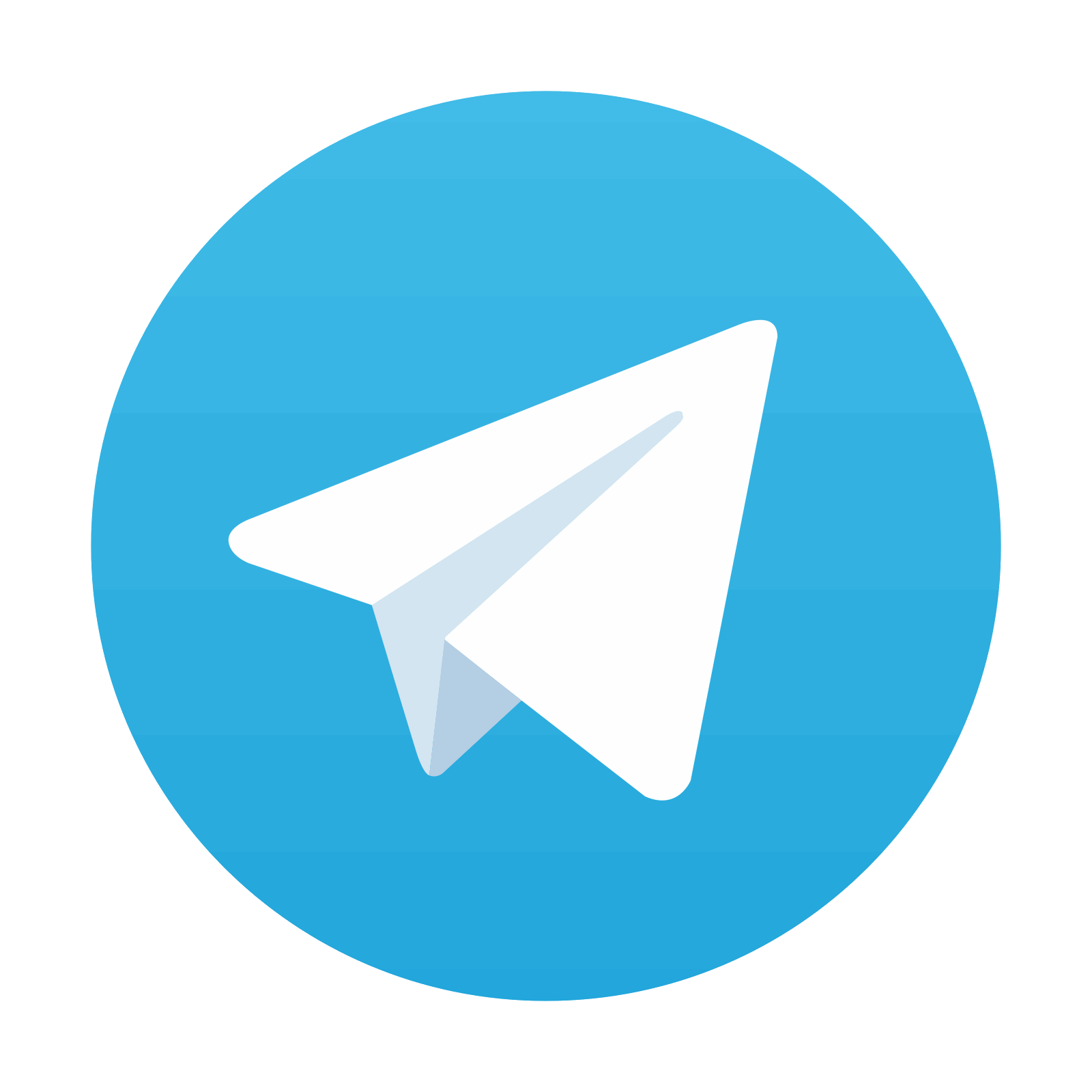
Stay updated, free articles. Join our Telegram channel

Full access? Get Clinical Tree
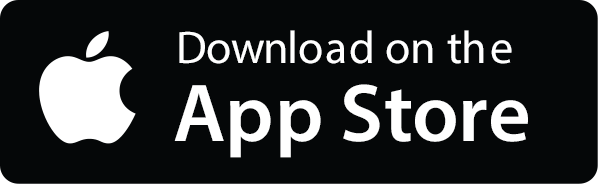
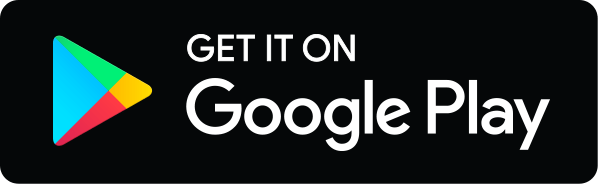