(1)
Department of Behavioral Neurobiology, Institute of Experimental Medicine, Budapest, Hungary
Abstract
The presentation of hormonal effects may be surprising in a work that focuses on the neural control of aggression. Hormones affect the organism in multiple ways, but none of these involves the direct activation of neurons or neuronal circuits. As such, they are not able to directly control the execution of behaviors. However, hormones have a large impact on how the neural system works. The neural mechanisms discussed in the next chapter are under hormonal control; moreover, dysfunctional aggression likely results from neural changes that are prompted by changes in hormone secretion patterns. As such hormone secretion patterns can be viewed as explanatory mechanisms for the neural control of aggression.
2.1 Hormones and Brain Function
The presentation of hormonal effects may be surprising in a work that focuses on the neural control of aggression. Hormones affect the organism in multiple ways, but none of these involves the direct activation of neurons or neuronal circuits. As such, they are not able to directly control the execution of behaviors. However, hormones have a large impact on how the neural system works. The neural mechanisms discussed in the next chapter are under hormonal control; moreover, dysfunctional aggression likely results from neural changes that are prompted by changes in hormone secretion patterns. As such hormone secretion patterns can be viewed as explanatory mechanisms for the neural control of aggression.
It is important to note that hormones affect brain function in an anatomically poorly specific manner: hormonal effects are exhibited wherever their receptors are expressed. Thus, hormonal effects are unspecific, which raises an important question: how do the nonspecific neural effects of hormones translate into specific behavioral roles? This question was frequently asked when I lectured on the relationship between glucocorticoids and aggression. The response to this question is that the specific role of hormones in behavioral control is determined not by the behavior specificity of hormonal effects but by the specific relationship between behaviors and brain activation patterns.
On a neural-mechanistic level, the previous statement can be explained in the following way. Any behavioral act is controlled by a specific set of neurons that form a network. If the properties of neurons are altered by hormonal influences, the functioning of the network is also altered, and consequently, the behavior controlled by the network will be changed. The effects of hormones are naturally not restricted to those neurons that control a particular behavior. However, effects on networks unrelated to the context (i.e., are not “working” in that particular moment) remain “silent” because they do not contribute to the execution of the behavioral act. According to this model, the specificity of hormonal effects derives from the context (Fig. 2.1). This mechanistic model can be complemented by situation-specific effects on emotional responses. In contrast to neural networks controlling movements (e.g., behavioral acts), emotions are controlled by extended brain regions commonly known as the limbic system, which is subject to hormonal effects as a whole. Emotions, however, do not involve the activation of the whole limbic system. On the contrary, particular emotions are associated with particular activation patterns as shown by brain imaging studies and amply detailed later. Consequently, hormones may indeed have overall effects on the limbic system, but only those effects become manifest under particular situations, which are related to the context.
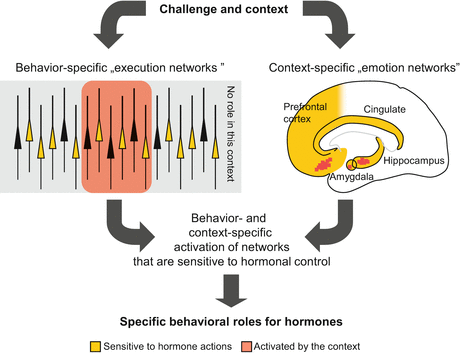
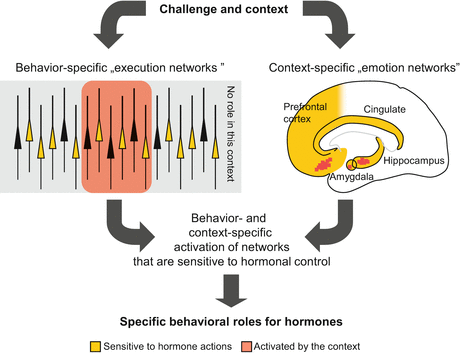
Fig. 2.1
A theoretical model of the behavioral specificity of neurally non-specific hormonal actions. The main point of this figure is that the behavioral specificity of hormonal actions is not defined by their neural specificity but by the behavior-specificity of brain activation patterns. Upper left schematic representation of a layer of cortical pyramidal neurons. While hormones affect the properties of many neurons, the consequences of this are manifested only when the neurons in question contribute to the elaboration of the actual behavioral response. Upper right schematic representation of the main limbic areas that control emotional responses. Emotions are associated with a specific pattern of limbic activation as shown by brain imaging studies. Again, hormones affect emotional responses specifically by virtue of the specificity of such activation patterns
We illustrate these by taking testosterone as an example. This hormone affects brain mechanisms involved in sexual behaviors but also those that are involved in aggression. While both mechanisms are promoted by testosterone, its effects on sexual behavior cannot be observed when the subject is challenged aggressively. The behavioral effects of testosterone are made specific by the context.
We discuss below the roles of two hormones: testosterone and glucocorticoids. These are not the only ones that affect aggression but seem more important than others for several reasons: (1) both hormones affect neural development by genomic actions. Aggression-related psychopathologies seem to be developmental disorders; therefore, studying mechanisms that influence neuronal development are revealing on how aggression-bound personalities arise; (2) both are highly sensitive to environmental influences and affect gene expression profiles throughout the lifespan. By these processes they continuously shape the function of the neural system and have an impact on aggression-related traits; (3) both have rapid, non-genomic effects, by which they are able to control ongoing behaviors.
In addition, they continue to be hot topics in aggression research, despite the fact that their roles were discovered rather early. While many studies attest their important role, contrasting findings are also frequent. We will argue below for the idea that contradictions are not due to experimental errors or misjudgment, but derive from the complexity of hormonal effects and the complexity of aggressive behavior. We will show that the role of hormones in aggression cannot be described in simple terms like “promote” or “inhibit.” Their effects depend on the constellation of several factors among which the context is likely the most important one. Both hormones are deeply involved in the control of aggression but not in all types of aggression, not in every context, and not linearly.
2.2 Testosterone
The dependence of animal aggression on testosterone secretion was scientifically recognized in the mid-nineteenth century (see below) and was amply confirmed by animal research ever since. The general idea is that the role of testosterone in aggression derives from its role in sexual behavior, as the ability to monopolize resources is a crucial factor of reproductive success, while access to resources depends to a large extent on aggressiveness. Testosterone is believed to be a common controlling factor of both. By contrast, the role of testosterone is considered uncertain in humans, where aggression has a minor role in sexual competition. Findings support neither assumption. Animals can be aggressive without testosterone, and evidence linking testosterone to human aggression is more robust than generally thought. The findings reviewed here, together with the theories concerning the role of testosterone, strongly support the notion that testosterone is an important mechanism of aggression control and significantly contributes to the emergence of abnormal forms of aggression. It will also be shown that aggression is not controlled by testosterone alone, and its role is embedded in a wider array of controlling mechanisms. We will argue that the role of testosterone cannot be understood without incorporating it into the context of other controlling mechanisms.
2.2.1 Mechanisms of Action
Similar to other steroid hormones, testosterone exerts its effects by modulating gene expression. Briefly, testosterone enters the cytoplasm where it remains intact or is metabolized to dihydrotestosterone or estradiol, which binds to androgen or estrogen receptors, respectively, and affects the transcription of genes that express androgen or estrogen response elements. Such hormone receptors and hormone response elements are nearly ubiquitously expressed, which explains the multitude of testosterone effects that range from the control of sperm production to aggression but at the same time makes it difficult to pin down the exact mechanism by which one particular phenomenon (e.g., aggression) is regulated by genomic effects.
In addition to the widely recognized role of testosterone in gene expression, recent findings suggest that this hormone may affect neuronal function and behavior by non-genomic mechanisms. In contrast to genomic effects, non-genomic ones are exerted rapidly and may affect ongoing behavior. Unfortunately, the non-genomic effects of testosterone are poorly known at present, and to our knowledge, there are no convincing reports on their implication in aggression control. By contrast, such non-genomic effects were implicated in the regulation of vascular and sexual functions, sexual behavior, social communication, and cognition (Balthazart et al. 2009; Cho et al. 2003; Cornil and Charlier 2010; Heinlein and Chang 2002; Walker 2003; Nyby 2008). Non-genomic behavioral effects are mediated by effects on membrane fluidity, interactions with intracellular calcium regulatory mechanisms, the activation of second messenger pathways (e.g., the mitogen-activated protein kinase), and modulatory actions on membrane receptors (GABAA, NMDA, glycine, nicotinic, and vasopressin V1a receptors) (Foradori et al. 2008; Gabor et al. 2012). In the absence of direct evidence on aggression, all effects will be discussed as being induced by genomic mechanisms, while the involvement of non-genomic ones seems quite likely.
It is important to note here that the activation of mechanisms influenced by testosterone may not entirely depend on its plasma levels. A large share of this hormone is sequestrated by sex hormone-binding globulin, and only free testosterone is ready to enter the cells. Binding and release from this globulin is a dynamic process influenced by many factors, not lastly by its amount. While the issue was not investigated systematically, a few studies indicate that the plasma levels of sex hormone-binding globulin free testosterone have a role in determining the degree to which testosterone can influence aggressive behavior in both animals and humans (Aluja and García 2007; Brambilla et al. 2001; Pajer et al. 2006; Witte et al. 2009). Such interactions may be especially relevant to short-term (possibly non-genomic) effects of testosterone, where the momentary availability of this hormone may have a large impact. Other modifiers of testosterone action are the enzymes that convert it into active metabolites, e.g., dihydrotestosterone and estrogen. The expression level and activity of 5α reductase and aromatase greatly influences the ability of testosterone to influence aggressiveness (Bethea et al. 2013; Compaan et al. 1994a, b; Schlinger and Callard 1990; Soma et al. 2003; Wu et al. 2009). Finally, the expression of receptors that mediate the effects of testosterone and its active metabolites also has an impact on testosterone effects (Bergeon Burns et al. 2013; Canoine et al. 2007; Scordalakes and Rissman 2004; Trainor et al. 2006). Noteworthy, testosterone effects are often influenced by these modifiers in a brain area-specific manner.
Taken together, these findings suggest that evaluating the effects of testosterone is not an easy task. For example, reduced plasma levels of the sex hormone-binding globulin, brain area-specific enhancement of aromatization levels, or receptor expressions may make testosterone efficient even if plasma levels are low. As such, negative findings may be misleading when only testosterone levels are measured.
2.2.2 Developmental Effects on Aggression in Animals and Humans
2.2.2.1 Normal Aggression
The first effects of testosterone on animal aggression are exerted in a critical perinatal period. At this early age, testosterone acts as an organizing principle that makes the brain sensitive to its own effects that are exerted much later, beginning with the pubertal period (Fig. 2.2). This “organizing” role of testosterone was first recognized in conjunction with sexual behavior. It was stated already in the 1960s that “Sex hormones of the embryo affect ultimate sexual behavior in two ways: indirectly by their control of morphogenesis of the genitalia and, in some species at least, directly by their neural-organizer effect” (Money 1965). It was soon discovered that testosterone has similar effects on aggressive behavior: neonatally administered testosterone increased, while neonatal castration decreased adult aggression in mice (Bronson and Desjardins 1968, 1969; Edwards 1969; Peters et al. 1972). This increase in aggression was found to be conditional; instead of leading to an overall increase in aggressiveness, neonatal testosterone in fact sensitizes the neural system to its later effects: the aggression-heightening effects of testosterone in adulthood are facilitated by perinatal exposures to testosterone (Hutchison 1974; Klein and Simon 1991). The early effects of testosterone are so strong that it can induce male-like aggressive behavior in female rodents, provided that these are treated with testosterone in adulthood (Gandelman et al. 1979). Moreover, intrauterine position, e.g., the sex of neighboring embryos, also has a role in establishing adult aggressiveness by virtue of the diffusion of testosterone through the amniotic fluid (Quadagno et al. 1987; Ryan and Vandenbergh 2002). The early organizing effects of testosterone with regard to its later behavioral effects are bound to a specific time window of animal development; this time window is species dependent and short; it encompasses a few hours or days shortly before, during, or after birth (Dixson 1993; Klein and Simon 1991; Motelica-Heino et al. 1993).
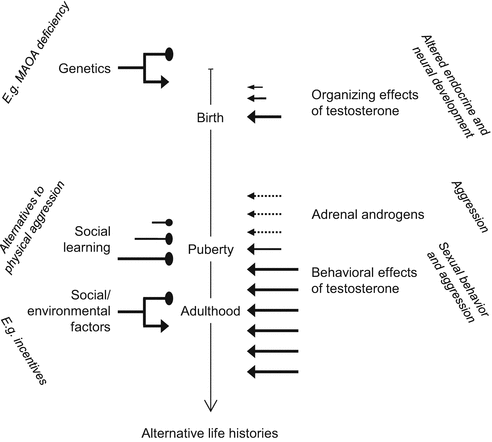
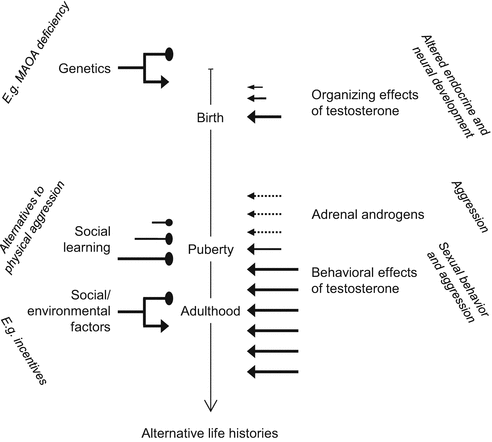
Fig. 2.2
The two waves of testosterone actions. The organizing effects of testosterone around birth includes changes in the development of the endocrine system (testosterone production, aromatization, androgen/estrogen receptor expression) of brain structure (size of various brain areas) and brain function (altered neurotransmission). These determine the way in which testosterone affects behavior in adulthood (including effects on endocrine variables). Around puberty, adrenal androgens (converted into testosterone and estrogens in the brain) may also mediate the effects of early testosterone exposure. The left-hand side of the graph illustrates the non-deterministic nature of testosterone effects, that may be augmented (arrows) or inhibited (oval pointed heads) by other factors. Such factors are only exemplified
These findings demonstrate that there is critical time period in the early development of animals, when testosterone “transforms” the brain such that it becomes sensitive to its own aggression-promoting effects in adulthood. Similar changes were seen in humans, where the prenatal period is also considered crucial for the sexual differentiation of brain and behavior (Warne et al. 1977; Rubin et al. 1981). This assumption is supported by three lines of evidence: (1) congenital adrenal hyperplasia, a condition that is associated with high testosterone levels in early periods of life, results in male-like aggressive behavior in females (Berenbaum et al. 2000; Hines et al. 2002); (2) prenatal exposure to androgens via treatments received by mothers increased aggressiveness in both male and female offsprings (Reinisch 1981); and (3) the length ratio of the second and fourth fingers (a somatic marker of prenatal testosterone exposure called 2D:4D digit ratio) associates negatively with aggressiveness, suggesting that larger testosterone exposure during embryonic life (when digit length is determined) is associated with higher aggression during adulthood (Butovskaya et al. 2013; Hampson et al. 2008; van der Meij et al. 2012). Moreover, girls of opposite-sex twins show a more masculine pattern of aggression than girls of same-sex twins, which may be considered a human analog of the intrauterine position effect seen in animals (Cohen-Bendahan et al. 2005).
Taken together, the findings reviewed above suggest that the developmental effects of testosterone are rather similar in animals and humans. Contradictory findings also exist. Voracek and Stieger (2009), for instance, found no association between 2D:4D digit ratio and aggression, and the first author of the above paper questioned the validity of the findings of van der Meij et al. (2012) based on data-analytic and statistical power issues (Voracek 2013). Such contradictions may be solved by taking aggression types and genetic constitution into consideration. Millet and Dewitte (2009) for instance found that 2D:4D digit ratio predicted aggression in the dictator game (a variant of the ultimatum game presented in Chap. 1) only when an aggression cue was present; in neutral situations, the low (more masculine-like) digit ratio predicted prosocial behavior. In line with this, Benderlioglu and Nelson (2004) found that a low 2D:4D digit ratio predicted aggressiveness in females under high provocation only. These authors deduced aggressiveness from the follow-up letters of subjects solicited to give donations for a fictitious charity organization. Under these conditions, digit ratios predict aggressiveness in women but not in men. In a study investigating trait aggression, the expected correlation with digit ratios was found in males but not in females (Bailey and Hurd 2005). Congenital adrenal hyperplasia by contrast increases aggression in females but not males (Pasterski et al. 2007). In another study, trait aggressiveness was predicted by digit ratios in conjunction with dopamine D4 receptor polymorphism (Butovskaya et al. 2012). Such findings may be considered contradictory, but in our view the differences reflect genetic background-, gender-, and aggression type-related differences in the involvement of testosterone.
2.2.2.2 Abnormal Aggression
Early treatment with testosterone induces male-like aggression patterns in female rodents, which may be considered abnormal by virtue of the second criterion of abnormal aggression (see Chap. 1, Sect. 1.2.2). Particularly, the criterion “disregard of species-specific rules” applies here and renders male-like behavior abnormal in females. As a similar phenomenon is noticed in congenital adrenal hyperplasia, the first criterion of abnormal aggression may also be met (mimicking an etiological factor of human abnormal aggression). Nevertheless, aggression is not a major symptom of this condition, which cannot be considered an aggression-related disorder. Moreover, aggressiveness is increased by this condition in females but not in males, a difference likely related to the reversal of sex roles. In contrast to the human case, early testosterone administration increases adult aggressiveness in rodents belonging to both sexes. Therefore, the resemblance between the human condition and the model is only vague. Beyond this equivocal similarity, there is no information on the nature of aggression elicited by early testosterone manipulations. This does not mean that such treatments result in normal forms of aggression in rodents; it only means that the issue was not investigated so far.
The relationship between early androgen hyperfunction and abnormal aggression was almost as poorly studied in humans as in rodents. There are only two studies suggesting a link. Liu et al. (2012) showed that early androgen exposure (as assessed by digit ratios) increased externalizing behavior in boys but not in girls. A similar association was recently found between digit ratios and intimate partner violence (Romero-Martínez et al. 2013). The only “flaw in the scheme” is that neither externalizing behavior nor intimate partner violence is an aggression-related psychopathology. Yet, a 24-year-long study of externalizing symptoms demonstrated that these often develop into, while intimate partner violence is frequently associated with antisocial behaviors; moreover, psychopathy (Fowler and Westen 2011; Reef et al. 2011). This suggests that early androgen hyperfunction may lead to abnormal human aggression, but the issue remains poorly studied.
2.2.2.3 Mechanisms of Developmental Effects
Perinatal testosterone hyperfunction (1) increases energy metabolism (Quadagno et al. 1977; Hill et al. 2012); (2) increases adult testosterone levels, the aromatization of testosterone to estrogens, and estrogen receptor expression (Ryan and Vandenbergh 2002; Schlinger and Callard 1989; Vaillancourt et al. 2012); (3) stimulates cell growth and differentiation in brain areas related to male-typical behaviors and aggression, e.g., the hypothalamic sexually dimorphic nucleus and its human equivalent, the 3rd interstitial nucleus of the anterior hypothalamus, the ventromedial hypothalamus, and amygdala (Hines 2010; Ryan and Vandenbergh 2002); and (4) upregulates the noradrenergic and dopaminergic innervation of the prefrontal cortex and downregulates the serotonergic innervation of the same area (Butovskaya et al. 2012; Dominguez et al. 2003; Stewart and Rajabi 1994). In addition it masculinizes the serotonergic innervation of the medial preoptic area in female rats (Simerly et al. 1985). Early testosterone treatments also affect the neuronal connectivity (Hines 2010). Importantly, findings in rodents and humans were highly compatible. Some of these testosterone-associated changes may be epiphenomena, but others may be causally linked to changes in aggressiveness.
2.2.2.4 Overall Evaluation of Developmental Effects
Summary of Findings
Findings in rodents clearly show that testosterone triggers the development of a testosterone-responsive regulatory system for aggressive behavior within a critical period of early life. The delayed behavioral effects of testosterone are likely mediated by its long-term effects on testosterone production, aromatization, and receptor expression; on the development of aggression-related brain areas; and on the development of noradrenergic, dopaminergic, and serotonergic neurotransmission. Findings in humans are highly compatible with those obtained in animals. The impact of early testosterone on abnormal aggression was not studied in animal models, but findings obtained in humans suggest its involvement in aggression-related psychopathologies.
Relevance
Early development is crucial for adult functioning; in the same vein, the early effects of testosterone on the development of aggression-related brain mechanisms may be crucial for aggressiveness expressed in adulthood. These functional and anatomical consequences of early testosterone exposure suggest that even normal adult levels of testosterone confer the individual a high predilection towards aggressiveness; moreover, early development may predispose individuals to abnormal forms of aggression if plasma testosterone levels increase. We note that while the available information can be ordered in a meaningful way, developmental studies are scarce, and many issues await clarification.
2.2.3 Postpubertal Effects in Animals: Normal and Abnormal Aggressions
2.2.3.1 Testosterone in Animals: Normal Aggression
Albeit castration was used for millennia to pacify domesticated male animals that are otherwise difficult to handle, the first scientific study attesting the role of the testes in aggression was performed by Arnold Berthold in 1847: he showed that castration decreased, while testis transplantation restored aggressiveness in domesticated roosters (cited based on Soma 2006). The particular role of testosterone was demonstrated considerably later by Seward (1945) and Beeman (1947) who showed that puberty-associated increases in testosterone are associated with the emergence of aggressiveness, while aggression suppressed by castration is restored by testosterone, respectively. These basic findings were amply replicated by subsequent research, which revealed further aspects of testosterone action. Seasonal variations in testosterone production covariate with aggressiveness (Butterfield and Crook 1968); current levels of circulating testosterone are in tight correlation with the propensity to behave aggressively (Rose et al. 1971); testosterone injected after the winning or the losing of aggressive encounters increases aggressiveness and decreases submissiveness, respectively, in subsequent encounters; and finally, social rank correlates positively with plasma testosterone in social groups (Lucion et al. 1996; Solomon et al. 2009; Trainor et al. 2004). This set of congruent findings resulted to a strengthened belief that there is a clear-cut causal relationship between testosterone production and aggression in animals—as opposed to humans, where this interaction is much less certain.
In fact, however, the testosterone-aggression link may be perceived as being just as volatile in animals as in humans. Albeit castration reduced aggressiveness in most studies published so far, there still exist carefully performed studies where this effect was not seen (California mice: Trainor and Marler 2001; lizards: Moore and Marler 1987; marmosets: Dixson 1993; prairie voles: Demas et al. 1999; rock hyrax: Manharth and Harris-Gerber 2002; Siamese fighting fish: Weiss and Coughlin 1979; song sparrows: Soma et al. 2000; Siberian hamster: Jasnow et al. 2000; wood rats: Caldwell et al. 1984); moreover, castration increased aggression in some species under certain circumstances (in laboratory mice, castration increases aggression towards lactating females: Haug et al. 1984; in mandarin voles, castration leads to an overall increase in aggressiveness: He et al. 2012). Thus, surprising effects of castration were seen in all major vertebrate classes and in a variety of taxa within mammals, including rodents. Interestingly, testosterone production was not totally disrupted from aggression control in these species; e.g., winning-related increases in testosterone facilitated aggression in subsequent encounters in California mice, despite the fact that castration did not reduce aggression in this species (Fuxjager et al. 2011).
Similarly, strange effects were noticed in studies investigating the correlation of aggressiveness with plasma testosterone levels, or the effects of testosterone administration. For example, basal plasma levels of testosterone did not show correlation with aggressiveness in Siberian dwarf hamsters (Castro and Matt 1997), wild-type rats maintained in the laboratory (Everts et al. 1997), the red jungle fowl studied in the laboratory (Johnsen and Zuk 1995), and in laboratory rats in the visible burrow system (Blanchard et al. 1995). Again, the relationship between testosterone and aggression was not totally disrupted in these models. In the study by Johnsen and Zuk (1995), basal levels did not, while acute increases in testosterone did correlate with aggressiveness; in the study by Blanchard et al. (1995), submission was not correlated with low testosterone when group members living in the visible burrow system were preselected for high aggressiveness, but the expected relationship was found when group members were not preselected for aggressiveness. As it regards testosterone administrations, these did not affect aggression in some studies (ground squirrels, postmating period: Millesi et al. 2002; male lambs, familiar environment: Ruiz-de-la-torre and Manteca 1999; European stonechats, nonbreeding season: Canoine and Gwinner 2002); moreover, testosterone decreased aggression in Siberian hamsters (Jasnow et al. 2000). As shown above for other models, aggression was not totally disrupted from regulatory control by testosterone, as treatments ineffective in the nonbreeding season or in familiar social environments became effective in the breeding season and in unfamiliar social environments (Canoine and Gwinner 2002; Millesi et al. 2002; Ruiz-de-la-torre and Manteca 1999).
The studies briefly reviewed above (and a series of others not cited here) show that there is no clear cause–effect relationship between testosterone production and aggressiveness. Even more strikingly, strange effects were noted in species where testosterone clearly controls aggression. For example, castration dramatically decreased, but did not abolish biting attacks in castrated mice; in addition, the surgery did not affect the frequency and duration of offensive threats (Clipperton-Allen et al. 2011). In male laboratory rats, Albert et al. (1986) reported that the presence of females increased aggression in both intact and castrated males. Thus, the absence of testosterone did not prevent males from being aggressive, not even in species where the relationship between aggressiveness and testosterone was believed to be clear-cut.
2.2.3.2 Is Testosterone “Responsible” for Aggression in Animals?
Asking the same question in a more general way: does a considerable body of contradictory findings question the role of testosterone in aggression? From a simplistic view it certainly does: the assumption that testosterone controls aggression is negated by these findings. However, testosterone does not control aggression: it affects the properties of neuronal circuits, which are under multiple influences. In other words, the neural and behavioral responses to social challenges are not defined by testosterone, but by the overall outcome of the multitude of concurrent influences. Interactions of this kind were systematically mapped in a few species, and several theories were advanced to explain the discrepancies reviewed above (Fig. 2.3).
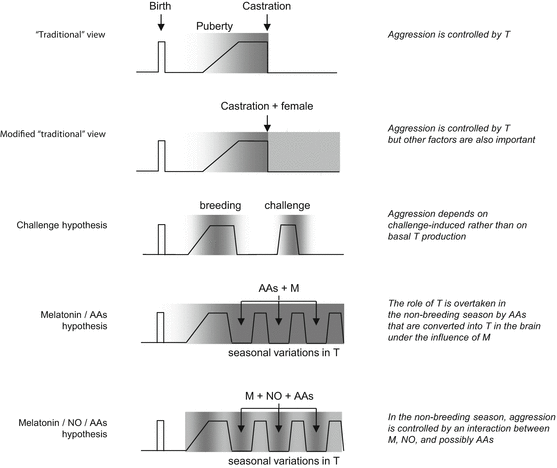
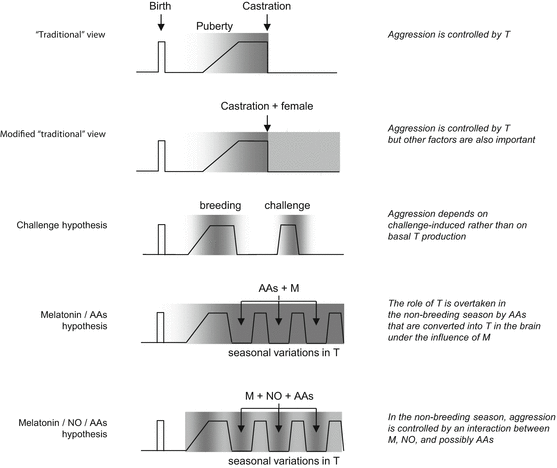
Fig. 2.3
Hypotheses on the role of testosterone and alternative mechanisms in animal aggression. zigzag lines, testosterone levels; gray colour and its intensity, presence of aggression and its itensity; Letters indicate alternative mechanisms. AA, adrenal androgens; M, melatonin; NO, nitric oxyde. The main point of this figure is that the testosterone/aggression link is not absolute in animals; powerful alternative mechanisms do exist
The first theory of this kind, the “challenge hypothesis” put forward by Wingfield et al. (1990), made a distinction between breeding season-related changes in testosterone production and those elicited by male–male interactions. The theory was based on data obtained in monogamous birds. It was hypothesized that maintaining high testosterone levels for the whole breeding season is detrimental in terms of both increased metabolic costs resulting from enhanced behavioral activity and reduced parental care, a “by-product” of enhanced testosterone production. Metabolic and parental trade-offs are solved by limiting testosterone production to the early phases of sexual competition and periods of social challenge in the parental period. Thus, the disruption of the testosterone-aggression link is ostensible; in fact, testosterone does control aggression, but its production is controlled by “needs.” This influential hypothesis was supported by a number of field and laboratory observations in birds and mammals (Cavigelli and Pereira 2000; Ferree et al. 2004; Muller and Wrangham 2004; Ros et al. 2002) and was exploited to explain the testosterone-aggression link in humans (Archer 2006).
The second theory addressing the disruption of the testosterone-aggression link was also based on data obtained in birds. This theory stipulates that nonbreeding season-related aggression (which is rather high in many species) is not controlled by testosterone but by adrenal steroids, e.g., dehydroepiandrosterone (Soma 2006; Soma et al. 2008). The mediator of this mechanism is melatonin, which is secreted in high amounts during the winter. Under the influence of this hormone, dehydroepiandrosterone is converted to estrogen (one of the active metabolites of testosterone) in the brain. Albeit based on bird data in the first place, this theory also assumes validity for other vertebrates (Soma et al. 2008). Albeit humans are no seasonal breeders, dehydroepiandrosterone blood levels correlate with certain forms of aggressiveness, suggesting that the mechanisms operating in animals are present in humans (Buydens-Branchey and Branchey 2004; Dmitrieva et al. 2001; van Goozen et al. 1998a, b, 2000a, b). Noteworthy, however, dehydroepiandrosterone does not affect aggression in a species that is critical for this hypothesis—the Siberian hamster, a seasonal breeder which shows high levels of aggression in the nonbreeding season (Jasnow et al. 2000; Scotti et al. 2008).
Studies in Syrian and Siberian hamsters gave rise to the third hypothesis. It was suggested that in these species, aggression is controlled by melatonin per se (Demas et al. 2004; Jasnow et al. 2002; Wang et al. 2012a, b). It remains to be elucidated how melatonin affects aggression. One series of evidence suggested that melatonin downregulates neuronal nitric oxide synthase activity independent of gonadal steroid hormones; according to this hypothesis, melatonin increases aggression in the nonbreeding season by reducing brain nitric oxide levels (Bedrosian et al. 2012; Wen et al. 2004). It is possible that the effects of melatonin are supported by testosterone synthesized from dehydroepiandrosterone under the effects of melatonin (Demas et al. 2004; Scotti et al. 2008, 2009). Taken together, aggressive behavior is independent of testosterone in certain species of hamsters, where aggression seems to be controlled by melatonin, likely by its effects on both nitric oxide synthesis and the conversion of adrenal steroids into testosterone. The consequences of this line of research for understanding aggression—beyond certain hamster species—are dual. Firstly, it directed attention towards the role of nitric oxide in aggression (Nelson et al. 2006); secondly, and more importantly for the present work, it revealed that the control of aggression may be overtaken by melatonin under certain conditions. Although this phenomenon received little attention in human research, some reports suggest that the aggression-promoting effects of melatonin are present in humans. For example, demented patients treated with melatonin showed increased aggression (Haffmans et al. 2001). Melatonin secretion may also be involved in self-injurious behavior (Herman 1990).
Taking into account the above, the response to the question asked in the title of this section is dual. Yes, testosterone affects aggression by interacting with brain mechanisms that control aggression. No, testosterone is not the only mechanism that controls aggression; moreover, there are contexts that render aggression independent of testosterone production. Under the influence of these contexts, alternative mechanisms are activated that enable aggressiveness to be expressed at high levels despite low levels of plasma testosterone.
2.2.3.3 Effects in Models of Abnormal Aggression
Testosterone seems to play a role in mice selected for aggressiveness. Short attack latency mice show higher levels of testosterone than long attack latency mice (the timid selection line) (van Oortmerssen et al. 1992; Compaan et al. 1993a, b, 1994a, b). Similar findings were obtained in Turku aggressive mice and cows selected for fighting ability (Plusquellec and Bouissou 2001; Sandnabba et al. 1994). The impact of testosterone seems weaker than that of genetic selection, because castration did not cancel genetically determined behavioral differences; however, aggression was decreased more strongly in short attack latency mice, suggesting that testosterone has a role in their abnormal aggression (van Oortmerssen et al. 1987). Subsequent research showed that the perinatal organizational effects of testosterone and adult brain aromatase activity are also altered in selection lines, further supporting the notion that abnormal aggression in these lines has a testosterone component (Compaan et al. 1992, 1993a, b, 1994a, b; Sandnabba et al. 1994; Sluyter et al. 1996).
The interaction between testosterone and abnormal aggression was also studied in the alcohol model of abnormal aggression. Two findings deserve attention: (1) the early organizational effects of testosterone and the effects of alcohol on aggression seem to interact because neonatal testosterone administration increased, while neonatal castration decreased the effects of alcohol on aggression in adulthood (Lisciotto et al. 1990; Winslow et al. 1988); (2) in adult animals (not treated in the neonatal period), there was a significant positive interaction between plasma testosterone and the effects of alcohol on aggression albeit this interaction was somewhat less robust (DeBold and Miczek 1985; Winslow and Miczek 1988).
In the case of other models, the role of testosterone in abnormal manifestations of aggression was not directly investigated (e.g., by castration or testosterone administration). Noteworthy, however, testosterone levels were measured in some of the models. Testosterone production was not altered in the maternal separation, postweaning social isolation, rat and hamster early subjugation, low-anxiety, and anabolic-androgenic models (Akbari et al. 2008; Amstislavskaya et al. 2013; Cunningham and McGinnis 2008; Ferris 2003; Ferris et al. 2005; Ortiz et al. 1984; Salas-Ramirez et al. 2008, 2010; Shimozuru et al. 2008; Veenema et al. 2006, 2007a, b; Veenema and Neumann 2009). In the high-anxiety model, basal levels remained unchanged, but the testosterone response to aggression was abolished (Veenema et al. 2007b). In the pubertal stress model, changes were seen not in testosterone levels per se but in the testosterone/corticosterone ratio increases (Márquez et al. 2013). In other models, e.g., the glucocorticoid dysfunction and repeated victory models, plasma testosterone levels likely increase but contradictory findings also exist (Balasubramanian et al. 1983; de Boer et al. 2003; Feek et al. 1989; Gao et al. 1996; Kalra and Kalra 1977; Poggioli et al. 1984).
In summary, the roles of testosterone were adequately studied in two models of abnormal aggression: in mice selected for aggressiveness and in the alcohol model. Testosterone appears to promote abnormal forms of aggression in both models. Indirect evidence suggests that the same is for the high-anxiety selection line, pubertal stress, glucocorticoid dysfunction, and repeated victory models, while a role for testosterone appears unlikely in other models. We mention, however, that this evidence is indirect.
2.2.4 Does Testosterone Affect Aggression in Humans?
2.2.4.1 Cons
The role of testosterone in human aggression is questioned and contested based on a series of arguments as follows: (1): females readily show aggressiveness despite their low testosterone levels; (2) aggression is not increased at puberty when testosterone levels increase; (3) high- and low-aggression individuals do not consistently differ in serum testosterone; (4) aggression does not increase in hypogonadal males when exogenous testosterone is administered to support sexual activity; (5) castration or antiandrogen administration to males is not associated with a consistent decrease in aggression; and (6) the replication of positive findings is often difficult. This set of contra arguments was formulated based on Albert et al. (1993); the reviews by Archer (1991) and Book et al. (2001) were also used. While studies that attest the role of testosterone in human aggression cannot be ignored, and these show that testosterone does control aggression, all the contra arguments listed above are valid.
Although less aggressive than males overall, females readily engage in aggressive conflicts in a variety of situations (aggressive response to hypothetical conflict situations: Reinisch and Sanders 1986; aggressive responses in the Taylor Aggression Paradigm: Böhnke et al. 2010a, b; bullying in prisons: Archer et al. 2007; criminal violence and homicide: Heide and Solomon 2009; dating violence: Cercone et al. 2005; intimate partner violence: Williams et al. 2008; substance dependence-related aggression: Bacskai et al. 2011). Gender differences were significant in most studies, but were not large. For example, the physical aggression score on the Buss-Perry Aggression Scale was 16.92 ± 0.69 in males and 14.63 ± 0.63 in females; gender differences were even smaller in substance-dependent subjects (males, 23.43 ± 0.49; females, 22.77 ± 0.89). In the study by Archer et al. (2007), males scored significantly higher on the physical aggression score of the Response to Victimization Scale (10.51), but the score of females was also considerable (7.58). Moreover, the score for displaced physical aggression was somewhat (nonsignificantly) higher in females (7.53) than in males (7.09). In the study by Böhnke et al. (2010a, b), unprovoked males and females showed comparable levels of aggression in the Taylor Aggression Paradigm; when provoked, males responded more aggressively, but females also increased their aggressive responses. Moreover, dating-related physical assault was significantly more frequent in female than in male undergraduate students (Cercone et al. 2005). Thus, females are almost as aggressive as males; this assumption is supported by meta-analyses showing that gender differences in aggression are consistent but are moderate in magnitude; the small effect size holds true for both verbal and physical aggressions (Hyde 2014).
The pubertal raise in testosterone production is accompanied by a rise in aggression in animals but not in humans. Moreover, physical aggression decreases in puberty as compared to childhood. While children primarily use physical aggression to resolve disputes, they gradually shift their behavior towards verbal (intimidation) and relational aggression to avoid the risk of retribution (Bjoerkqvist et al. 1992; Tremblay 1999). As a consequence, the positive correlation between testosterone and aggression seen in 12–13-year-old boys is lost by the age of 15–16 (Turner 1994), and plasma testosterone is linked to social dominance but not to physical aggression in early adolescence (Schaal et al. 1996).
Regarding the correlation between testosterone levels and aggression in males, we will avoid a “battle of references” (the counting of positive and negative findings) by taking examples from studies where the correlation was found significant. A common characteristic of such studies is that while the correlation is significant overall, individual correlation points are rather dispersed; consequently, some subjects are aggressive on the background of low testosterone, while other subjects show low aggression scores despite elevated plasma levels of testosterone. Such dispersed correlations or highly overlapping plasma testosterone ranges were found in a number of paradigms (aggressive delinquency: Banks and Dabbs 1996; Buss-Durkee Hostility Inventory: Ehrenkranz et al. 1974; Point Subtraction Aggression Paradigm: Carré et al. 2010; reactive aggression: Benderlioglu et al. 2004; response to anger faces: Wirth and Schultheiss 2007; venturesomeness in personality disorders: Coccaro et al. 2007a, b).1 The conclusion deriving from this analysis is supported by the meta-analysis of Book et al. (2001): testosterone is a significant but weak contributor of aggressive behavior. Finally, a number of studies attest that increased testosterone production in women (due, e.g., to polycystic ovarian syndrome), and hypogonadism in males, and testosterone-based corrective treatments have no significant effects on aggressiveness (Finkelstein et al. 1997; O’Connor et al. 2002; Shufelt and Braunstein 2009; Stanworth and Jones 2008; Weiner et al. 2004).
These are serious arguments in favor of the idea that the role of testosterone in human aggression is minimal. Nevertheless, a substantial body of evidence demonstrates that under particular conditions, the effects of testosterone are rather strong (see Sect. 2.2.5). This conflict prompted a series of speculations on how this endocrine factor may affect aggression strongly such that its overall effects remain small.
2.2.4.2 Theories
A simplistic approach would suggest that the conflict is explained by errors in investigation. However, the large number of studies and the high scientific level at which many of these were performed excludes this possibility. The volatility of findings is not due to the volatility of methodologies, even if there was a permanent need to improve these. It occurs that the reason of contradictions lies in the complexity of the phenomenon. In fact, the question is not whether testosterone controls aggression or not; the real question asks about the conditions and circumstances that make testosterone operative or ineffective.
The Defensive Aggression Hypothesis
The first animal studies substantiating the hypothesis were performed by Albert et al. (1986), while the theory was formulated by Albert et al. (1993). Animal observations suggested that in contrast to rivalry aggression which is typical to males, defense against predators is shown by both genders and does not depend on testosterone. In its final form, the theory postulated that human aggression is not influenced by testosterone because it resembles defensive aggression in animals (Albert et al. 1993). This resemblance is not very strong, however; defense against predators may model fear responses better than interpersonal conflicts (Blanchard and Blanchard 1989). Nevertheless, one can still assume that in some cases, human conflicts elicit fears similar to those felt by rodents faced with predators, and the behavioral response depends neither on gender nor on testosterone. This assumption explains why aggression does not depend on testosterone in some cases. Additional theories are needed to explain cases where it does.
The Challenge Hypothesis
This hypothesis was put forward by Archer (2006) and is in fact a human extension of the hypothesis proposed by Wingfield et al. (1990) for monogamous birds. It was also influenced by a study in primates (Muller and Wrangham 2004). The hypothesis suggests that testosterone is increased by and promotes aggression under conditions of social challenge in a manner very similar to that seen in birds. It was also suggested that testosterone influences the trade-offs between momentary interests (sexual stimulation, competition, reputation, etc.) and parental investment: it promotes an extraverted, uninhibited, dominant personality that pursues shorter-term reproductive strategy, emphasizing mating rather than parental effort (Archer 2006).
The Developmental Hypothesis
The observation that neonatal testosterone injections increase adult aggressiveness in mice (Bronson and Desjardins 1968, 1969) was later followed by human studies demonstrating that the same happens in people (Auyeung et al. 2009; Bailey and Hurd 2005; Benderlioglu and Nelson 2004; Hines et al. 2002; Reinisch 1981; Yildirim and Derksen 2012a, b). These studies showed that all three prenatal androgenic treatments, high amniotic fluid testosterone, and low 2D:4D digit ratios (indicative of high fetal testosterone) increase aggressiveness later in life. Albeit not formally advanced as a hypothesis so far, these findings (replicated by an increasing number of studies) suggest that the long-term neural effects of early testosterone exposure make adult aggressiveness somewhat independent of testosterone secreted in adulthood. In other words, normal adult levels of this hormone promote aggression by virtue of sensitization produced during the fetal/neonatal period. Consequently, not adult, but perinatal levels of testosterone set the propensity to behave aggressively, for which the measurement of adult plasma levels may be misleading regarding the relationship between testosterone and aggression.
“Cofactor” Hypotheses
A series of findings suggest that the behavioral relevance of individual differences in testosterone production is increased or made noticeable by the co-occurrence of other conditions. Some of the authors adhering to this approach emphasize dual influences (testosterone-MAO A polymorphisms: Sjoberg et al. 2008; testosterone-plasma cortisol: Dabbs et al. 1991; Terburg et al. 2009; testosterone-noradrenaline, testosterone-serotonin, or testosterone-dopamine neurotransmission: Chichinadze et al. 2010; Gerra et al. 2007; Pavlov et al. 2012; Witte et al. 2009). The common denominator of such findings (some being formally incorporated into hypotheses; Terburg et al. 2009) is the assumption that while testosterone per se may have a minor impact, it makes a difference if associated with low cortisol production, low serotonin neurotransmission, etc. This hypothesis also explains contradictory findings and suggests that the role of testosterone may be understood in the context of another deficiency. Other authors assume that the role of testosterone can be understood in the context of multiple imbalances. Hypotheses of this kind suggest that testosterone is only one piece of a mosaic of features that lead to aggression. The most elaborated form of this hypothesis is the triple imbalance hypothesis of reactive aggression by van Honk et al. (2010), suggesting that reactive aggression is predicted by an imbalance between testosterone and cortisol secretion, cortical–subcortical communication, and left-sided frontal brain asymmetry that results from low serotonergic function. Although not elaborated to a comparable degree, the embedding of testosterone effects into a framework of multiple neural/endocrine deficits was suggested by a series of other authors (for examples, see, e.g., George et al. 2001; Mong and Pfaff 2003; Nelson 2005; Pavlov et al. 2012). These hypotheses suggest that testosterone is one of the multitudes of factors that contribute to the expression of aggression.
“Social Context Hypothesis”
Some authors assume that the effects of testosterone are strongly modulated by social factors (Booth and Osgood 1993; Dabbs and Morris 1990; Fang et al. 2009; Kreuz and Rose 1972; Sluyter et al. 2000; Soler et al. 2000). In these studies, socioeconomic status, cultural background, and family relationships affected the testosterone response to conflicts and also the interaction between testosterone and aggression. Noteworthy, social context affects the impact of testosterone also in animals (Gleason et al. 2009; van Anders et al. 2011), suggesting that the interaction between testosterone, aggression, and context is a general phenomenon.
Taken together, these theories suggest that the testosterone-aggression relationship cannot be expected to be linear. Studies where no association between testosterone and aggression was found may fall short at considering conditions that influence testosterone efficacy. In other words, negative findings may have turned out positive if conditions other than testosterone were also taken into account.
2.2.5 Testosterone and Abnormal Aggression in Humans
High testosterone levels were associated with both criteria based on which normal and abnormal forms of aggression are delimited in humans: rule-breaking violence and aggression-related psychopathologies. In the first large-scale study, a sample of 4,462 US military veterans showed a significant correlation between testosterone and a variety of antisocial behaviors (Dabbs and Morris 1990). In a series of subsequent studies, plasma testosterone levels differentiated normal controls from violent offenders as well as nonviolent offenders from violent offenders (Aluja and García 2007; Banks and Dabbs 1996; Brooks and Reddon 1996; Booth and Osgood 1993; Chichinadze et al. 2010; Dabbs and Morris 1990; Dabbs et al. 1991; Fang et al. 2009; Kreuz and Rose 1972; Popma et al. 2007a, b; Rasanen et al. 1999; Terburg et al. 2009; Virkkunen et al. 1994a, b). The relationship was moderated by a variety of conditions in some of these studies (cortisol: Dabbs et al. 1991; Terburg et al. 2009; Popma et al. 2007a, b; noradrenaline: Chichinadze et al. 2010; psychopathology: Rasanen et al. 1999; serotonin: Virkkunen et al. 1994a, b; sex hormone-binding globulin: Aluja and García 2007; social factors: Booth and Osgood 1993; Dabbs and Morris 1990; Kreuz and Rose 1972; Fang et al. 2009). Nevertheless, a role for testosterone in violent delinquency was clear, even if this role was not played alone or was not independent of other factors.
High testosterone levels were also found in adolescent boys showing externalizing problems (Maras et al. 2003), conduct or oppositional-defiant-disordered boys and girls (Pajer et al. 2006; Kirillova et al. 2008), antisocial personality-disordered adults (George et al. 2001; Lindberg et al. 2003; Rasanen et al. 1999; Sjoberg et al. 2008; Virkkunen et al. 1994a, b), borderline personality-disordered women (Roepke et al. 2010), as well as psychopathic patients (Glenn et al. 2011; Stalenheim et al. 1998; Terburg et al. 2009; Yildirim and Derksen 2012a, b). Again, these effects were moderated by a series of other conditions (alcohol dependence, MAOA polymorphisms, serotonin functioning, severe conduct disorder in adolescence, sex hormone-binding globulin in plasma, and social factors), but often showed correlations with symptom severity. In a few studies, increased adrenal androgen (androstenedione and/or dehydroepiandrosterone) rather than testosterone production was associated with conduct and oppositional-defiant disorder in children (Dorn et al. 2009; van Goozen et al. 1998a, b, 2000a, b). Taking into consideration, however, that these adrenal steroids are converted into testosterone and estrogens in the brain, these findings suggest alternative routes of brain androgen supply at early ages when the production of this hormone is low rather than negate the role of testosterone.
2.2.6 Overview
The question whether testosterone influences aggression can be broken down into several sub-questions, the answers to which are all affirmative: perinatal testosterone positively affects the development of brain mechanisms that control aggression; testosterone in adulthood promotes aggression; the mechanisms of abnormal aggression have a testosterone component; and all these mechanisms are operational in both animals and humans. The major question unanswered by these statements is why the association between testosterone and aggression is so weak when investigated in large human samples?
We propose the following explanations:
1.
The mechanisms mediating testosterone effects are complex, and changes in one variable may provide insufficient information on their functioning. For example, normal levels of testosterone production can easily promote aggression if the brain mechanisms mediating its effects are “over-primed” perinatally.
2.
While brain mechanisms involved in the control of aggression are upregulated by testosterone, decisions regarding behavior are not taken at hormonal levels. Testosterone does not activate brain mechanisms, but facilitates functions when particular brain mechanisms are activated by other factors, e.g., a social challenge. In this respect, we refer again to the theoretical paper by Archer (2006) and also to Fig. 2.1. The interaction between challenges and testosterone functions may explain why effects are clearer in animals than in humans. In animals, the role of testosterone is studied in animals that are socially challenged (e.g., in the resident-intruder test). Correlational studies in humans may include people living in challenging environments but also include subjects who lead lives rather free of aggressive challenges.
3.
The role of this hormone becomes especially conspicuous when associated with other mechanisms that promote aggressiveness (see, e.g., the triple imbalance hypothesis by van Honk et al. 2010). The activation of aggression-controlling brain circuitries by other factors inherently makes the roles of testosterone noticeable (see above). Interactions of this kind may explain why the role of testosterone is so strong in abnormally aggressive people who show multiple dysfunctions and so weak in healthy controls where testosterone is insufficient to activate aggression-bound brain mechanisms alone (Fig. 2.4).
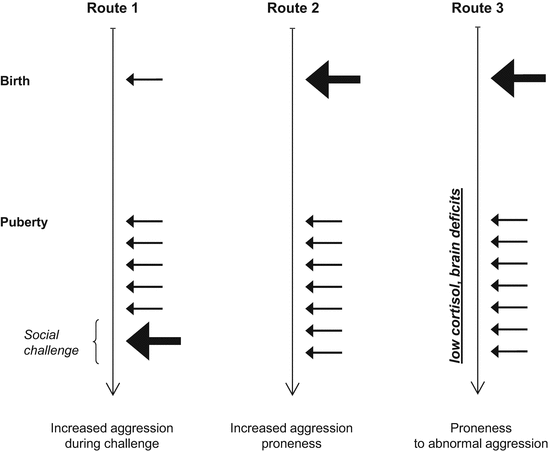
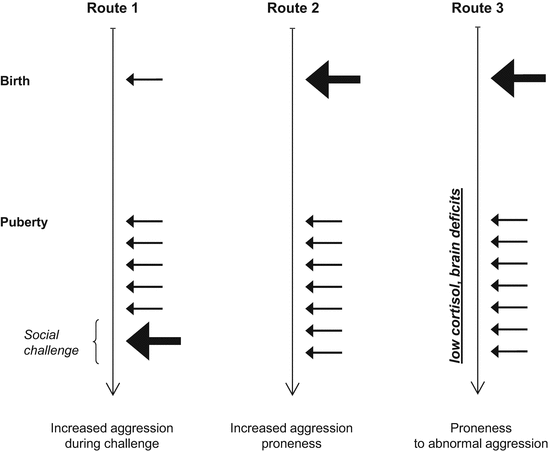
Fig. 2.4
Three theoretical rutes by which various forms of aggression may develop despite normal basal testosterone levels. The arrows indicate testosterone secretion; their length and thickness depicts the amount secreted. Route 1, challenge-induced increases in testosterone elevate aggressiveness temporarily (challenge hypothesis, Archer 2006); Route 2, increased prenatal exposure to testosterone elevates aggression-proneness overall; Route 3, a pattern similar to the previous one elicits abnormal forms of aggression when associated with other deficits (tripple inbalance hypothesis, van Honk et al. 2010). Note that testosterone influences aggression indirectly by affecting brain function
Taken together, the above considerations suggest that the role of testosterone should be evaluated in conjunction with the complexity of its mechanisms of action and in conjunction with other factors that effect aggressiveness.
2.3 Glucocorticoids
Glucocorticoids control a wide array of physiological functions from blood glucose to immunity and brain mechanisms. The diversity of its actions forecasts some difficulties regarding the evaluation of the glucocorticoid/aggression relationship. This difficulty is increased by the complex secretion patterns of these hormones and by the multitude of its cellular and subcellular mechanisms of action. Its effects are further complicated by the fact that glucocorticoid effects are highly time dependent; effects occur and vanish on a time scale of minutes to month. The general characteristics of hormone action also need to be kept in mind: glucocorticoids do not activate behaviors but affect brain function. For these reasons, the analysis of the glucocorticoid/aggression relationship should be carried out carefully: the complexities of both aggressiveness and glucocorticoids should be taken into account.
2.3.1 Mechanisms of Action
In principle, two types of glucocorticoid action were identified: rapidly developing and transient effects mediated by a variety of cellular mechanisms and more slowly developing but persistent effects mediated by the genome. The non-genomic mechanisms endow glucocorticoids with the ability to rapidly affect brain function, by stimulating the voltage-dependent Ca2+ channel and calcium trafficking in general, by binding to and affecting the function of various neurotransmitter receptors, by binding to various membrane and cytoplasmic proteins, and by affecting protein–protein interactions within the neuron (for recent reviews, see de Kloet et al 2008a, b; Groeneweg et al. 2011; Haller et al. 2008). Genomic effects develop more slowly (within hours, days, weeks, and even month) and consist of the activation or inhibition of gene transcription. Such effects are rather wide ranging because glucocorticoid responsive elements are frequent in the genome, and in addition, glucocorticoids regulate gene expression also posttranscriptionally (Datson et al. 2008; Ing 2005).
Beyond large differences in lag time and cellular mechanisms, the two types of action also differ in the glucocorticoid secretion patterns that activate them, and in their ultimate outcome. Non-genomic effects are activated by acute surges in plasma glucocorticoids and are behavior activating in nature: they aim at controlling the critical situation that triggered the stress response (de Kloet et al. 2008a, b; Koolhaas et al. 2011; Haller et al. 1998a, b). This is valid for aggression as shown below. Non-genomic effects by contrast are typically activated by persistent changes in glucocorticoid secretion; overall, such effects are behavior depressing in nature and promote survival by limiting risks and costs associated with behavioral activity (Haller et al. 1998a, b). This is valid for aggressive behavior as shown below.
The particularities of glucocorticoid action require that its role in behavior control necessitates the detection of both acute surges and persistent changes. The first task is relatively easy because the timing of surges is made obvious by events, while their magnitude is large. Note that aggression is among the strongest stressors (Koolhaas et al. 1997). Uncovering persistent changes is rather difficult because glucocorticoid secretion shows diurnal, ultradian, and circahoral secretory rhythms, which are identifiable in both blood plasma and saliva samples (Follenius et al. 1987; Stupfel and Pavely 1990; Trifonova et al. 2013). In addition, they rapidly respond to meals and minor stressors and in addition the anticipation of stressors (Gaab et al. 2005; Tornatzky and Miczek 1994). Consequently, plasma levels are highly variable, and the timing of peaks and nadirs is unpredictable. This problem can be overcome by repeated sampling that washes out the effect of oscillations, by studying the so-called awakening response and by obtaining urine samples over prolonged periods (e.g., 24 h) (Fig. 2.5). The phrase awakening response covers the relatively rapid surge in glucocorticoid secretion that follows awakening. This is rather free of environmental influences and is considered the most appropriate measure of HPA-axis basal activity (Kudielka and Wüst 2010).
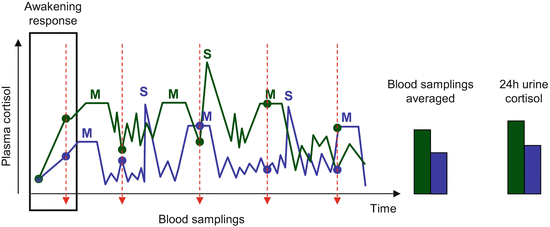
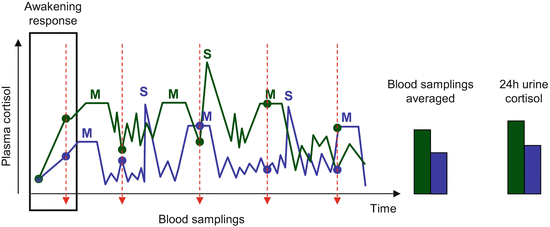
Fig. 2.5
The interaction between glucocorticoid secretion rhythms and glucocorticoid levels measured at particular time-points. Left-hand panel, an example on how the moment of blood sampling (red arrows) influence detected cortisol values (filled circles) in two individuals with clearly different cortisol secretion profiles (blue and green) depending on endogenous secretion rhythms, as well as minor stressors (S) and meals (M). Right-hand panel, Cortisol levels estimated by averaging multiple samplings and in urine collected over the whole day in the very same individuals. Note that variations during the night are poorly known, for which these were not represented on the graph
It is worth noting that persistent changes in secretion patterns are not restricted to the chronic stress response which involves a persistent increase in secretion. The possibility that the HPA-axis can chronically lower its activity together with the assumption that this condition leads to psychopathology was recognized by a study on violent antisocially disordered people (Virkkunen 1985) and received much attention in subsequent studies on abnormal forms of aggression. Compared to the conditions that chronically activate the HPA-axis, the factors that have the opposite effect are considerably less well known. This endocrine condition is generally attributed to early adversities (Caldji et al. 2000; Gunnar and Vazquez 2001; Saxbe et al. 2012; van Goozen et al. 2007). The same influences usually elicit chronic stress responses in most people, and only a minority of them develops the aforementioned reduction in HPA-axis function (Gunnar and Vazquez 2001). The distinctive features of this subgroup are unknown. Genetic predispositions, epigenetic phenomena triggered by gene–environment interactions, and the functional impairment of the adrenal cortex after birth complications or other dramatic stressors are suspected but unproven factors of developmental trajectories associated with chronic HPA-axis hypofunction (Rutter et al. 2006).
2.3.2 Normal Aggression in Animals
2.3.2.1 Acute Interactions
It was recognized very early that aggression is stressful and leads to glucocorticoid production in a variety of species (fish: Earley et al. 2006; lizards: Woodley et al. 2000; mice: Bronson and Eleftheriou 1965; quails: Ramenofsky 1985; rats: Schuurman 1980). In the rat resident-intruder test, the increase in glucocorticoids was similar in the two contestants at the beginning of the encounter, but values rapidly returned to basal levels in the winner while remaining high in the loser once dominance relationships were established.
Disparate early findings suggested that corticosterone secreted in response to the encounter might have an impact on ongoing behavior, as ACTH and corticosterone treatments increased aggressiveness while corticosterone synthesis inhibition decreased it (Brain et al. 1971; Bronson and Eleftheriou 1965; Munro and Pitcher 1985; Mainardi et al. 1987). Similar findings were obtained later in fish, where aggressiveness tightly correlated with corticosterone levels that preceded the aggressive encounter and acute treatment with glucocorticoid receptor antagonists decreased aggressiveness (Chang et al. 2012; Schjolden et al. 2009). In two parallel studies, Hayden-Hixon and Ferris (1991a, b) identified certain subregions of the hypothalamus as the main sites of the effect of corticosterone on attack behavior. In view of the fact that the hypothalamus is tightly involved in the control of biting attacks in all the species studied so far (Haller 2013), these findings suggest that corticosterone directly influences brain mechanisms that control attacks.
The existence of a “positive feedback loop” between aggression and HPA-axis activation was challenged in the early 1980s by the discovery of the genomic mechanisms of glucocorticoid action. The general assumption by that time was that glucocorticoids exert their effects only by the genomic mechanisms. As these mechanisms need time to take effect (at least 30 min but usually much more) and, in addition, the increase in glucocorticoids per se needs time (about 5 min), it was assumed that glucocorticoids cannot influence the aggressive acts that triggered their release, because by the time when plasma glucocorticoids increased and the genomic mechanisms were activated, most of the aggressive acts of dyadic encounters were already carried out. As a result, there remained nothing else to be influenced by glucocorticoids than submission, i.e., the consequence of losing the encounter. This belief was reflected in a series of studies, even in those where glucocorticoid synthesis blockade clearly decreased aggressiveness, and glucocorticoids increased aggressiveness (Munro and Pitcher 1985). Later on, a series of studies demonstrated that the rapid effects of glucocorticoids are real and several non-genomic mechanisms were also identified (de Kloet et al 2008a, b; Groeneweg et al. 2011; Haller et al 2008).
In a series of studies on the rapid interactions between glucocorticoids and aggression, we showed first that psychosocial encounters per se are sufficient to increase plasma glucocorticoids, i.e., the mere sensory contact with opponents is able to activate the HPA-axis (Haller et al. 1995). Similar findings were obtained in free-ranging animals as well (Landys et al. 2010; Silverin 1993). As aggressive encounters are preceded by a series of nonsocial and social behaviors that can be perceived as preparations for fights but are not aggressive themselves, these findings demonstrated that glucocorticoids are increased before the actual fights are started. In the following, we showed that natural variations in glucocorticoid synthesis, e.g., those related to ultradian and diurnal variations, are paralleled by changes in aggressiveness when rats are challenged in the resident-intruder test (Haller et al. 1998a, b, c, 2000a, b). Particularly, rats were significantly more aggressive in the increasing phase of their corticosterone fluctuation than their counterparts in the decreasing phase of their corticosterone fluctuations. Moreover, glucocorticoid enhancement-induced aggressiveness was normalized when the natural increase in glucocorticoid secretion was counteracted by glucocorticoid synthesis blockade or by glucocorticoid receptor antagonists. As the time frame of these hormonal and behavioral changes was less than 20 min, these findings indirectly supported the notion that glucocorticoids rapidly promote aggressiveness via non-genomic mechanisms. In the next step, we studied the interaction between glucocorticoid secretion and biting attacks that were elicited by the electrical stimulation of the hypothalamic attack area (Kruk et al. 2004). We found that the stimulation of the attack area dramatically increased corticosterone secretion while corticosterone decreased the electric threshold of attacks, i.e., facilitated the behavioral response. This suggested that there was a fast positive feedback loop between the adrenocortical stress response and brain mechanisms involved in aggressive behavior, the time frame of this interaction being approximately 10 min. Finally, we demonstrated that within 2 min, corticosterone administration restored aggressiveness that was downregulated by corticosterone synthesis inhibition (Mikics et al. 2004). This fast effect of corticosterone was resistant to protein synthesis inhibition, clearly demonstrating both the rapid aggression-promoting effect of glucocorticoids and the non-genomic nature of the mediating mechanism.
Taken together, the findings reviewed above show that (1) the HPA-axis is activated by encounters with unfamiliar individuals before the actual fights start and (2) glucocorticoids secreted in response to the encounter rapidly promote aggressiveness. Actually, aggressiveness is expressed concomitantly and under the supporting influence of glucocorticoids. In a subsequent study we showed that corticosterone does not affect aggressiveness in established colonies of rats, i.e., in colonies where the general level of aggressiveness is low and animals are not under the pressure of an acute social challenge (Mikics et al. 2007).
Thus, glucocorticoids rapidly and non-genomically promote aggressiveness specifically under conditions of a social challenge.
2.3.2.2 Chronic Effects
While acute surges in glucocorticoids promote aggressiveness in socially challenged animals, the effects of chronic increases in glucocorticoids are opposite.
As shown above, the aggressive encounter is stressful for both contestants; however, plasma glucocorticoids rapidly decrease after winning, but remain elevated in losers (fish: Höglund et al. 2002; lizards: Summers et al. 2003; mice: Bronson and Eleftheriou 1965; monkeys: Yodyingyuad et al. 1985; rat colonies: Blanchard et al. 1995; rat dyadic encounters: Schuurman 1980; snakes: Schuett and Grober 2000). In an experiment involving opponents with contrasting levels of aggressiveness, we showed that the effects of losing do not depend on the prefight status of individuals; plasma corticosterone levels were rapidly reduced in intruders that won the encounter, while the opposite happened in residents that were defeated in their home cage (Haller et al. 1996).
A series of studies show that elevated levels of glucocorticoids decrease aggression and promote submissiveness. This was demonstrated in a variety of species treated with glucocorticoids for prolonged periods (from 3 days to 3 weeks) (decreased aggressiveness in fish, lizards, birds, and rats: Meddle et al. 2002; Hayden-Hixson and Ferris 1991a, b; Overli et al. 2002; Tokarz 1987; increased submissiveness, fish, mice, and rats: DiBattista et al. 2005; Leshner et al. 1980; Hayden-Hixson and Ferris 1991a, b; reduction of home-range lizards: DeNardo and Sinervo 1994). Similar findings were obtained in experiments where subjects were exposed to stressors. Prolonged and strong single stressors (e.g., restraint for 2–6 h) or chronic social and nonsocial stressors decreased aggressiveness (Blanchard et al. 1995; Corum and Thurmond 1997; Wood et al. 2003; Yohe et al. 2012). The effects of post-encounter increases in glucocorticoids appear to be very strong and not necessarily related to the subjective experience of losing. For example, rats showed increased submissiveness if injected with corticosterone after an aggressive encounter on the previous day (Timmer and Sandi 2010). Thus, the primary mechanisms of losing are hormonal and not cognitive in rats.
Thus, durable increases in circulating glucocorticoids—which may or may not result from defeat experience—decrease aggressiveness and promote submissive behaviors.
2.3.3 Abnormal Aggression in Animals
Basal levels of glucocorticoids were not elevated in any of the abnormal models developed so far, which may not be surprising if one considers the consequences of chronic stress that were presented above. The glucocorticoid response to stress, however, was elevated in a number of studies, e.g., in the amphetamine, cocaine, instigation, early maternal separation, and postweaning social isolation models as well as in rats selected for low anxiety (Ansah et al. 1996; Fish et al. 1999; Moldow and Fischman 1987; Neumann et al. 2010; Toth et al. 2011; Veenema and Neumann 2009). The common feature of these models is that their subjects show exceedingly high levels of aggression. Other symptoms of abnormal aggression were investigated in one case only, namely, in the postweaning social isolation model, where increased glucocorticoid responses to aggression were associated with behavioral agitation, increased autonomic responses to aggression, and abnormal forms of attack (attack on vulnerable targets, deficits in social communication, and increased defensiveness on the background of increased attacks).
In another subgroup of models, either the basal levels of glucocorticoids were low or the glucocorticoid response to aggression was blunted (subjugation model: Ferris et al. 2005; anabolic steroid model: Rejeski et al. 1990; rats selected for high anxiety: Neumann et al. 2010; mice selected for high aggressiveness: Caramaschi et al. 2008a, b; Veenema et al. 2004). In the glucocorticoid dysfunction model, low basal glucocorticoid levels and blunted stress responses were experimentally induced (Haller et al. 2001, 2004). Where investigated, abnormal attack patterns were commonly seen; high attack counts were seen in some but not all models. No signs of behavioral agitation were observed, while autonomic responses to aggression or basal heart rates were diminished in those models where these were studied.
A third case is the pubertal stress model, where neither basal corticosterone nor the glucocorticoid stress response was altered, but the testosterone/corticosterone ratio increased, indicating a decreased corticosterone production relative to testosterone (Márquez et al. 2013).
The findings reviewed above demonstrate that abnormal aggression may be associated with either increased acute glucocorticoid responses to aggression or diminished HPA-axis functioning. The available data also suggest that subjects belonging to these two types of glucocorticoid/aggression associations also show differential emotional responses in terms of autonomic responses and may show some behavioral differences as well.
2.3.4 Healthy Human Controls
2.3.4.1 Acute Interactions
In the studies reviewed here, plasma cortisol was measured before and/or after the subjects performed a test where they executed aggressive acts. In this respect, these studies belong to that category of human studies which were called above “manifest aggression studies.”
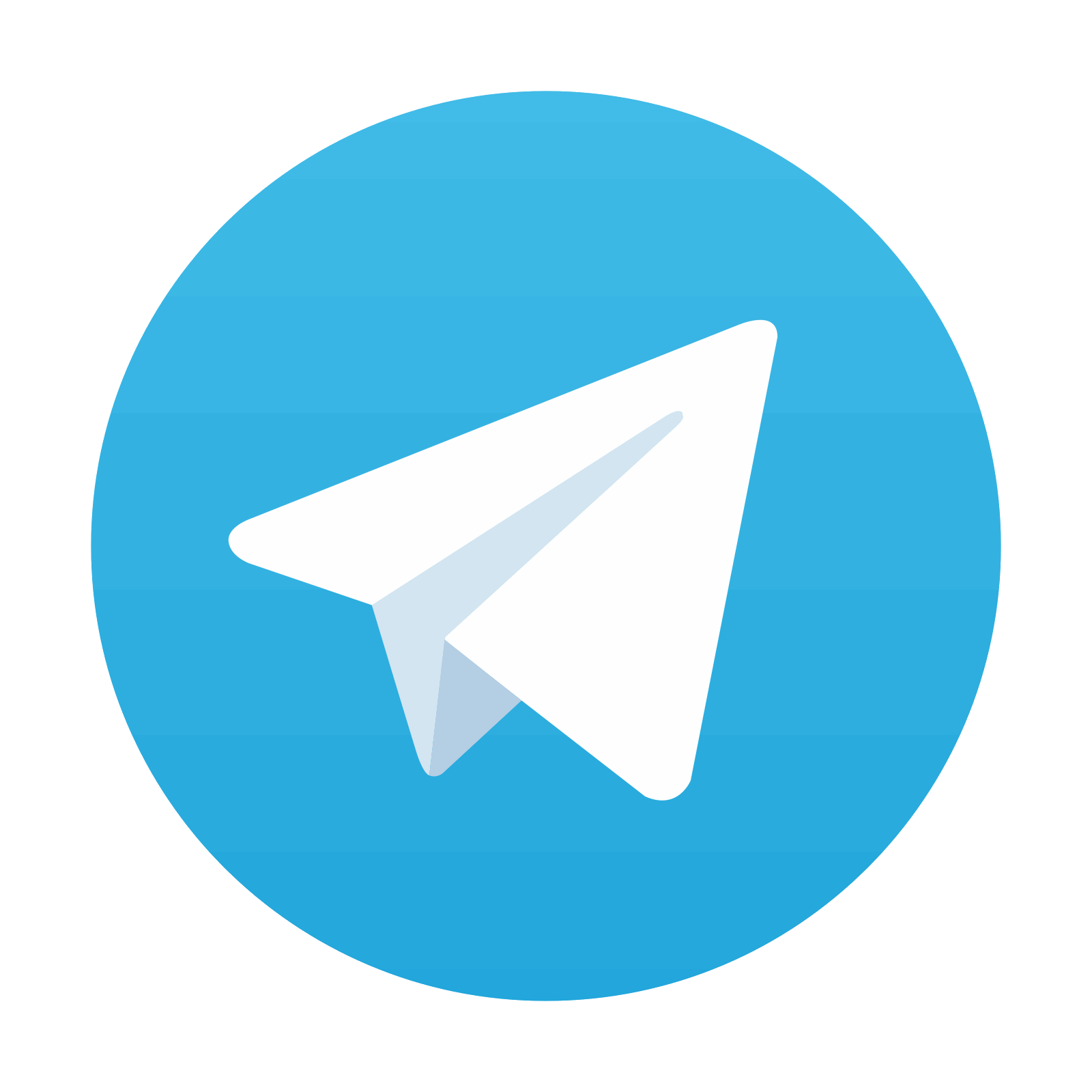
Stay updated, free articles. Join our Telegram channel

Full access? Get Clinical Tree
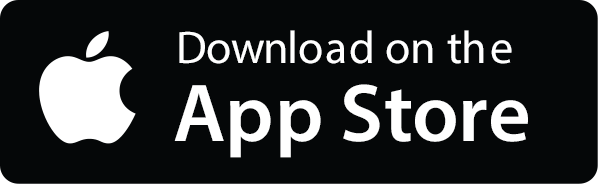
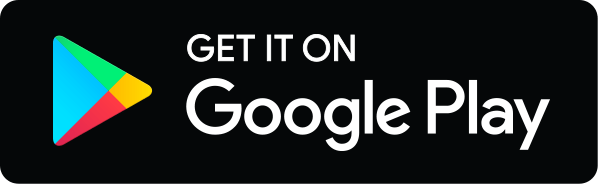
