Pooled, weighted Cohen’s d effect sizes reflecting the performance of currently depressed patients (black bars) and remitted depressed patients (gray bars) compared to healthy controls on tasks of executive function [OTS/SOC, (One Touch) Stockings of Cambridge; SWM, Spatial Working Memory; IED, Intra-Extra Dimensional Set Shift; SSP, Spatial Span], memory (DMS, Delayed Matching to Sample; PAL, Paired Associates Learning; PRM, Pattern Recognition Memory; SRM, Spatial Recognition Memory), attention (RVP, Rapid Visual Information Processing) and reaction time (RTI, Reaction Time). Error bars represent 95% confidence intervals (CIs).
Impairment in cold cognitive function can be debilitating. Working memory performance, for instance, is thought to be important for educational success (Friedman et al., 2006; Yuan, Steedle, Shavelson, Alonzo, & Oppezzo, 2006). Correlational studies support a link between both working memory and fluid intelligence and science achievement (Yuan et al., 2006). Indeed working memory at the start of formal education is a more powerful predictor of subsequent academic success than IQ (Alloway & Alloway, 2010). Most importantly, from a clinical perspective, working memory is affected in many neuropsychiatric disorders, including depression (Rock et al., 2014), attention-deficit/hyperactivity disorder (ADHD) (Martinussen, Hayden, Hogg-Johnson, & Tannock, 2005), and schizophrenia (Aleman, Hijman, de Haan, & Kahn, 1999). Identifying and targeting this impairment thus holds promise as a means of significantly improving outcomes in these disorders. In the CANTAB battery, working memory can be measured using the Spatial Working Memory Task in which the goal is to find a token under a series of boxes without returning to a location where a token was previously found. This measures a subject’s ability to hold spatial locations in memory. Patients with major depression show significant impairment on this task with a moderate Cohen’s d effect size of –0.54 (n = 806) (Rock et al., 2014). Functional imaging research has shown that in healthy individuals, such tasks recruit a neural network involving the dorsal regions of the prefrontal cortex (Owen, Evans, & Petrides, 1996; Owen et al., 1999). Exaggerated activation within this circuit is seen in unmedicated patients with depression (Harvey et al., 2005) which predicts poor response to fluoxetine (selective serotonin reuptake inhibitor (SSRI)) treatment (Graham et al., 2013; Walsh et al., 2007).
Behavioral effects in working memory are, however, also seen in remitted individuals (effect size –0.53, n = 214) indicating that the effect is dissociable from the negative mood state (Abas et al., 1990; Beats et al., 1996; Robinson & Sahakian, 2013; Rock et al., 2014). This, in turn, leads to the possibility that spatial working memory impairment may act as a “trait” measure of depression risk. That is to say, it may constitute a marker that is present before and after the disorder that indicates underlying ongoing susceptibility. Impaired cold executive functions, attention, and memory all appear to be present both during depression and in remission and may thus constitute such markers (Rock et al., 2014). Treatments that target this impairment will therefore have to target more than just the overt symptoms.
Hot cognition: negative affective bias in depression
Unlike cold cognitive processing, hot cognition involves emotional information. That is to say: positive, rewarding information about actions that should be repeated and negative, punishing information about actions that should be avoided. Indeed, there is often a conflict between risk and reward such that higher rewards might be achieved but at risk of greater punishments. A good example of this would be a gambling task in which the more money staked, the greater the potential gain and potential loss. Hot cognitive functions are also frequently time limited – a decision has to be made as quickly as possible. In the extreme this is illustrated by fight or flight mechanisms where an individual must decide how to tackle an immediate threat.
Patients with depression demonstrate “negative affective biases” on such hot tasks (Harmer et al., 2011; Robinson & Sahakian, 2013; Roiser et al., 2012). Specifically, they show a bias toward processing negative information manifest as, for instance, reduced reaction time to identify sad relative to happy words on the affective Go/NoGo task (Erickson et al., 2005). Healthy subjects, by contrast, often show the opposite pattern – a bias toward positive information (Erickson et al., 2005; Robinson, Cools, & Sahakian, 2012). These negative biases in depression likely serve to both promote and uphold the depressive mood state. Specifically, as recognized by Beck in his early cognitive model (Beck, 1967), increased recall of negative at the expense of positive life events will serve to reinforce the depressed mood and trigger a negative feedback loop. Neurally, such negative affective biases have been linked to altered responses in a wide range of brain regions, from subcortical regions such as the striatum and amygdala to ventromedial and lateral frontal cortical regions (Eshel and Roiser, 2010). In contrast to the cold working memory effects outlined above, negative biases have been shown to exist in the current depressive state but may disappear in remission (Harmer et al., 2011; Maalouf et al., 2012; Robinson & Sahakian, 2013; Roiser et al., 2012). Thus, hot cognitive change may act as a “state” biomarker that tracks the presence/absence of symptoms (Pulcu et al., 2014). For example, a negative view of the future (temporal discounting, or devaluing, of future vs. current rewards) is present in patients undergoing a current episode but normalizes on remission (Pulcu et al., 2014). Indeed, patients who have responded to antidepressants can perform indistinguishably from healthy controls on hot cognitive tasks (Harmer, 2008; Harmer et al., 2009b).
It should be noted that altered hot cognitive function can also be seen on tasks which do not require responses to be made directly to emotional stimuli. Negative biases can also be seen on ostensibly “cold” cognitive tasks if subjects are also provided with feedback. Depressed individuals demonstrate what has been referred to as a “catastrophic response to negative feedback” (Elliott, Sahakian, Herrod, Robbins, & Paykel, 1997; Elliott et al., 1996) – i.e. their performance, which may have previously been good, collapses when they make a mistake. This is particularly evident on tasks in which subjects are provided with misleading negative feedback. For instance, on probabilistic reversal-learning tasks, subjects are asked to select the one of two stimuli which is “winning” in the face of misleading feedback. Depressed patients are very sensitive to this feedback, switching their responses as soon as they receive misleading feedback (rather than waiting for confirmation like healthy controls) (Murphy, Michael, Robbins, & Sahakian, 2003; Murphy et al., 2001; Taylor-Tavares et al., 2008). A similar pattern is seen on the one-touch Tower of London planning task, in which depressed subjects’ performance deteriorates after they make a mistake (real or misleading). It has been argued that this “choking” response is driven by a failure of “top-down” control over emotional responses (Elliott et al., 1996, 1997). Importantly, similar effects of negative biases and increased response to negative feedback were seen in adolescents with first-episode depression and at risk for depression (Kyte, Goodyer, & Sahakian, 2005). At the neural level this may arise due to inefficient control over the amygdala by the dmPFC and ventrolateral prefrontal cortex (VLPFC) in patients (Taylor-Tavares et al., 2008). Such observations have led to a refined model of treatment for depression in which cognitive treatments are thought to target higher-level (perhaps cortical) control, which phenomenologically may correspond to altered evaluation of negative events, whilst antidepressants target lower-level bottom-up emotional processing in, for example, the amygdala (Figure 6.2).
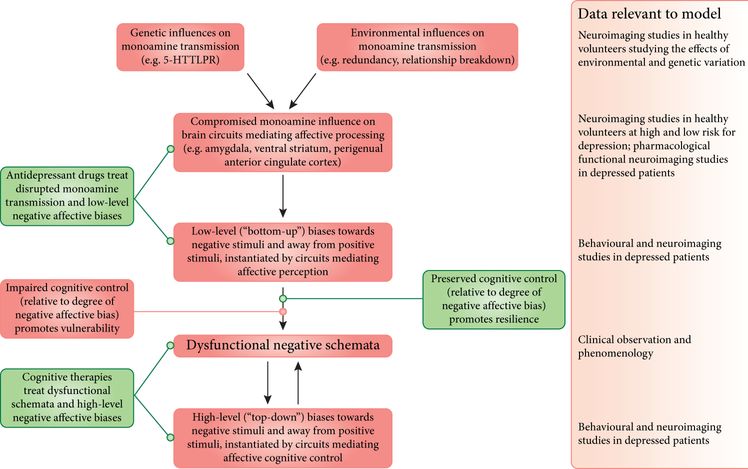
Dark gray boxes indicate factors contributing to the development and maintenance of depressive symptoms. Light gray boxes indicate factors contributing to the treatment of and recovery from depression. 5-HTTLPR: serotonin transporter-linked polymorphic region.
Biomarkers: early detection and treatment of depression using cognitive measures
Early detection of depression is critical because the longer an individual remains within an episode, and the more recurrent episodes an individual suffers from, the worse the clinical outcomes. Specifically, untreated depression is associated with: (1) poorer response to antidepressants; (2) lower rates of remission; (3) higher risk of chronicity; and (4) a higher number of recurrences (Ghio et al., 2014; Robinson & Sahakian, 2008).
As such, biomarkers that allow us to catch depression sooner than full-blown symptoms develop would be enormously beneficial. Biomarkers can constitute a wide range of observations from genetic and neuroimaging to blood-based measures (e.g. recent work has identified early morning cortisol as a stratified, population biomarker in boys with high depressive symptoms (Owens et al., 2014)). However, altered cognitive changes specific to depression risk highlight the possibility of a non-invasive means of identifying early onset of depression. In one recent study, for instance, it was shown that individuals who possessed two “s” alleles of the serotonin transporter-linked promoter region (5-HTTLPR; a genetic polymorphism associated with depression risk) and suffered early psychological abuse – but who, critically, were not currently depressed – showed negative affective biases and oversensitivity to negative feedback on cognitive tasks (Owens et al., 2012). When these adolescents were followed up a year later, a relationship was found between these cognitive biomarkers and emotional disorders. Similar patterns are seen in healthy first-degree relatives of patients with bipolar disorder (Clark, Sarna, & Goodwin, 2005; Olvet, Burdick, & Cornblatt, 2012) and negatively biased neural responses in highly neurotic (but never-depressed) healthy individuals can be normalized using antidepressant treatment (di Simplicio, Norbury, & Harmer, 2012). Given that this was seen in healthy individuals, albeit with subclinical symptoms, it highlights the possibility of cognitive testing for the detection of early depression risk. Thus, it may be possible to assess a wide range of symptoms in at-risk individuals, monitor the changes in these symptoms, develop an individualized profile of depression risk, and target appropriate interventions sooner. As highlighted above, trait and state markers may have different uses in this endeavor; state markers determining the presence or absence of the depressed state; trait makers detecting underlying risk (Robinson & Sahakian, 2013).
Use of technology for detecting and monitoring cognition in depression
It should also be noted that one goal of standardized computerized cognitive batteries is to improve access to cognitive biomarker assessment across medical specialties. Large numbers of depressed patients are thought to be missed by their general practitioner (GP) in their first-line encounter with treatment (Ormel, Koeter, van den Brink, & van de Willige, 1991; Paykel & Priest, 1992). Standardized testing batteries of cognitive biomarkers might be able to provide a GP three simple outcomes: (1) “no concern”; (2) “a need to monitor”; or (3) a “need to investigate” depression-related cognitive functions, thereby providing a more objective measure of when to refer for further treatment. Moreover, once a patient is diagnosed, standardized assessment could be used for “deep profiling” within the clinic, or for continuous monitoring of biomarkers at home using, for example, smartphones.
Future directions
Conventional antidepressants tend to take a minimum of two weeks to take effect on mood (despite potentially rapid effects on negative biases) (Harmer, Goodwin, & Cowen, 2009a; Harmer et al., 2009b). However, there has been a recent push to explore the use of alternative medications that may have more immediate effects on mood state, such as ketamine (Zarate et al., 2006). Moreover, as described above, some cognitive impairments, particularly cold cognitive impairments, persist into remission with standard medications and treatments. These residual traces may ultimately contribute to continued vulnerability (Robinson & Sahakian, 2008) and as such, interventions that ameliorate these symptoms would be particularly valuable. In this final section we highlight some non-conventional depression treatments which may either induce more rapid improvement in mood and hot cognitive functions or target cold impairment.
Modafinil
Modafinil (Provigil) is a treatment which may target underlying cold cognitive impairments. Modafinil’s mechanism of action is unclear, but recent evidence suggests that its actions may be multimodal, altering noradrenaline, dopamine, and glutamatergic mechanisms (Scoriels, Jones, & Sahakian, 2013). At the cognitive level modafinil improves working memory in healthy individuals (Müller et al., 2013; Scoriels et al., 2011) alongside an increase in overall task-related motivation (i.e. it improves cold cognitive function). In addition to these cold cognitive effects, modafinil may be of clinical value. In first-episode psychosis modafinil has been shown to improve recognition of emotional faces (Scoriels et al., 2011); and a recent meta-analysis (of three studies) showed that adjunctive modafinil at doses of 100–400 mg a day significantly improved depression severity scores in patients with MDD (Goss et al., 2013; Scoriels et al., 2013). More work is necessary to disentangle these effects, but modafinil shows promise as a potential means to tackle cold and hot cognitive deficits, perhaps in combination with conventional antidepressants (Goss, Kaser, Costafreda, Sahakian, & Fu, 2013).
Methylphenidate
Methylphenidate is a stimulant that acts via inhibition of dopamine and noradrenaline reuptake (Mehta et al., 2000). Its primary clinical use is in ADHD treatment but in healthy individuals it enhances spatial working memory by promoting load-related activity in the fronto-parietal working memory network (Mehta et al., 2000). Moreover, there are some data to suggest that it may be effective at combating depression symptoms (Hardy, 2009). Again, this might be explored as a potential treatment for cold cognitive impairment in depression.
Vortioxetine
Vortioxetine has a combination of direct effects on receptor activity and serotonin (5-HT) reuptake inhibition. Specifically, in vitro studies show that vortioxetine is a 5-HT3, 5-HT1D, and 5-HT7 receptor antagonist, 5-HT1B receptor partial agonist, 5-HT1A receptor agonist, and a 5-HT transporter inhibitor (Bang-Andersen et al., 2011; Mørk et al., 2012). It was approved for use in the USA in 2013 and scheduled for UK launch in early 2015 (NICE, 2014). Vortioxetine may also be effective in treating cold cognitive impairment. In one study it improved psychomotor slowing and word list learning on the Digit Symbol Substitution Test in elderly depressed patients (Katona, Hansen, & Olsen, 2012). Moreover, both 10 and 20 mg doses improved cold cognition across memory, executive function, attention, and general response speed (McIntyre, Lophaven, & Olsen, 2014). Critically, it also improves depressive symptoms, with effects of 20 mg emerging within a week (McIntyre et al., 2014). Thus, vortioxetine may target cold cognitive effects as well as mood effects at a faster speed than standard SSRIs (which usually take two weeks).
Ketamine
Ketamine is an NMDA receptor antagonist. In contrast to the majority of NMDA antagonists, ketamine serves to increase glutamate transmission in the prefrontal cortex via blockade of tonic firing of GABAergic interneurons (Duman & Aghajanian, 2014). This results in a “burst of glutamate” and contributes to activity-dependent release of BDNF and increased synaptogenesis. In one of the first randomized control trials of ketamine carried out by Zarate and colleagues, subjects receiving ketamine (0.5 mg/kg) showed significant improvement in depressed mood symptoms (relative to placebo) within 110 minutes after injection. Most importantly, this mood effect remained significant throughout the following week (Zarate et al., 2006). Ketamine is, however, associated with cold cognitive impairment in both healthy volunteers at sub-anesthetic doses (Malhotra et al., 1996) as well as in current recreational ketamine users (Tang, Liang, Lau, Tang, & Ungvari, 2013) and it is thought to be the dissociative effects of ketamine (as measured by the Clinician-Administered Dissociative States Scale (CADSS)) that lead to this antidepressant effect (Luckenbaugh et al., 2014). An intriguing possibility is using a brief initial course of ketamine to lift depressed mood and allow patients to begin engaging in psychological treatment, perhaps combined with long-term use of standard antidepressants.
Deep brain stimulation
Early imaging work implicated rostral and ventral regions of the cingulate cortex, referred to as the subgenual anterior cingulate, in depression (Drevets et al., 1997; Kennedy et al., 2001). This increased activity was subsequently linked to hot cognitive processing (Elliott, Rubinsztein, Sahakian, & Dolan, 2000) and was shown to normalize following a course of antidepressants (Kennedy et al., 2001). This formed the basis of the current attempts to use deep brain stimulation to treat depression. Direct stimulation of the subgenual anterior cingulate has been shown to improve functional outcomes in depression in open-label trials but would only be recommended for particularly resistant cases (Kennedy et al., 2011).
Conclusions
In this review we have provided a narrative overview of the cognitive alterations in depression; encompassing hot and cold changes; and how they may have value as biomarkers. We have also highlighted some potential novel interventions for these symptoms. We would emphasize, however, that complete treatment of depression will require a holistic approach to prevent depression from becoming debilitating, chronic, and relapsing. Early detection is critical, an effort which standardized computerized cognitive screening in general practices may help. The earlier it is possible to catch the disorder, the better the likely outcomes (Sahakian, 2014). Critically, however, once detected it is important to focus on all aspects of the disorder – not simply the mood state, but also cognitive function, well-being, and integration back into society, with patients taking an active role in their treatment (Insel et al., 2012; Sahakian, 2014). Indeed, it may be fruitful to emphasize the “active” manner of treatment for depression; a patient’s outcome may be better if they are able to fully engage with treatment. For example, treatments may include not only pharmacological and psychological components, but also cognitive training and exercise components. To this end, rapid-acting antidepressants (such as ketamine) may prove valuable at the outset of treatment to enable patients to reap the benefit of later cognitive or pharmacological treatments.
In summary, to improve functional outcome, quality of life, and well-being, cognition is an important target for treatment in depression. Future work should seek to establish reliable cognitive biomarkers as well as explore the impact that both standard (e.g. SSRI or cognitive behavioral therapy (CBT)) and novel treatments have on these markers. Moreover, future work should seek to understand the mechanistic cause of these alterations and so help develop future, as yet undiscovered, interventions (Insel & Gogtay, 2014; Insel et al., 2013).
Disclosures
Barbara Sahakian consults for Cambridge Cognition, Servier, and Lundbeck. She holds a grant from Janssen/J&J. She has share options in Cambridge Cognition. Jonathan Roiser consults for Cambridge Cognition and has participated in a media advisory board for Lundbeck. Oliver Robinson declares no conflict of interest.
References
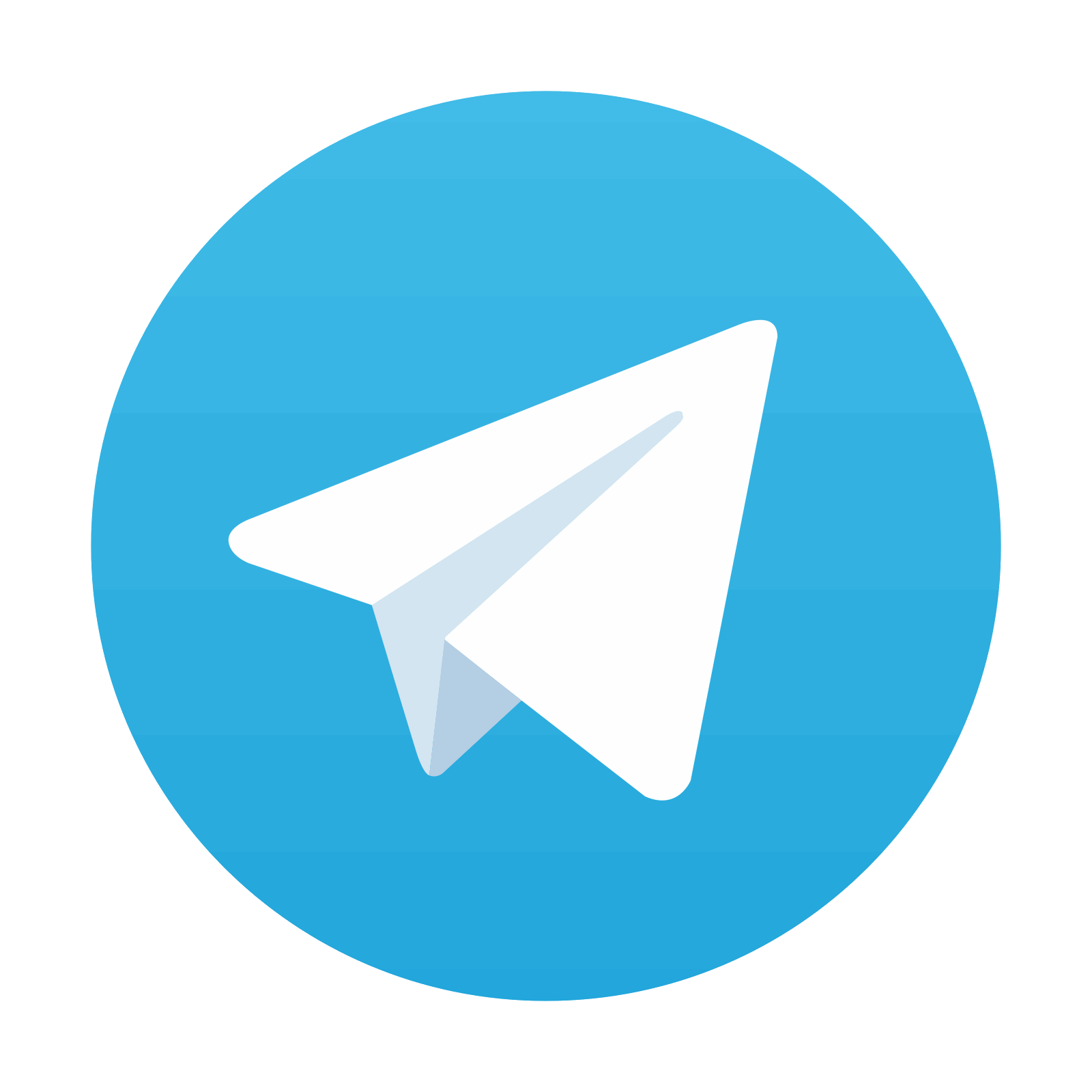
Stay updated, free articles. Join our Telegram channel

Full access? Get Clinical Tree
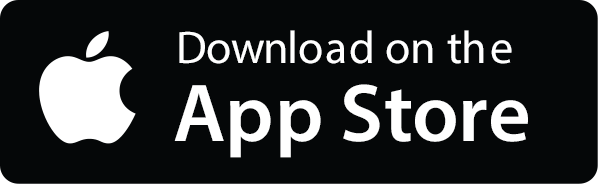
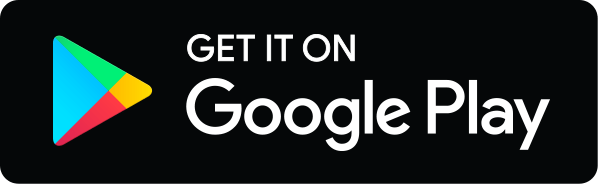