14 How to Achieve a Successful Fusion with MIS Techniques
Summary
Keywords: biologics bone graft extenders bone morphogenic protein BMP MIS TLIF
14.1 Introduction
Spine fusion or arthrodesis is a critical aspect of many spine surgical procedures. As minimally invasive techniques have increased in popularity and have been shown to provide better immediate postoperative outcomes, their use will not positively affect patient clinical outcomes unless the surgeon can achieve a successful and stable arthrodesis. Failure of fusion can result in severe clinical symptoms and pseudoarthrosis rates in spine surgery have been reported up to 30% of lumbar spine fusions.1 Despite the advent of improved rigid internal fixation, fusion promoting materials such as bone morphogenic protein (BMP), and improved surgical techniques, pseudoarthrosis still remains a significant cause of negative patient outcomes and results in higher medical costs, morbidity, and rates of revision surgery. Achieving a successful fusion requires a balance of biological, technical, and biomechanical factors including an optimization of host factors, low-strain mechanical environment, vascularization of the fusion bed, osteoinduction, osteoconduction, osteogenesis, and appropriate surgical technique for graft bed preparation.
14.2 Host Factors
It is imperative for the surgeon to recognize that successful fusion, regardless of surgical technique, is dependent on multiple factors. Patient factors such as age, bone density, metabolic disorders, hormonal imbalance, nicotine and smoking use, and comorbidities are equally as important as technical surgeon-controlled factors in achieving a successful union. Regardless of open versus minimally invasive surgery (MIS), these factors must be examined and optimized preoperatively by the operating surgeon in order to achieve a successful arthrodesis. Osteoporosis, for example, should be suspected especially in elderly women and treated accordingly before surgery.
Interbody fusion is the “sine qua non” when arthrodesis is pursued via MISS techniques. The use of powerful biologics to improve fusion rates will not obviate the need for careful and thorough discectomy, endplate preparation, and adequate carpentry of the implant.
14.2.1 Biomechanics
Ideal biomechanics are essential to successful arthrodesis in spine surgery. A low-strain environment combined with solid internal fixation and instrumentation that allows load sharing between host and graft tissues are critical principles.2 Pedicle screw constructs have now been established as the gold standard for rigid internal fixation in the thoracolumbar spine and have shown significantly higher successful arthrodesis rates when compared with noninstrumented fusions.3,4,5 MIS fixation techniques include navigated and percutaneous pedicle screw placement to avoid the traditional open soft-tissue dissection and are outside of the scope of this chapter but are critical for achieving rigid fixation in minimally invasive lumbar fusion.
14.2.2 Biologics
In achieving a successful fusion, the surgeon must not only optimize biomechanical considerations of low-strain and rigid fixation, but also must create an environment conducive to osteogenesis across a motion segment. Various biologics can be added to the fusion bed to aide in this process. The principles of osteogenesis require osteoconduction, osteoinduction, and osteogenesis. Osteoconductivity refers to the ability of graft material to act as a scaffold or matrix to support osteoblasts and osteoprogenitor (OPG) cells. Osteoinduction refers to the ability of a graft to signal and induce osteoblastic differentiation of OPG stem cells. Osteogenic potential of a graft refers to the ability of a graft to directly provide bone-forming cells to the fusion bed. All biologics have at least one if not more of these properties with the ideal graft combining all of these aspects.
The traditional gold standard for spine fusion has long been iliac crest bone autograft (ICBG) as it combines cancellous bone, structural cortical bone, and autogenous bone marrow cells. However, due to finite supply and patient morbidity, its utility in spine fusion procedures has steadily decreased given up to a reported 30% complication rate including pain, infection, hematoma, and neurovascular injury.6,7,8,9,10,11,12 Minimally invasive procedures in particular aim to limit patient morbidity and soft-tissue dissection, and have made ICBG nearly prohibitory for use.
Local autograft, while more limited in quantity in minimally invasive procedures when compared with traditional open surgery, is still an integral part of obtaining a successful fusion and should be maximized in quantity during any fusion procedure. The surgeon should make all attempts during decompressive surgery to preserve, collect, and reuse as much local autograft as possible given its potential for osteoinduction, osteoconduction, and osteogenesis. Particularly in minimally invasive transforaminal lumbar interbody fusion (MIS TLIF), ample amount of local autograft can be obtained through careful surgical technique. Specific techniques to the preservation of autograft include minimizing burr use, using a Lukens trap (Fig. 14.1) to collect bone shavings, preserving bone chips from decompression performed with a Kerrison rongeur, and cutting both the inferior and superior articular processes with an osteotome, and removing them whole to be used as a significant source of structural local autograft.13 Local bone shavings have been shown to have preserved histological properties with no significant damage at a cellular level to potential osteogenic cells; however, they likely have inferior osteogenic potential when compared with local autograft bone chips obtained from Kerrison rongeurs.14,15 Kasliwal et al used isolated local autograft collected with a Lukens trap during MIS TLIF in a retrospective series with a 67% fusion rate in 40 patients with a 1-year follow-up assessed with thin-cut computed tomography (CT) scan.16
Fig. 14.1 Lukens trap used for collection of bone shavings during minimally invasive lumbar fusion procedures.
Bone allografts function as bone extenders to help aide in fusion in cases without adequate autograft. Their benefits have been well described in the literature and include elimination of donor site morbidity and ample supply, but they are not without risks including disease transmission and antigenicity that can induce host reactions.17 Various alternatives to autograft exist: demineralized bone matrix (DBM), ceramics, composite grafts, BMPs, and mesenchymal stem cells (MSCs). Careful graft selection is critical for a successful outcome in fusion and an understanding of the variety of graft materials is an important consideration for the surgeon during minimally invasive procedures.
14.2.3 Allograft
Cadaver allograft is available in both a structural and nonstructural varieties. It is processed after harvest to reduce antigenicity as well as disease transmission; however, this eliminates their osteogenic potential. Advantages include abundance of supply and avoidance of autograft donor site morbidity. Disadvantages include disease transmission risk, however this has been reduced to less than 1 in 1.5 million. They are an osteoconductive scaffold and weakly osteoinductive, and when used in isolation in a recent meta-analysis obtained a collective fusion rate of 52% in posterolateral lumbar fusion.18
DBM is obtained by acid extraction demineralization of allograft bone. This process results in a matrix of isolated Type 1 collagen and noncollagenous proteins and growth factors that may have osteoconductive properties and potentially weak osteoinductive properties while sacrificing structural support. DBMs are available commercially in many forms including moldable putty, injectable gel, and flexible strips. There is a wide variety of osteoinductive qualities between graft vendors and even lot numbers that has previously been well described.19,20 This variability leads to limitations in their osteoinductive capabilities. Their isolated use for fusion is also not recommended as they require combination with mechanically resistant materials to avoid migration or excess motion.21 However, they do have a strong track record of success as a bone graft extender when combined with autograft.22
14.2.4 Ceramics
Ceramics such as calcium carbonate and beta-tricalcium phosphate provide osteoconductive scaffold for new bone formation but lack osteoinductive and structural support qualities. Hydroxyapatite coating of ceramic grafts may promote bony ingrowth and slow resorption rates.23 Advantages of ceramics include synthetic nature resulting in unlimited supply and lack of antigenicity or disease transmission risk. Disadvantages include brittle structure and requirement of supplemental fixation to protect against excess forces. In addition, they require large surface areas of decorticated bone for success.24 Their routine isolated use for lumbar arthrodesis has been attempted with some success; however, acceptable fusion rates with ceramics in the lumbar spine have been described when used in combination with bone marrow aspirate and local bone graft.25 A recent meta-analysis of ceramics demonstrated an 85% fusion rate in posterolateral lumbar fusion when combined with an osteoinductive source of autologous cells.18 Further study is needed into their use as an isolated bone graft substitute.
14.2.5 Bone Marrow Aspirate
Bone marrow aspirate allows for collection and concentration of growth factors and MSCs which have osteogenic potential due to their ability to differentiate into OPG cell lines. Their osteogenic properties were first described by Burwell in 1964.26 It is readily available and can be harvested percutaneously from the posterior superior iliac spine (PSIS; Fig. 14.2) among other sites of the body. Selective cell retention technology can be used to increase the number of OPG cells by three to four times.27 Bone marrow aspiration (BMA) does require a osteoconductive carrier or scaffold to obtain optimal fusion mass.
Fig. 14.2 Bone marrow aspiration from the posterior superior iliac spine.
In combination with local autograft, BMA has been shown to be a viable alternative to ICBG autograft with similar fusion rates in open posterolateral spine fusions.28,29 Equivalent clinical outcomes and fusion rates with BMA combined with a collagen carrier compared with ICBG and BMP-2 in single-level revision posterolateral lumbar fusion has also previously been demonstrated.30 A combination analysis of 2 studies totaling 40 patients undergoing posterolateral fusion resulted in 85% fusion rate when a concentrated BMA protocol with osteoconductive ceramic or allograft was used.18
Some disadvantages of BMA include: variations in the osteoblast colony-forming units per individual, inability to extract more than 2 mL per location due to dilution in blood, and unclear evidence for clinical advantages of centrifugation and concentration.31,32,33
14.2.6 Bone Morphogenic Protein
BMP is an osteoinductive growth factor first described by Urist in 1965.34 It has been commercially available in its recombinant form since 2002 and provides significant osteoinductive capabilities to promote spinal arthrodesis in multiple human studies.35,36,37,38,39,40,41,42,43 It has been well described for both primary and revision lumbar fusion surgery for its benefits in environments with limited local autograft.30,44
While it has significant osteogenic potential, it lacks structural and osteoconductive ability and requires combination with a carrier matrix to prevent dissolution from the operative site. It is commercially available in multiple forms; however, the most commonly utilized is rhBMP-2, which has been approved by Food and Drug Administration (FDA) for use in anterior lumbar interbody fusion procedures. Use of rhBMP-2 in the posterior lumbar spine is physician-directed and not FDA approved. However, there is evidence suggesting that rhBMP-2 produces better clinical and radiographic results than allograft alone, and has equivalent or better fusion rates and clinical results when compared with ICBG, with fusion rates as high as 94 to 100% being reported in the posterolateral lumbar spine.18,36,45,46 Despite the clinical evidence supporting its use to promote fusion, there have been several reported complications.
BMP Complications
Several complications have been reported regarding rhBMP use.
Temporary ipsilateral postoperative inflammatory radiculitis has been seen in up to 11% of patients undergoing MIS-TLIF with rhBMP-2 use.47 All resolved in 6 weeks with nonoperative treatment. It has been theorized to occur due to the chemotactic and proinflammatory characteristics of BMP that make it such an effective osteoinductive factor.48 While a known complication of TLIF without BMP, there is a suggestion that there is a higher rate of radiculitis in patients undergoing TLIF with BMP use.49
Aggressive, early vertebral body osteolysis has also been described with use of BMP in the interbody space in lumbar surgery. It has been seen in up to 44 to 69% of operated levels undergoing TLIF procedures with rhBMP-2 and resulted in both significant back pain (often treated conservatively) as well as graft migration and pseuodarthrois.50,51 It has been theorized that this osteolysis and resorption is a part of the normal osteoclastic remodeling process that is amplified by BMP; however, it has been associated with both spontaneous resolution as well as cage migration, subsidence, and nonunion.48,52 Overstuffing of the joint, BMP dosing, L5-S1 level with high inclination, and endplate violation have all been proposed as possible causes of the excess osteolysis seen in these patients.51
Excess and heterotopic bone formation resulting in neural compression has also been described in relation to BMP-2 use in the lumbar spine. It has been attributed to BMP leaking from the intervertebral space into the epidural space and resulting in either canal or foraminal compression.53 Cage placement within 2 mm of the posterior vertebral cortex was associated with excess extradiscal bone formation with BMP-treated patients undergoing posterior lumbar interbody fusion (PLIF).40 A systemic review has identified the rate of extradiscal heterotopic bone formation associated with BMP use of approximately 8%. Whether or not these patients required reoperation due to heterotopic bone formation is not as clear.51
De novo cancer as a result of BMP use has also been described in the literature by the Yale Open Data Access project with nearly a double risk of development of cancer in patients receiving rhBMP-2.54 Contradictory studies have demonstrated no significant increase in cancer rates.55,56 A conclusive link between BMP and de novo cancer rates has yet to be established.
Additional complications such as postoperative wound seromas, extradural cyst formations, and anterior cervical swelling and dysphagia have additionally been described.51
BMP Dosing
Many efforts have been made to counteract some of the negative aspects of BMP including reduced and low dosing BMP. Most studies describing the use of BMP in the interbody space describe typical BMP use of 4.0 to 12.0 mg/level.40,47 However, many authors have purported that decreasing BMP dosing can still achieve successful fusion in order to mitigate the potential side effects of vertebral osteolysis and ectopic bone formation in low levels.51 Optimal dosing for BMP use is yet to be defined.
14.3 Interbody Fusion
In the past three decades, circumferential fusion utilizing the interbody space in the lumbar spine has had increasing popularity in degenerative conditions. Purported benefits include increased surface area for bony fusion. The interbody space can be accessed from the anterior, lateral, and posterior approaches. Specifically, MIS for the posterior lumbar spine via a transforaminal approach (MIS TLIF) has gained popularity due to its potential for improved short-term patient outcomes when compared with open TLIF.57,58,59,60 Advantages include decreased estimated blood loss, shorter length of stay, and avoidance of the morbidity seen with both anterior and minimally invasive lateral trans-psoas procedures for interbody fusion.
The axis of rotation in flexion and extension within the lumbar spine is at the anterior aspect of the disc space. Limiting motion as close to this axis as possible limits the length of the lever arm of forces against the fusion location.2,61 Increased distance from this lever arm creates increased distractive forces and can predispose to pseudoarthrosis. Interbody fusion provides increased bony surface areas and compression loading across grafts to aide in fusion.62 Wolff’s law states that bone develops in response to mechanical stress and interbody fusion helps allow for fusion across a region of the spine that is loaded in compression as opposed to tension (as seen in the posterior elements). In addition, there are the purported benefits of restoration of segmental lordosis that may prevent adjacent segment degeneration.63
Interbody fusion combined with posterior instrumentation (pedicle screws) has been shown to have increased stability which allows for a more favorable low-strain environment for fusion.64 While the theoretical benefits of interbody fusion are well described, definitive studies showing that posterior interbody fusion decreases pseudoarthroiss rates have not yet been established. Technical factors are critical to the success of interbody fusion and include appropriate discectomy, vertebral body endplate preparation, and thorough removal of cartilaginous structures.
14.3.1 Minimally Invasive Transforaminal Lumbar Interbody Fusion
MIS TLIF combines many of the aforementioned techniques and theories in order to obtain a successful lumbar arthrodesis while preserving the midline ligamentous tension band and potentially still restoring sagittal alignment. Moreover, TLIF avoids the high rates of iatrogenic nerve injury seen in PLIF, allows for circumferential arthrodesis entirely from a posterior approach, and avoids the morbidity associated with anterior and lateral approaches to the interbody space. Ideal bone grafting in this situation is debated among surgeons and highly dependent on surgeon preferences; however, general principles of creating a vascularized fusion bed with a bone graft with ostoeconductive, osteoindiuctive, and osteogenic potential remain critical.
Choosing graft material in MIS TLIF is a complex question with limited data. Kasliwal et al examined using solely local autograft shavings combined with interbody cage in a small series of MIS TLIF patients and saw a 67.5% fusion rate, however, there was noted to be good clinical outcomes in 92% of patients.16
A recent meta-analysis of MIS TLIF patients including 1,533 patients over 40 series demonstrated high fusion rates (> 90%) overall regardless of graft choice.65 Subgroup analysis showed the addition of rhBMP to autologous local bone graft increased the fusion rate from 91.8 to 95.3%. The highest fusion rates were seen when BMP was combined with local autologous bone as well as bone graft extenders (99.1%). The highest fusion rate without the use of rhBMP was seen with autologous local bone and bone extender (93.1%). This suggests that high arthrodesis rates can be achieved without routine BMP use; however, the addition of BMP can further enhance overall fusion rates in MIS procedures. However, its use is not without complications as described previously.
14.3.2 Simultaneous Posterolateral Fusion in MIS TLIF
Posterolateral fusion using the transverse processes, pars interarticularis, and facet joint was first described by Watkins in 1953.66 It has widely become standard for posterior lumbar fusion procedures as it allows movement of the fusion mass anteriorly, closer to the axis of rotation of the lumbar spine, decreasing micromotion and tensile forces on graft material.2
MIS TLIF has been well described and can be performed with simultaneous posterolateral fusion by furthering dissection around facet joints and exposing and decorticating the transverse processes. While one critique of MIS TLIF includes difficult access to the intertransverse window for posterolateral fusion, we find that retractor systems can be easily used to achieve concomitant posterolateral fusion during MIS TLIF with a careful docking technique centered on the facet (Fig. 14.3).
Fig. 14.3 Minimally invasive surgery retractor system docking over the facet allowing for both transforaminal lumbar interbody fusion procedure and posterolateral intertransverse fusion. (Reproduced from Pipeline Expandable Access System (DePuy). In: Sandhu F, Voyadzis J, Fessler R, ed. Decision Making for Minimally Invasive Spine Surgery. 1st Edition. New York: Thieme; 2011.)
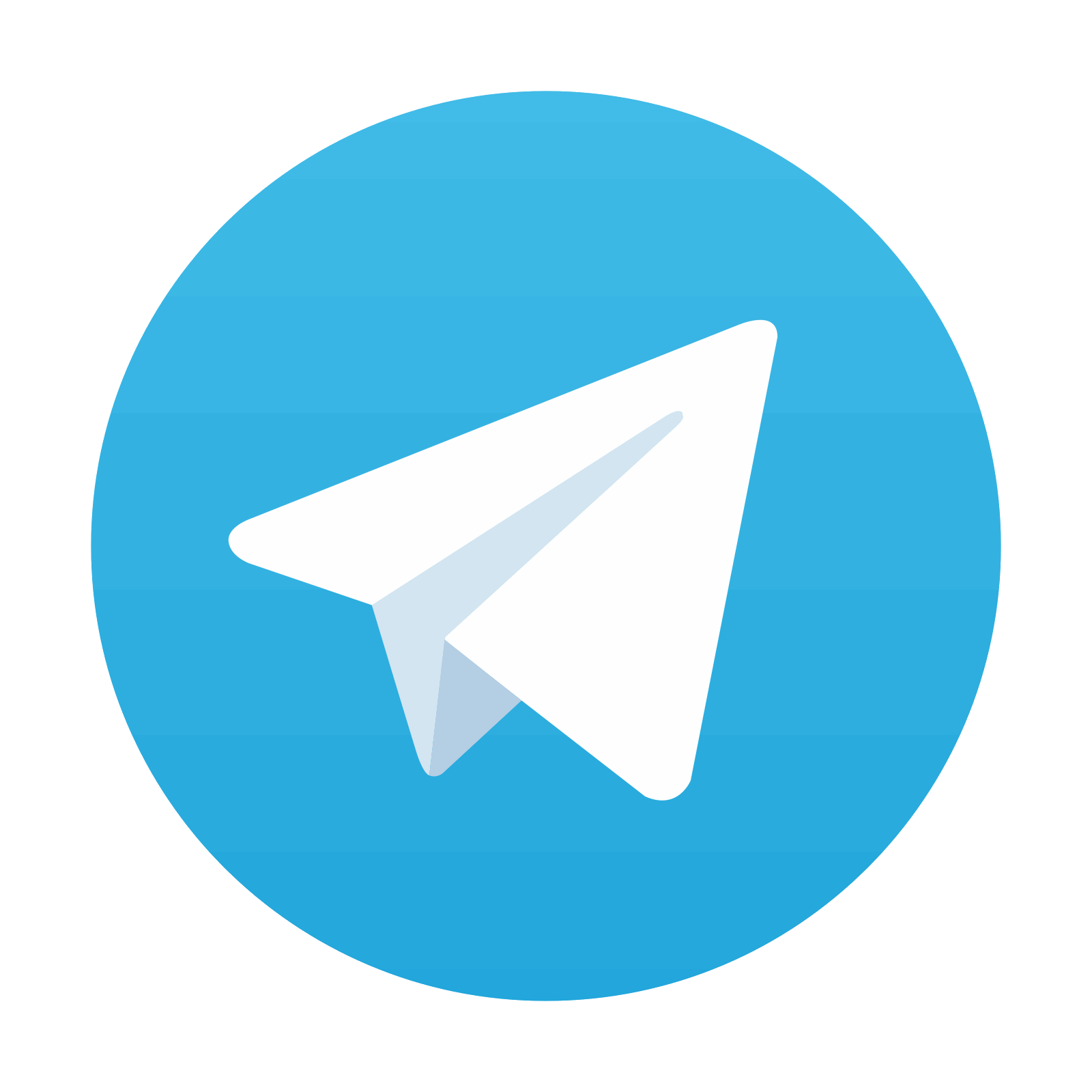
Stay updated, free articles. Join our Telegram channel

Full access? Get Clinical Tree
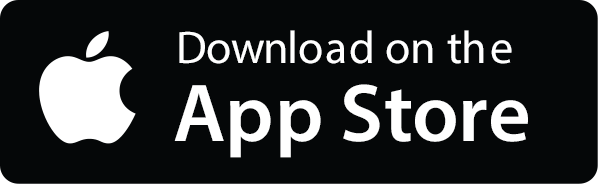
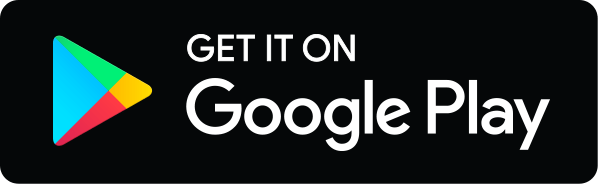