Chapter 6 Hydrocephalus in Children and Adults
• Hydrocephalus is one of the most challenging and common conditions that a neurosurgeon encounters.
• This condition can be due to intraventricular hemorrhage in the preterm infant, and its many other causes include trauma, intracranial hemorrhage, tumor, infection, aqueductal stenosis, and idiopathic origin in both the infant or adult.
• For 50 years, the mainstay of hydrocephalus treatment for patient survival and improved quality of life has been cerebrospinal fluid (CSF) diversion by shunting the ventricle or lumbar subarachnoid space to the peritoneum or atrium. However, the long-term complications of placement of a CSF shunt apparatus include infection, overshunting, and failure from obstruction.
• A more recent advance or alternative to shunting in a selected population of patients with hydrocephalus is endoscopic third ventriculostomy (ETV), in which CSF diversion is achieved via minimally invasive endoscopic fenestration of the floor of the third ventricle. It appears safe and is most effective for children over 1 month of age, those with aqueductal stenosis, and those who have not had a shunt previously.
Hydrocephalus refers to the buildup of cerebrospinal fluid (CSF) within the intracranial compartments usually associated with clinical sequelae from an increase in intracranial pressure (ICP). It can develop at any time, from the fetal period into adulthood, and it can have a myriad of causes including, but not limited to, congenital defects, perinatal insults, acquired conditions such as infection, tumor, traumatic and nontraumatic hemorrhage, and rarely CSF overproduction (Fig. 6.1).
Hydrocephalus is one of the most commonly encountered conditions in neurosurgery. One recent study by Sipek and colleagues analyzed data from the Czech national registry from 1961 to 2000 retrospectively and found the mean incidence of congenital hydrocephalus diagnosed both pre- and postnatally to be 6.35 per 10,000 liveborn infants.1 Another series by Fernell and Hagberg from Sweden found that the prevalence of infantile hydrocephalus was 6.99 per 1000 in the 1970s, increasing to 25.37 in the 1980s.2 The increase was thought to be due to the increased survival of very preterm infants. In the 1990s the prevalence of infantile hydrocephalus in the Swedish population decreased to 13.69. Despite changes in prevalence rates, outcome in surviving children with hydrocephalus remained similar. Other studies have looked at the prevalence of hydrocephalus in the adult population. A recent Norwegian study by Brean and Eide found the prevalence of idiopathic normal-pressure hydrocephalus (NPH) to be 21.9 per 100,000 and the incidence to be 5.5 per 100,000, reflecting minimum prevalence/incidence rate estimates.3 The incidence of hydrocephalus in developing countries is unknown but may be higher.
History
The recognition of hydrocephalus as a clinical entity dates back to antiquity. In the fifth century BC Hippocrates described the clinical presentation of hydrocephalus secondary to water accumulation within the head.4,5 Later, Galen described the choroid plexus and the relationship of CSF to the brain, though his understanding of CSF physiology was lacking.4 In the seventeenth century, Willis proposed the secretion of CSF by the choroid plexus and its absorption into the venous system, although the pathways he described were less than accurate.4 In 1701 Pacchioni described the arachnoid granulations, though he misidentified their function as the site of CSF production rather than absorption, and it was not until the late nineteenth century that the current accepted physiology of CSF production and absorption was clarified.4,6,7
The evolution of the treatment of hydrocephalus can be described by three stages.7 The first stage, up to the Renaissance, was characterized by poor medical understanding of CSF dynamics and pathology; therefore, nonsurgical and surgical treatment was largely useless. The second stage encompasses the period between the nineteenth and mid-twentieth centuries, when CSF physiology and pathology were elucidated but treatment options were in their infancy. In 1891 Quincke described lumbar puncture as a diagnostic modality and a means of treating hydrocephalus. Keen drained the cerebral ventricles through a temporal approach.4 Various lumbar and ventricular cannulation attempts were described, some more successful than others. Cushing reported treating hydrocephalus by a lumbar peritoneal connection, which was encouraging. Lespinasse in 1910 was the first to describe choroid plexus coagulation and the use of an endoscope (cystoscope) for the cannulation of the cerebral ventricles.4,7 In 1922 Dandy was the first to perform a third ventriculostomy through a subfrontal approach, and a year later Mixter performed the first endoscopic third ventriculostomy for noncommunicating hydrocephalus using a urethroscope.4 In 1939 Torkildsen described the use of a valveless rubber catheter to connect the lateral ventricles with the cisterna magna for noncommunicating hydrocephalus.4
The third stage of evolution in the treatment of hydrocephalus started with the development of silicone shunts with unidirectional valves in the 1950s. Nulsen and Spitz used a stainless steel unidirectional ball valve connected to a rubber catheter to divert CSF from the ventricles to the jugular vein in a hydrocephalic child.8 This was a landmark operation that marked the beginning of a new and powerful way of treating hydrocephalus. Variations and improvements on the valve used by Nulsen and Spitz ensued. Eventually, ventriculoperitoneal shunting became the standard surgical treatment for hydrocephalus, although both historically and in current clinical practice various sites for CSF diversion have been, and are still, used. However, shunt systems represent the introduction of a foreign material in the body, and complications related to infection and plugging of the shunt system are often encountered in clinical practice. Currently advances in technology and endoscopy have prompted resurgence in the use of third ventriculostomy for treating noncommunicating hydrocephalus as a means of obviating the inherent complications associated with shunts.
Cerebrospinal Fluid and Pathophysiology of Hydrocephalus
CSF is a clear colorless fluid produced mostly by the choroid plexus of the lateral, third, and fourth ventricles, and to a lesser degree (<20%) by the interstitial space and ependymal lining of the ventricles. In the spinal compartments, the nerve sleeve dura is responsible for CSF production9 (Table 6.1). Ninety-five percent of CSF produced by the ventricular choroid plexus occurs at the level of the lateral ventricles. CSF is found within the ventricles and cisterns of the brain and subarachnoid space, and surrounds both the brain and spinal cord. The infant has an approximate total volume of about 50 mL of CSF, and adults average 150 mL, half in the cranial compartment and half in the spinal compartment. Newborns produce CSF at a rate of 25 mL/day, which increases to about 500 mL/day in the adult (0.3-0.35 mL/minute).9 Intracranial pressure ranges from 9 to 12 cm H2O in the newborn to less than 18 to 20 cm H2O in the adult population. Generally, the rate of CSF formation is not dependent on intracranial pressure (ICP); however, CSF absorption is pressure dependent, and it occurs at the level of the arachnoid villi that are found in proximity to the dural venous sinuses.
TABLE 6.1 Sites of Cerebrospinal Fluid Production
Compartment | Site |
---|---|
Intracranial | Choroid plexus of the lateral, third, and fourth ventricles Ependymal lining Interstitial space |
Spinal | Dura of the nerve root sleeves |
The circulation and physiology of CSF and its circulation can be quite complex. More than 2 centuries ago Alexander Monro applied principles of physics to the relationship of intracranial contents.10 This was supported by experiments conducted by Kellie.10 Today their work is known as the Monro-Kellie doctrine (Table 6.2), which states that within the rigid container of the skull, the sum of intracranial contents, including CSF, blood, and brain, is constant.10,11 Therefore, a change in one component (e.g., increase in CSF) requires a compensatory change in one or both of the other components. However, in very young children, usually less than 2 to 3 years of age, the fontanelles are still open and the system is no longer closed; in this scenario the hypothesis does not apply.
TABLE 6.2 The Monro-Kellie Doctrine
The sum of intracranial contents to include blood, CSF, and brain should remain constant in a fixed container, such as the skull. |
CSF flow through the ventricular system occurs in a progressive manner, from the lateral ventricles through the foramina of Monro to the third ventricle, through the sylvian aqueduct to the fourth ventricle, then through the foramina of Luschka and Magendie to the subarachnoid spaces (Fig. 6.2). From the subarachnoid spaces, CSF is absorbed into the venous circulation through the arachnoid granulations. A dysregulation in production, absorption, or circulation of CSF can lead to symptomatic hydrocephalus.
Rarely hydrocephalus can be associated with CSF overproduction, as in the case of choroid plexus tumors. However, in most instances hydrocephalus is due to an obstruction along the pathway of CSF flow. Tumors of the lateral ventricles can cause hydrocephalus by mass effect or CSF overproduction in the case of tumors derived from the choroid plexus. Common tumors of the lateral ventricles include meningiomas, gliomas, and choroid plexus tumors. Choroid plexus tumors are rare, seen more commonly in children younger than 2 years of age, and account for less than 1% of all intracranial tumors12 (Fig. 6.3). Even with complete surgical tumor resection, hydrocephalus may still persist and require treatment. This is thought to be due to more distal obstruction (i.e., aqueduct, arachnoid villi) either from preoperative microhemorrhages or scarring after surgery. Rarely choroid plexus villous hypertrophy can lead to CSF overproduction, which can be treated by choroid plexus coagulation.13 Obstruction at the foramina of Monro can also be caused by congenital atresia, membranes, or gliosis after hemorrhage, and can lead to unilateral ventriculomegaly.14
In the third ventricle, cysts and tumors can cause hydrocephalus by CSF obstruction. Colloid cysts, found at the anterior superior part of the third ventricle, generally represent less than 2% of intracranial tumors and are more commonly symptomatic in the adult population, with clinical presentations ranging from acute to chronic hydrocephalus due to obstruction of the foramina of Monro15 (Fig. 6.4). Treatment is aimed at stereotactic aspiration, endoscopic resection, or open microsurgery.16 Other cystic lesions include arachnoid and ependymal cysts, and rarely dermoid cysts. Third ventricular neoplasms include craniopharyngiomas and gliomas, including hypothalamic astrocytomas and subependymal giant cell astrocytomas (in association with tuberous sclerosis). Hydrocephalus may persist after glioma resection, necessitating shunt placement.
Obstruction may be seen at the level of the sylvian aqueduct, especially in neonates in whom the small diamter (0.2-0.5 mm) of the aqueduct puts it at risk for obstruction from congenital and acquired causes (Fig. 6.5). This leads to enlargement of the third and lateral ventricles. Congenital aqueductal malformations include stenosis, forking, septum formation, and subependymal gliosis due to in utero infections. True luminal stenosis is not as common. In addition, lesions such as arteriovenous malformations and periaqueductal tumors such as tectal gliomas and pineal region tumors can cause obstruction at or near the sylvian aqueduct, leading to triventricular obstructive hydrocephalus (Fig. 6.6). Often, resection of the lesion is sufficient to treat the hydrocephalus. Newer methods of endoscopic aqueductoplasty and stenting are showing promise.16
The fourth ventricle and basal foramina can also be sites of obstruction leading to hydrocephalus. Dandy-Walker malformations present in infants with a large posterior fossa cyst in the setting of cerebellar vermian hypoplasia and cerebellar atrophy, commonly associated with hydrocephalus as well as other congenital abnormalities (Fig. 6.7). Tumors of the posterior fossa and fourth ventricular region can present with acute or chronic hydrocephalus in addition to other associated posterior fossa symptoms. In the adult population common tumors include metastases, gliomas, meningiomas, neuromas, and hemangioblastomas. In the pediatric population, infratentorial tumors are common causes of hydrocephalus and common offenders include medulloblastomas, ependymomas, cerebellar astrocytomas, and brainstem gliomas. Across the age spectrum, infections and subarachnoid hemorrhage can lead to arachnoid scarring and hydrocephalus (Fig. 6.8). Congenital conditions such as Chiari malformations may cause hydrocephalus by obstruction of CSF flow around the base of the skull. Obstruction of CSF flow can also occur at the level of the arachnoid granulations with impairment of CSF absorption. This may be idiopathic or may be seen after infection, subarachnoid hemorrhage, trauma, or tumors and can lead to enlargement of CSF spaces over the convexities.
In models of adult hydrocephalus, conditions leading to scarring distal to the ventricular system (e.g., subarachnoid hemorrhage or infection) cause a resistance to normal CSF outflow. However, the pathophysiology of CSF circulation is quite complex. CSF pulsation variability and cerebral blood flow dynamics have been implicated in both idiopathic and secondary hydrocephalus, though their exact role is still a topic of investigation.17,18 In addition, the properties of the surrounding parenchyma, particularly its compressibility, has been proposed as a means of explaining the observation of hydrocephalic symptoms in the settings of lower ventricular pressures.19 For example, if the brain parenchyma is able to attenuate increases in intraventricular pressure, the overall intracranial pressure does not need to rise above normal levels in the setting of abnormal transventricular pressure gradients, leading to the entity commonly called normal-pressure hydrocephalus (NPH).19 It is not clear if parenchymal damage plays a primary or secondary role in the development of NPH.
Classification of Hydrocephalus
There are different classifications and means of describing hydrocephalus. Often different schemes are employed for hydrocephalus in infants and children versus adults, delineating the difference in pathophysiology and clinical presentation across the age spectrum. In general, hydrocephalus can be defined as nonobstructive, associated with ventricular system enlargement (e.g., hydrocephalus ex vacuo), or obstructive, associated with defective CSF circulation or absorption (Table 6.3). A more clinically useful scheme divides obstructive hydrocephalus into communicating and noncommunicating types. Generally, communicating hydrocephalus refers to an obstruction outside the ventricular system, often at the level of the subarachnoid space or the arachnoid villi. Noncommunicating hydrocephalus refers to an impedance of CSF flow within the ventricular system, such as blockage at the aqueduct of Sylvius or the basal foramina of Luschka and Magendie. Less clinically useful schemes describe hydrocephalus as physiological (secondary to CSF overproduction) or nonphysiological, external or internal (obstruction outside or within the ventricular system, respectively).
TABLE 6.3 Hydrocephalus Classification
Type | Features |
---|---|
Nonobstructive | Ventricular enlargement (e.g., hydrocephalus ex vacuo) |
Obstructive | |
Communicating | Obstruction outside the ventricular system (e.g., subarachnoid space or arachnoid villi) |
Noncommunicating | Obstruction within the ventricular system (e.g., aqueduct or basal foramina) |
A system proposed by Gowers in 1888, still useful today, divides hydrocephalus as either acute or chronic, primary or secondary.20 Generally, acute hydrocephalus implies a rapid decompensation usually associated with an underlying condition and presents with elevated intracranial pressure. Chronic hydrocephalus may be either idiopathic or secondary to a known pathological condition, and generally is associated with lower or normal intracranial pressures. Secondary hydrocephalus can be due to a number of clinical conditions, including but not limited to tumors, hemorrhage, trauma, and infection.
Etiology and Clinical Presentation
There are different etiologies for hydrocephalus depending on the age group of patients (Table 6.4). In infants, numerous causes are clinically identified. Particularly in premature infants, posthemorrhagic hydrocephalus (PHH) is often seen as a sequela of intraventicular or germinal matrix hemorrhage (Table 6.5). The intraventricular blood leads to fibrosing arachnoiditis, meningeal fibrosis, and subependymal gliosis, altering the physiology of CSF flow.21 In full-term infants hydrocephalus can be ascribed to a different etiological set, including but not limited to aqueductal stenosis, Dandy-Walker malformations, tumors, arachnoid cysts, vein of Galen malformations, Chiari malformations, and so on.22 Intrauterine infections can also lead to congenital hydrocephalus. In older children, common causes include various tumors that obstruct CSF flow along its ventricular path, as well as trauma and infection (e.g., meningitis).
TABLE 6.4 Pathologic Conditions Associated With Hydrocephalus
Congenital | Acquired |
---|---|
Chiari I malformation | Postinfectious |
Chiari II malformation (associated with myelomeningocele) | Posthemorrhagic (including subarachnoid and intraventricular hemorrhage) |
Primary aqueductal stenosis (or gliosis secondary to intrauterine infection or germinal matrix hemorrhage) | Post-traumatic |
Dandy-Walker malformation | Secondary to mass lesions (e.g., tumors, vascular malformations, cysts) |
Hydranencephaly | Postoperative (e.g., after tumor resection including posterior fossa tumors) |
TABLE 6.5 Grading of Germinal Matrix Hemorrhage
Grade | Description |
---|---|
I | Subependymal hemorrhage |
II | Intraventricular hemorrhage with no ventricular dilatation |
III | Intraventricular hemorrhage with ventricular dilatation |
IV | Intraventricular hemorrhage with intracerebral hemorrhage |
Several studies have looked at trends in causes of hydrocephalus in specific groups. A retrospective review by Green and co-workers of 253 infants and children with hydrocephalus treated at a British tertiary care center over a 10-year period revealed interesting trends in the causes of this condition.23 In the first half of the decade, the predominant causes were posthemorrhagic hydrocephalus and hydrocephalus due to brain tumors, which decreased from over half of the children to one third by the end of the decade; the rate of neonatal intraventricular hemorrhage decreased by 45%.23
In older children, hydrocephalus may occur secondary to neoplasms or trauma (Fig. 6.9). Children can present with headaches (dull, typically upon awakening), vision changes (blurry or double vision), lethargy, vomiting, decreased food intake, behavioral disturbances, poor school performance, and endocrinopathies (e.g., short stature, precocious puberty). On examination, papilledema and lateral rectus palsies can be seen, as well as hyperreflexia and clonus. If progressive lethargy is noted, diagnosis and treatment become urgent. In severe cases of increased intracranial pressure and severe hydrocephalus, Cushing’s triad can be seen. Cushing’s triad consists of bradycardia, hypertension, and irregular breathing, and requires emergent evaluation and treatment.
NPH is a common diagnosis encountered by neurosurgeons in the adult population (Fig. 6.10). Patients with NPH present with the clinical triad of gait disturbances, dementia, and urinary incontinence, usually in the sixth to eight decades of life24 (Table 6.6). Generally, gait disturbances, described as apraxic or magnetic, are the first symptoms noted. Patients develop a slow, wide-based shuffling walking pattern. Urinary incontinence is a common presentation of NPH. Early in the course patients develop urinary frequency and urgency, which progress to incontinence owing to bladder hyperactivity.24 The cognitive decline must be differentiated from other causes of dementia (vascular, Alzheimer’s disease, dementia with Lewy bodies, etc.). Generally, the dementia associated with NPH is subcortical in nature. Often patients show apathy, psychomotor retardation, difficulty with executive function, and inattention; apraxia, aphasia, and agnosia are not seen.24 In the elderly, the symptoms associated with NPH must be distinguished from other common causes seen in this patient population, including vascular and neurodegenerative disorders.
TABLE 6.6 Symptomatic Triad of Normal-Pressure Hydrocephalus (NPH)
A nonhydrocephalic clinical entity presenting with increased intracranial pressure, seen in both the pediatric and adult populations, is the syndrome of idiopathic intracranial hypertension, also commonly known as pseudotumor cerebri. The ventricles are not enlarged and are usually small. Patients are generally overweight women who develop increased intracranial pressure without an identifiable source, and it remains a diagnosis of exclusion. The pathophysiology of this syndrome is still unclear, though venous stenosis and effects of the hormone leptin have been implicated.25 Symptoms include headaches and papilledema, and up to 25% of patients develop visual deterioration from optic nerve atrophy.
Diagnosis
Neuroimaging studies are the mainstay for visualizing and understanding ventricular anatomy and diagnosing hydrocephalus in symptomatic cases. In utero diagnosis of hydrocephalus is often accomplished via ultrasound studies, though fetal MRIs are gaining popularity as diagnostic adjuncts.26 In infants with intraventricular hemmorrhage (IVH) and suspected hydrocephalus, sonography is often used to evaluate the ventricles because the anterior fontanelle provides a good window for ventricular visualization.27 Diagnosis of mono-, bi-, or triventricular hydrocephalus can be made by using ultrasound evaluation of the lateral and third ventricles; however, posterior fossa imaging is limited (Fig. 6.11).
The current standard imaging method in the diagnosis of hydrocephalus is CT scanning. CT scans provide an effective way of visualizing ventricular morphology, blood products complicating the picture of hydrocephalus, transependymal edema, and signs of increased intracranial pressure such as sulcal/gyral effacement and obliteration of subarachnoid spaces. The Evans ratio describes the ratio of the lateral ventricular frontal horn width to the maximal biparietal diameter, and is abnormal and indicative of ventriculomegaly if it is greater than 0.3.28 Occasionally, in select cases, cisternography or ventriculography can be performed. This often entails the administration of a radiopaque contrast agent into the ventricular system to evaluate the compartmentalization of cysts and determine whether communication of CSF is present (i.e., posterior fossa cysts in Dandy-Walker malformations, or a trapped fourth ventricle).29 MRI studies are particularly useful in delineating disease responsible for CSF pathway obstruction, such as tumors compressing the ventricular system, and provide a better evaluation of the posterior fossa and foramen magnum. MRIs allow for the evaluation of ventricular anatomy in coronal, sagittal, and axial planes (Fig. 6.12). In addition, MRI CSF flow studies (e.g., cine flow studies) timed to the cardiac cycle can provide useful information in selected cases.
< div class='tao-gold-member'>
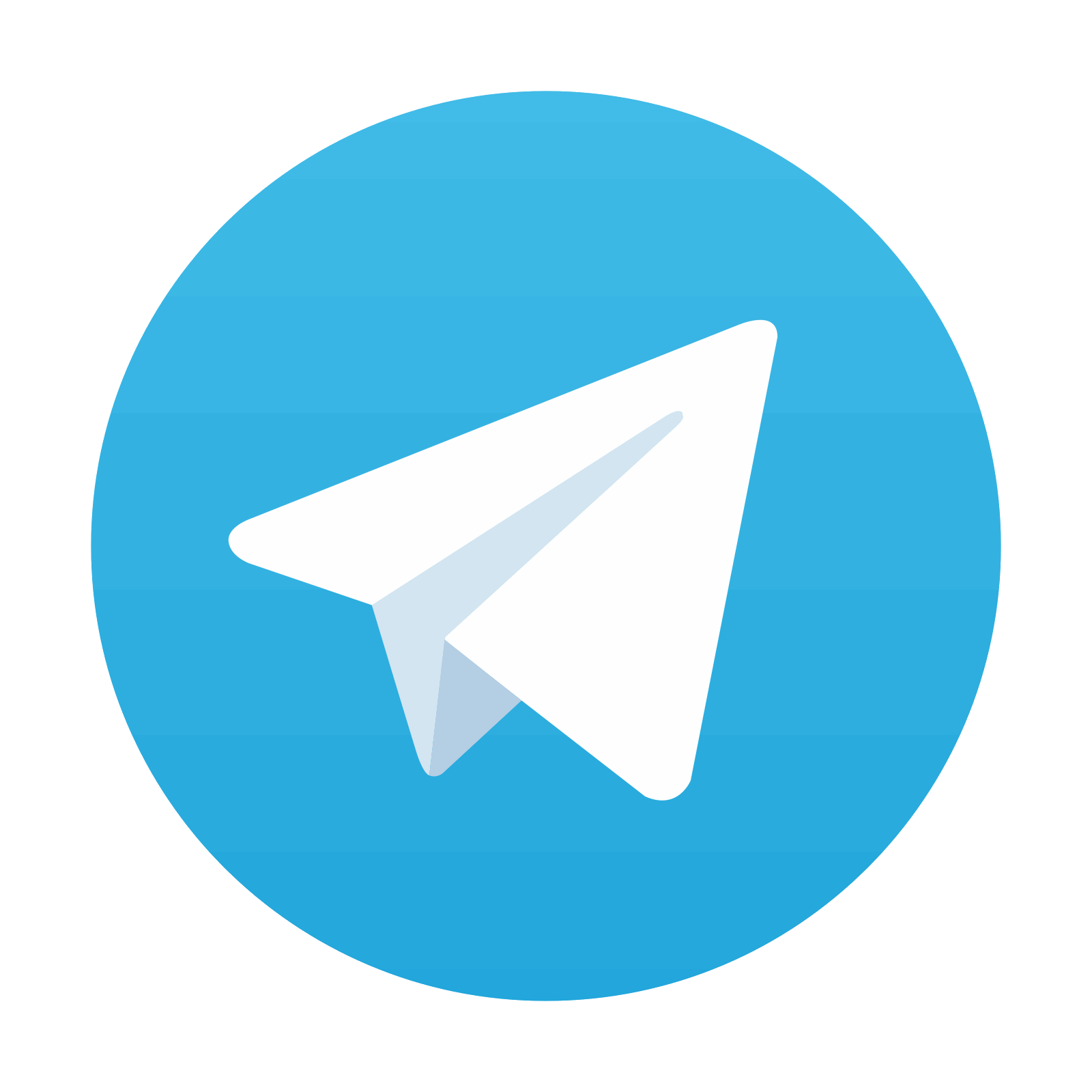
Stay updated, free articles. Join our Telegram channel

Full access? Get Clinical Tree
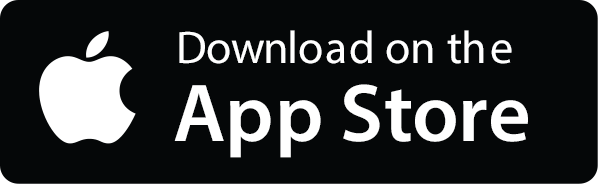
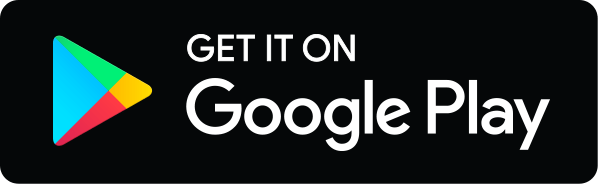