Abstract
Neonatal encephalopathy occurs in 2 to 5/1000 live births and is principally related to hypoxic-ischemic injury to the newborn brain in the immediate peripartum period. In the last decade, there have been significant advances in neuroprotection that have been successful in reducing the risk of death and disability in infants who have suffered from a potential hypoxic-ischemic cerebral injury. The advent of therapeutic hypothermia has led to a major focus on the early clinical recognition of infants that may benefit from such therapies. In addition, optimal recognition and therapeutic targeting of neonatal seizures, with other neuroprotective approaches, have been emphasized. This chapter will summarize the clinical presentation, neuropathological correlates, neurodiagnostic evaluation, prognosis, and management of this condition.
Keywords
hypoxic-ischemic encephalopathy, neonatal encephalopathy, neonatal seizures, perinatal asphyxia, deep nuclear gray matter injury, watershed injury
The clinical aspects of neonatal hypoxic-ischemic encephalopathy (HIE) are appropriately discussed following the neuropathology (see Chapter 18 ) and pathophysiology (see Chapter 19 ), because understanding of the clinical phenomena is facilitated greatly by an awareness of the underlying pathological substrates. Moreover, choice of appropriate diagnostic modalities, formulation of rational prognostic statements, and development of appropriate plans of management are based, in many ways, on awareness of the probable neuropathologies. In this chapter, we discuss the clinical settings for neonatal HIE, the clinical syndrome, diagnostic studies, clinical correlations, prognosis, and management.
Clinical Settings
The importance of the early recognition of the clinical risk factors for hypoxic-ischemic cerebral injury in the term born infant has escalated significantly in the last decade with the implementation of successful neuroprotection with therapeutic hypothermia (see later sections). To initiate such therapy requires a recognition of the infant who may have suffered hypoxic-ischemic cerebral injury—predominantly in the peripartum period. The peripartum period is defined as the period shortly before, during, and immediately after birth. The clinical settings for neonatal HIE are dominated by the ultimate occurrence of ischemia (i.e., diminished blood supply to brain), usually, but not necessarily, preceded or accompanied by hypoxemia (i.e., a diminished amount of oxygen in the blood supply). Hypoxemia leads to brain injury principally by causing myocardial disturbance and loss of cerebrovascular autoregulation, with ischemia the major consequence. The temporal characteristics and the severity of the hypoxemia and ischemia, as well as the gestational age of the infant, are the principal determinants of the type of resulting neuropathology (see Chapters 18 and 19 ).
The major causes of serious hypoxemia in the peripartum period are: (1) hypoxia-ischemia with intrauterine disturbance of gas exchange across the placenta (i.e., asphyxia) or with failure to establish independent respiration at the time of birth or both; (2) postnatal respiratory insufficiency secondary to severe respiratory disease; and (3) severe right-to-left shunt secondary to persistent fetal circulation or cardiac disease. The major causes of serious ischemia are: (1) intrauterine asphyxia (i.e., hypoxemia, hypercarbia, and acidosis) with cardiac insufficiency and loss of cerebrovascular autoregulation both in utero and at the time of birth; (2) postnatal cardiac insufficiency secondary to severe hypoxemia or congenital heart disease; and (3) postnatal (postcardiac) circulatory insufficiency secondary to patent ductus arteriosus (with “ductal steal”) or vascular collapse (e.g., with sepsis).
In this chapter, we will focus on the encephalopathic syndrome in the term infant and the presence of evidence for peripartum hypoxic-ischemic injury. We prefer the term “peripartum” hypoxic-ischemic injury as it acknowledges the potential presence of (1) fetal or maternal prepartum conditions that may accentuate propensity to intrapartum hypoxic-ischemic injury; (2) intrapartum hypoxic–ischemic injury per se; and (3) the often associated protracted postpartum resuscitative efforts for such infants, with no or low heart rate for several minutes. Although the postnatal cardiac compromise is not the primary etiology of the poor birth transition, it may contribute to the extent of the ultimate hypoxic-ischemic cerebral injury in the infant. However, there is also evidence that even with prolonged resuscitation, in the era of therapeutic hypothermia there can be better outcomes than might be expected (see the section on prognosis ).
It is important to recognize that not all neonatal encephalopathies are related to hypoxic-ischemic disease. Antepartum and postpartum disorders (e.g., infectious, metabolic, dysgenetic) may lead to neonatal encephalopathies, as discussed throughout this book. In one large population-based observational study, the prevalence of moderate to severe encephalopathy was 1.64 per 1000 live term births, and the prevalence of “birth asphyxia” was 0.86 per 1000 live term births. Fully 56% of all cases of newborn encephalopathy were related to hypoxic-ischemic injury that occurred during the intrapartum period. These findings are consistent with a more recent large cohort study of 4165 singleton term infants with any one of the following: seizures, stupor, coma, Apgar score at 5 minutes less than 3 and/or receiving hypothermia therapy. In this study, 15% of the infants experienced a clinically recognized sentinel event, such as antenatal hemorrhage (presumably, often placental abruption), uterine rupture, or cord prolapse, all of which are capable of compromising oxygen supply. Almost one half of the infants displayed umbilical cord blood gas acidemia and/or fetal bradycardia. Of note, signs of inflammation were also not uncommon with 27% of mothers displaying elevated maternal temperature in labor and 11% clinical chorioamnionitis. However, the contributing role of chorioamnionitis is not consistently supported. Although intrapartum sentinel events provide clear evidence of a hypoxic-ischemic insult, in three studies of neonatal encephalopathy, sentinel intrapartum events were only identified in 8% to 25% of infants. In a referral sample of 500 term infants with neonatal encephalopathy evaluated for therapeutic hypothermia, 48 (9%) had a sentinel birth event. Thus, it can be challenging to confirm an hypoxic-ischemic etiology for the infant with neonatal encephalopathy and/or the need for resuscitation as only 10% to 20% of such infants may have a clinical history of a major risk factor, whereas approximately 50% or more may have a constellation of risk factors including maternal history, cord acidemia, and the need for resuscitation that supports this as the most likely etiology for their neurological syndrome.
In addition, although obvious, hypoxic-ischemic injury may affect the infant’s brain during the antepartum and postnatal periods, albeit much less commonly than the intrapartum period . On the basis of earlier work, approximately 20% of hypoxic-ischemic injury recognized in the newborn period was said to be related primarily to antepartum insults. These data should be interpreted with the awareness that assessment of timing of insults to the fetus in these reports generally was based on imprecise methods, and the variability of findings is considerable. Moreover, more recent studies with consistent use of magnetic resonance imaging (MRI) suggest that the large majority of hypoxic-ischemic injury occurs during the intrapartum period.
The best data indicate that most infants with neonatal HIE and intrapartum evidence of hypoxic-ischemic insult exhibit, on MRI, evidence only of injury from the immediate peripartum period with no clear evidence of long-standing antenatal hypoxic-ischemic disease ( Table 20.1 ).
Of 245 infants who had an MRI scan after neonatal neurological signs (“neonatal encephalopathy”), and evidence of intrapartum perinatal asphyxia:
|
In one study of 245 term infants with neonatal encephalopathy and evidence of intrauterine asphyxia, fully 80% had evidence of acute lesions (within the period immediately before or during labor and delivery) consistent with hypoxic-ischemic disease, 16% had normal MRI scans, and only 4% had concomitant evidence of chronic antenatal injury (see Table 20.1 ). In another MRI study of 173 term newborns with encephalopathy and signs of intrauterine asphyxia, only acute injury was observed. Related clinical and epidemiological data also support a marked preponderance of intrapartum events in the origin of neonatal HIE, especially in the term infant.
The principal intrapartum events leading to hypoxic-ischemic fetal insults include acute placental or umbilical cord disturbances, such as abruptio placentae or cord prolapse, prolonged labor with transverse arrest, difficult forceps extractions, or rotational maneuvers (see Chapter 17 , Chapter 18 , Chapter 19 ). Postpartum events alone (e.g., severe persistent fetal circulation, severe recurrent apneic spells, cardiac failure secondary to large patent ductus arteriosus or other congenital heart disease, severe pulmonary disease) may lead to HIE and may account for approximately 5% to 10% of cases. Most of these and related postnatal factors are much more important in the pathogenesis of hypoxic-ischemic brain injury in the premature infant than in the term infant (see Chapter 14 , Chapter 15 , Chapter 16 ). Although hypoxic-ischemic injury certainly can occur in the antepartum period (e.g., secondary to maternal trauma, maternal hypotension, uterine hemorrhage), this injury cumulatively accounts for between 5% and 20% of neonatal HIE (as noted earlier). However, antepartum factors appear to be of some importance in the risk for neonatal encephalopathy related to peripartum events. Such factors may indeed predispose to intrapartum hypoxia-ischemia during the stresses of labor and delivery, especially through threats to placental flow. Such factors include maternal diabetes, preeclampsia, placental vasculopathy, intrauterine growth restriction, and twin gestation that may compromise fetal cerebral perfusion ( Table 20.2 ; see Chapter 13 ). In one series, such factors were present in approximately one third of cases of intrapartum asphyxia. Indeed, “perinatal asphyxia” was identified in 27% of infants of diabetic mothers, and its occurrence correlated closely with diabetic vasculopathy (nephropathy) and presumed placental vascular insufficiency. In a more recent cohort of infants that received therapeutic hypothermia ( n = 98), the frequency of pregestational diabetes and preeclampsia were significantly higher (threefold to fivefold) in women with infants requiring cooling.
FREQUENCY IN GENERAL POPULATION (%) | FREQUENCY IN HIE POPULATION (%) | ||
---|---|---|---|
Antepartum/Maternal | Hypothyroidism | 0.5 | 3 |
Obesity | 10–25 | 15–50 a | |
Diabetes (particularly pregestational) | 0.5–2 | 5–20 a | |
Fetal growth restriction <5% | 5 | 10–15 | |
Hypertension | 3–5 | 5–15 | |
Clinical chorioamnionitis | 1–4 | 5–10 |
Regarding intrauterine growth restriction, in the largest North American series of neonatal encephalopathy, collected by the Vermont Oxford Registry, 16% of infants were defined as less than the 10th% for weight. In a major controlled study of neonatal encephalopathy, 16% of infants with neonatal encephalopathy were growth-restricted compared to only 1.2% of term infants without encephalopathy. Growth restriction was the strongest predictor of neonatal encephalopathy examined, associated with a 30-fold increase in risk. In a regional study of moderate or severe neonatal encephalopathy in term infants, 17% were small for their gestational age. The additional stress of labor would be expected to compromise placental blood flow. Similarly, impaired placental function and an increased risk of perinatal asphyxia in the infant with intrauterine growth restriction are recognized and appear to account for some of the increased risk of subsequent neurological disability in such infants. In the most recent series of infants receiving hypothermia, infant birthweight below the 5th percentile for gestational age was significantly associated with the need for therapeutic hypothermia. Other factors (e.g., dysmorphic syndromes, severe undernutrition, infection) may also lead to increased risk of neurological disability in intrauterine growth restriction. Moreover, studies in fetal and neonatal animals suggest that the mechanisms for the increased vulnerability of the growth-restricted fetus relate not only to placental insufficiency but also to diminished glucose reserves in the heart, liver, and brain, and to impaired capability to increase substrate supply to the brain with the hypoxic stress of vaginal delivery.
Other less characterized maternal factors have been recognized as important risk factors for neonatal encephalopathy, although pathogenetic mechanisms remain unclear. One such factor is maternal hypothyroidism. In four prospective studies, an elevated risk of up to 10-fold was found for maternal hypothyroidism in infants with neonatal encephalopathy (see Table 20.2 ). Further, maternal drug use can impair the transition of infants after delivery, and infants can display abnormal neurological signs that can mimic neonatal encephalopathy. The details of these agents are outlined in Chapter 38 .
Although the particular importance of intrauterine hypoxic-ischemic injury, especially intrapartum asphyxia with or without antepartum predisposing factors, in the genesis of the clinical syndrome of neonatal HIE is apparent, most infants who experience intrapartum hypoxic-ischemic insults do not exhibit overt neonatal neurological features or subsequent neurological evidence of brain injury. a
a References .
The severity and duration of the hypoxic-ischemic insult is obviously critical. The elegant studies of Low and others demonstrate a striking relationship among the severity and duration of intrapartum hypoxia, assessed by the use of fetal acid-base studies (see Chapter 17 ), the subsequent occurrence of a neonatal neurological syndrome, and later neurological deficits. Current data suggest that approximately 10% of all term deliveries require some resuscitation with 1% requiring extensive resuscitation, Of the latter. only 1 to 3 per 1000 will develop signs of evolving encephalopathy consistent with HIE.Neurological Syndrome
The neurological syndrome that accompanies serious peripartum hypoxic-ischemic cerebral injury is the prototype for neonatal HIE. In considering the nature and timing of hypoxia-ischemia as the etiology of neonatal HIE, we consider three features to be important: (1) evidence of fetal distress and/or fetal risk for hypoxia-ischemia (e.g., fetal heart rate (FHR) abnormalities, sentinel event, fetal acidemia); (2) the need for resuscitation and/or low Apgar scores; and (3) an overt neonatal neurological syndrome in the first hours or day of life.
Although not discussed here in depth, important systemic abnormalities , presumably related to ischemia, often accompany the neonatal neurological syndrome. The relative frequencies of manifestations of organ injury in term infants with evidence of asphyxia have been investigated in several studies. The findings varied as a function of the severity of asphyxia and the definitions of organ dysfunction. In combined data from two reports ( Table 20.3 ), approximately 20% of infants with apparent fetal asphyxia had no evidence of organ injury. Evidence of involvement of the central nervous system occurred in 62% of infants. Indeed, in 16% of infants, involvement of only the nervous system was apparent. The order of frequency of systemic organ involvement overall has been hepatic > pulmonary > renal > cardiac. In an autopsy series, cardiac involvement was the most common among affection of systemic organs. With careful electrocardiographic and enzymatic studies of living infants after perinatal asphyxia, evidence of myocardial ischemia has been commonly observed. Representative data from a well-studied series of 144 infants with moderate-severe encephalopathy, found that all infants displayed some form of organ dysfunction, with pulmonary and hepatic approximately 85%, renal 70%, and cardiac 60%. These frequencies may relate, in part, to the nature of the diagnostic categories for these abnormalities, but confirm that multiorgan dysfunction is very common in the setting of moderate-severe peripartum HIE and should be sought by appropriate diagnostic studies.
ORGAN | PERCENTAGE OF TOTAL |
---|---|
None | 0–36 |
CNS only | 0–36 |
CNS and one or more other organs Renal & cardiac approx. 65%: Pulmonary & liver approx. 85% | 46–100 |
Other organ(s), no CNS | 10–20 |
a Cumulative total of 107 term infants; definition of asphyxia in both series included umbilical cord arterial pH < 7.2.
Defining the neurological syndrome of HIE by clinical evaluation is important. Central to this definition is awareness of the characteristics of the normal neurological examination (see Chapter 9 ). The abnormal features of the examination in infants with HIE were discussed in previous editions of this book and in Chapter 9 . To improve interobserver reliability, standardized scores have been developed, and have proven useful in large-scale clinical research studies (see later) ( Table 20.4 )
SCORING SYSTEM | PURPOSE/UTILITY | NUMBER OF ELEMENTS | ELEMENTS | EEG NECESSARY |
---|---|---|---|---|
Sarnat | Prognosis applied in first 7 days | 14 | Alertness, tone, posture, reflexes, myocolonus, suck, Moro, oculovestibular, tonic neck, pupils, heart rate, secretions, GI motility, seizures, EEG | Yes |
Modified Sarnat | Prognosis applied in first 7 days | 5 | Alertness, tone, suck, Moro, seizures | No |
Thompson | Prognosis applied in first 7 days | 6 | Alertness, tone, respiratory status, reflexes, seizure, feeding method | No |
NICHD | Selection in first 6 h of life of moderate-severe NE for hypothermia | 9 | Alertness, spontaneous activity, posture, tone, suck, Moro, pupils, heart rate, respirations | No |
SIBEN | Defining mild, moderate, and severe NE in first 6 h of life | 10 | Alertness, spontaneous activity, posture, tone, suck, Moro, pupils, heart rate, respirations, seizures | No |
The initial neurological examination classification systems developed evaluated infants over the first week of life to define the severity of their encephalopathy for prognostication. The first of these scoring systems was that developed by Sarnat, which was based on serial examinations of 21 term-born infants over the first few weeks of life. Three clinical stages of “postanoxic encephalopathy” were described. Stage 1 lasted less than 24 hours and was characterized by hyperalertness, uninhibited Moro and stretch reflexes, sympathetic effects, and a normal electroencephalogram. Stage 2 was marked by obtundation, hypotonia, strong distal flexion, and multifocal seizures. The electroencephalography (EEG) showed a periodic pattern sometimes preceded by continuous delta activity. Infants in stage 3 were stuporous, flaccid, and their brain stem and autonomic functions were suppressed. The EEG was isopotential or had infrequent periodic discharges. Infants who did not enter stage 3 and who had signs of stage 2 for less than 5 days appeared normal in later infancy. Persistence of stage 2 for more than 7 days, or failure of the EEG to revert to normal, was associated with later neurologic impairment or death. This classification system was then further simplified, to be known as the Modified Sarnat score (see Table 20.4 ).
The next scoring system developed for prognostication in neonatal encephalopathy was the Thompson Encephalopathy Score, developed in 1997 (see Table 20.4 ). This scoring system was simpler to apply and did not require EEG to increase its widespread applicability. The initial evaluation showed a good correlation between the maximal score in the first 7 days of life and neurodevelopmental outcome at 18 months in 44 infants with neonatal HIE.
It is important to note that both the Sarnat and the Thompson scoring systems aimed to define neonatal neurological signs during the first week of life to improve the prediction of subsequent neurological deficits. However, as the era of neuroprotection emerged, it became apparent that a standardized neonatal neurological examination tool to be applied in the first few hours of life would be necessary to define eligibility for randomized controlled trials, such as therapeutic hypothermia. For some of the latter trials, the modified Sarnat and Thompson scales were used. For the largest North American study, a new scoring system was developed: the NICHD (National Institute of Child Health and Human Development) Neonatal Encephalopathy Scoring System (see Table 20.4 ). The aim of this scoring system was to identify infants with moderate-severe encephalopathy who were eligible for entry into the trial within the first 6 hours of life. There was recognition that the examination could evolve over the first day of life, as described by Sarnat and Thompson (see temporal evolution of the neurological syndrome later).
To further refine the NICHD scoring system by the addition of a mild encephalopathy grouping, the HIE Score of the Iberoamerican Society of Neonatology (SIBEN) was developed in 2016 and involved the assessment of 10 clinical aspects that could be undertaken from immediately after delivery room resuscitation (see Tables 20.4 and 20.5 ). The recognition of mild encephalopathy is of great relevance as it is recognized that at least 40% of hypoxic-ischemic cerebral injury presents as mild disease (see later). To classify HIE as mild, moderate, or severe, each item evaluated varies according to the degree of severity (see Table 20.5 ). With this scoring system, a point is given to every item that corresponds to a level in the SIBEN score, with the diagnosis of HIE considered in the presence of three points or more. This scoring system has been evaluated in clinical practice in Brazil, but it remains under ongoing investigation for its utility in application to the evaluation of all infants requiring resuscitation who may benefit from therapeutic hypothermia. It remains the only current published scoring system to include the evaluation of all three grades of neonatal encephalopathy in the first 6 hours of life.
MILD | MODERATE | SEVERE | |
---|---|---|---|
Level of consiousness | Hyperalert | Lethargy | Stupor/Coma |
Spontaneous activity | Normal | Decreased | Not present |
Posture | Mild distal flexion | Marked distal flexion | Decerebrate |
Tone | Normal | Hypotonia | Flaccidity |
Suck | Weak | Weak or absent | Not present |
Moro | Strong | Weak | Not present |
Pupils | Mydriasis | Miosis | Diverted/nonreactive |
Heart rate (HR) | Tachycardia | Bradycardia | Lack of HR variability |
Breathing | Spontaneous | Periodic | Apnea |
Seizures | Absent | Present—Frequent | Present—infrequent |
Temporal Evolution of the Neurological Syndrome
The temporal evolution of the neurological syndrome in the era before therapeutic hypothermia has been outlined in detail in previous editions of this book. This evolution is most readily apparent in severely affected infants. With less severe forms of HIE changes in the clinical syndrome may be less stereotyped, and thus careful serial clinical evaluation every hour over the first 6 to 12 hours of life may be more sensitive to the evolution.
With regard to the most severe form of neonatal encephalopathy, occurring in 20% of HIE, a clear evolution has been documented. Although the temporal evolution of the neurological syndrome is more complex in the infant undergoing therapeutic hypothermia because of sedation and response to hypothermia, the principles remain unchanged.
In the first hours after the insult , signs of presumed bilateral cerebral hemispheral disturbance predominate ( Table 20.6 ).
|
The severely affected infant is either deeply stuporous or in coma (i.e., not arousable and minimal, or no response to sensory input). Periodic breathing, or respiratory irregularity akin to this pattern, is prominent, which may be considered as a form of respiratory disturbance as a neonatal counterpart of Cheyne-Stokes respiration, which is observed in older children and adults with bilateral hemispheral disease. In one series, approximately 80% of infants with severe neonatal encephalopathy had abnormal breathing patterns, particularly periodic breathing. Those most severely affected may exhibit marked hypoventilation or apnea. Pupillary responses to light are intact, spontaneous eye movements are present, and eye movements with the oculocephalic response (doll’s eyes maneuver) are usually full. (Pupillary size is variable, although dilated reactive pupils tend to predominate in the less affected infants, and constricted reactive pupils are common in the more severely affected infants. ) Commonly, disconjugate eye movements are apparent. However, only in a few babies are eye signs of major brain stem disturbance seen. Fixed, midposition, or dilated pupils and eye movements fixed to the doll’s eyes maneuver and to cold caloric stimulation are unusual at this stage. If either of these signs is evident at this time, especially in the full-term infant, injury to the brain stem is likely. Most infants at this stage are markedly and diffusely hypotonic with minimal spontaneous or elicited movement. Less severely affected infants have a preserved tone. Others exhibit an increased tone, especially with prominent involvement of the basal ganglia.
Clinical seizure-like activity often occurs by 6 to 12 hours after birth in approximately 50% to 60% of the infants who ultimately have seizures. (This early onset of seizures is unlike the later onset, 19 to 28 hours, in infants with neonatal arterial ischemic stroke [see Chapter 21 ].) A major challenge occurs in the correct clinical recognition of seizures. In one recent study of staff observing high-risk newborns, only 9% of 526 electrographic seizures were identified by clinical observation, indicating an underdiagnosis of seizures . In addition, 78% of 177 nonictal events were incorrectly identified as seizures, indicating an overdiagnosis of seizures. Problematically, the more difficult to diagnose seizure types tend to occur more often than the more readily diagnosed seizure types in newborns. A study of 61 seizures in 24 newborns classified seizures by their most prominent clinical features. Clonic and tonic seizures, which might be more readily identified, only occurred in 20% and 8%, respectively, while orolingual, ocular, and autonomic features, which might be more difficult to identify, were the main features in 55%. These clinical features contrast with the high frequency of focal clonic seizures in infants with neonatal arterial ischemic stroke (see Chapter 21 ).
Thus, neonatal seizures in the setting of HIE are frequently subtle or clinically invisible. More recent studies using EEG in the assessment of infants with moderate-severe HIE and undergoing therapeutic hypothermia identified the median timing of onset of EEG seizure activity at 13 hours (interquartile range [IQR]: 11 to 22 hours), with maximal seizure burden at a median of 19 hours (IQR: 12 to 29 hours).
From approximately 12 to 24 hours , the infant’s level of consciousness changes in a variable manner ( Table 20.7 ).
|
Infants with severe disease remain deeply stuporous or in a coma. Infants with less severe disease may often begin to exhibit some degree of improvement in alertness. A recent report from Biselele et al. serially examined 21 infants with evidence of hypoxic-ischemic insult and found that in the first hour 70% of the infants that displayed neurological signs scored greater than 7 on the Thompson scale (see earlier), thereby permitting entry into therapeutic hypothermia; while at 6 hours only 20% of infants scored at this level. The authors warn that this “apparent” improvement may prevent infants that need to receive therapeutic hypothermia from being eligible, if their examination is delayed to close to 6 hours of life. Thus, it is regarded that in many of these infants, this improvement is more apparent than real , because the appearance of alertness may not be accompanied by visual fixation or following, habituation to sensory stimulation, or other signs of cerebral function. The notion of apparent rather than real improvement in such cases is supported further by the occurrence at this time of seizures (as noted earlier, median occurrence at 13 hours), apneic spells, jitteriness, and weakness. Apneic spells appear in approximately 50% of infants (65% in one series). Jitteriness develops in about one-fourth of infants and may be so marked that the movements are mistaken for seizures. Distinction can usually be made at the bedside (see Chapter 9 ). Infants with involvement of basal ganglia may exhibit an increase in their hypertonia, especially in response to handling. Many infants manifest definite, albeit not marked, weakness (see Table 20.7 ). Although precise correlation is often difficult, these infants appear from the clinical circumstances surrounding their insult to have sustained particularly marked ischemic insults. Full-term infants most often exhibit weakness in the hip–shoulder distribution, with more impressive involvement usually of the proximal extremities. Distinct asymmetry of these latter motor findings is unusual to elicit at this time, although a few full-term infants do exhibit weakness that is confined to or is clearly more severe on one side than on the other.
Between approximately 24 and 72 hours , the severely affected infant’s level of consciousness often deteriorates further, and deep stupor or coma may ensue ( Table 20.8 ).
|
Respiratory arrest may occur, often after a period of irregularly irregular (“ataxic”) respirations. Brain stem oculomotor disturbances are now more common. These usually consist of skew deviation and loss of responsiveness of the eyes to the doll’s eyes maneuver and to cold caloric stimulation. (Rarely, ocular bobbing may appear.) Pupils may become fixed to light in the mid or dilated position. Reactive but constricted pupils are more common in less severely affected infants. Babies who die with HIE most often do so at this time, particularly if the criterion is “brain death.” In one large series of infants who died after perinatal asphyxia and HIE, the median age of death was 2 days. The cause for the apparent delay in progression to brain death until this period is not known definitely, but delayed cell death has been documented in in vivo models and in neurons in culture (see Chapter 13 , Chapter 18 , Chapter 19 ). The importance of excitatory amino acids, calcium-mediated deleterious metabolic events, and free radical production has been detailed in Chapter 13 . Indeed, studies by MR spectroscopy in the asphyxiated human infant (see later) have documented a delayed deterioration of cerebral energy state. However, although delayed cell death most probably accounts for this clinical deterioration, consideration should also be given to the occurrence of frequent subclinical electrical seizures as the reason for the deterioration. Although EEG is required for this determination, the potential effectiveness of anticonvulsant therapy warrants the procedure.
Infants who survive to greater than 72 hours will at this point usually improve over the next several days to weeks; however, certain neurological features persist ( Table 20.9 ).
|
Although the level of consciousness improves, often dramatically, mild to moderate stupor continues. Disturbances of feeding are extremely common and relate to abnormalities of sucking, swallowing, and tongue movements. The power and coordination of the muscles involved (innervated by cranial nerves V, VII, IX, X, and XII) are deranged. A few infants require tube feedings for weeks to months, particularly those with involvement of deep nuclear gray matter and brain stem (see Prognosis ). In the large series studied by Brown and co-workers, 80% of infants required early tube feedings because of feeding difficulty. Generalized hypotonia of limbs is common, although hypertonia, particularly with the passive manipulation of limbs, is frequent on careful examination, especially among infants with prominent involvement of basal ganglia. The patterns of weakness discussed in the previous section become more readily elicited, although the weakness is rarely marked.
Diagnosis
The recognition of neonatal HIE depends principally on information gained from a careful history and a thorough neurological examination. The contributing role of certain metabolic derangements requires evaluation. Determination of the site or sites and extent of the injury is made by the history, neurological examination, EEG, and neuroimaging studies (ultrasonography, MRI).
History
Recognition of neonatal HIE requires awareness of those intrauterine situations that account for most cases. Thus, information should be sought regarding maternal disorders with an increased risk for peripartum HIE (see Table 20.2 ) and that could lead to uteroplacental insufficiency and disturbances of labor or delivery that could impair placental respiratory gas exchange or fetal blood flow. The value of fetal evaluation including electronic fetal monitoring, particularly when supplemented by fetal blood sampling to determine acid-base status, is discussed in Chapter 17 .
Neurological Examination
Recognition of the neurological signs outlined previously provides critical information concerning the presence, site, and extent of hypoxic-ischemic injury in the newborn infant. The neurological examination plays two critical roles. First, the systematic neurological examination in the first 6 hours of life may allow recognition by the clinician of the presence and severity of any neonatal HIE. This is essential to allow that infant to be eligible for potentially neuroprotective strategies, such as therapeutic hypothermia. Second, the regular and systematic neurological examination of the infant with encephalopathy over the first week of life carries very important information for establishing a prognosis (see the section on prognosis ).
Metabolic Parameters
Certain metabolic derangements may contribute significantly to the severity and qualitative aspects of the neurological syndrome, and the diagnostic evaluation should include evaluation of such derangements. Hypoglycemia, hyperammonemia, hypocalcemia, hyponatremia (inappropriate secretion of antidiuretic hormone [ADH]), hypoxemia, and acidosis are among the metabolic complications that may occur, often because of associated disorders, and that may exacerbate certain neurological features or add new ones.
Of particular interest in this context is the occurrence of hypoglycemia and its potential role in the accentuation of brain injury. In a detailed study of 185 infants with evidence of intrauterine asphyxia (cord pH < 7.00), fully 15% exhibited blood glucose concentrations lower than 40 mg/dL in the first 30 minutes of life. The hypoglycemia may relate, in large part, to enhanced anaerobic glycolysis and, therefore, glucose use, in an attempt to preserve cellular energy levels (see Chapter 13 ). By multivariate analysis, the odds ratio (OR) for an abnormal neurological outcome was 18.5 when infants with blood glucose levels lower than 40 mg/dL were compared with those with levels higher than 40 mg/dL. These data may have important implications for management (see later).
Hyperammonemia may occur in newborns with severe perinatal asphyxia. Although very uncommon, levels of approximately 300 to 900 µg/mL have been detected in the first 24 hours of life and are usually accompanied by elevated serum glutamic oxaloacetic transaminase levels. Clinical correlates may be difficult to distinguish from those secondary to HIE, although hyperthermia and hypertension have been frequent additions in patients with hyperammonemia. Clinical improvement is coincident with falling blood ammonia levels. The pathogenesis of the hyperammonemia is unclear, although a combination of increased protein catabolism, secondary to hypoxic “stress,” and impaired liver function, and therefore hepatic urea synthesis, is a good possibility (see Chapter 27 ). Recall that hepatic disturbance is a common feature of the systemic multiorgan dysfunction observed with intrauterine asphyxia (see earlier).
Other metabolic parameters have been studied and some may hold promise as measures of severity of the hypoxic-ischemic insult ( Table 20.10 ), although currently the precise sensitivity and specificity of these determinations require further study before general use is warranted. The metabolites and markers are best considered in terms of their relevance to energy metabolism, excitatory amino acids, free radical metabolism, inflammation, brain-specific proteins, and compounds from other organ systems that may have sustained hypoxic-ischemic injury (see Table 20.10 ). Thus, the early clinical detection of blood or cerebrospinal fluid (CSF) biomarkers might allow an earlier diagnosis compared with neuroimaging. This identification would allow the earlier initiation of intervention measures to improve neonatal survival and reduce the degree of brain injury. In summary, such biomarkers could be important for diagnosis of neonatal HIE, selection of intervention, determination of efficacy, as well as assessment of the severity of illness and the estimation of prognosis.
DETERMINATION | BODY FLUID |
---|---|
Energy metabolism | |
Glucose | Blood |
Lactate | Blood, CSF |
Lactate/creatinine ratio | Urine |
Lactate dehydrogenase | CSF |
Excitatory amino acids | |
Glutamate | CSF |
Aspartate | CSF |
Glycine | CSF |
Free radical metabolism | |
Hypoxanthine | Blood, urine |
Uric acid | Blood, urine |
Nonprotein-bound iron | Blood |
Protein carbonyls | CSF |
Isoprostanes | CSF |
Ascorbic acid | CSF |
Arachidonate metabolites | CSF |
Nitric oxide | Blood, CSF |
Antioxidant enzymes | CSF |
Inflammatory markers | |
Interleukin-6 | Blood, CSF |
Interleukin-10 | CSF |
Interleukin-1 beta | Blood |
Tumor necrosis factor-alpha | Blood, CSF |
Brain-specific proteins | |
Neuron-specific enolase | Blood, CSF |
Neurofilament protein | CSF |
Protein S-100 | Blood, urine, CSF |
Glial fibrillary acidic protein | Blood, CSF |
Creatine kinase-BB | Blood, CSF |
Other | |
Erythropoietin | Blood |
Nerve growth factor | CSF |
Cyclic adenosine monophosphate | CSF |
Concerning energy metabolism , perinatal asphyxia has been associated with hypoglycemia, elevated lactate in blood and CSF, elevated lactate/creatinine (L/C) ratio in urine, and elevated lactate and hydroxybutyrate dehydrogenases in CSF. Of these, the value of detection of early hypoglycemia was discussed earlier. Of particular interest is the ratio of L/C in urine. In a study of 40 infants with evidence of intrapartum asphyxia, the mean (±SD) ratio within 6 hours of life was 16.8 ± 27.4 in the asphyxiated infants who subsequently developed the clinical features of HIE versus 0.2 ± 0.1 in those who did not develop encephalopathy, and 0.09 ± 0.02 in normal infants. Moreover, the ratio was significantly higher in the infants who had neurological sequelae at 1 year (25.4 ± 32.0) than in those with favorable outcomes (0.6 ± 1.5). The degree of elevation of lactate in blood at 30 minutes of life also may be a useful predictor of the severity of perinatal asphyxia. A more recent study of L/C in urine by proton nuclear MR spectroscopy within 6 and 24 hours after birth in 50 normal infants and 50 infants with asphyxia who developed HIE showed that the L/C ratio was elevated among asphyxiated neonates in the first 6 hours after birth to 11-fold greater than in normal neonates ( P = .0001). This ratio decreased to 1.5 ± 0.55 for asphyxiated cases over the first 24 hours after birth, fivefold greater than in the control group ( P = .0001). The severity of asphyxia correlated with the greater L/C ratio among cases ( P = .0007). The sensitivity and specificity of the L/C ratio were 96.1% and 100%, respectively. This measure is not yet used in routine clinical practice for the detection or confirmation of HIE.
Concerning excitatory amino acids , elevations of the excitotoxic amino acids glutamate, aspartate, and glycine (through the N -methyl- d -aspartate [NMDA] receptor) have been observed in CSF in the first day of life (see Table 20.10 ). Correlations with severity of HIE have been shown. Clinical observation showed increased levels of serum glutamate after neonatal HIE insult. Within 24 hours, the increase of glutamate was significant, and reached a peak at day 3 of postnatal life. At day 7 (recovery period) the levels returned to normal, and serum glutamic acid concentrations were closely related to the severity of HIE.
Concerning free radical metabolism , many studies support the involvement of reactive oxygen and nitrogen species in the final common pathway to cell death with neonatal HIE (see Table 20.10 ). These studies have shown elevations in sources of free radicals (e.g., hypoxanthine, non-protein-bound iron, arachidonate metabolites), indicators of lipid peroxidation (e.g., isoprostanes) or oxidized proteins (e.g., protein carbonyls), and markers of free radical use (e.g., ascorbic acid, antioxidant enzymes). Of particular note in clinical studies is superoxide dismutase (SOD), an antioxidant enzyme that removes the oxygen free radical, superoxide, to protect cells from free radical damage (see Chapter 13 ). Its activity level reflects the oxygen free radical scavenging capacity. Glutathione peroxidase, a second key antioxidant enzyme, detoxifies hydrogen peroxide, the product of action of SOD. In addition, also frequently studied is the lipid peroxidation product of free radical activity, malondialdehyde (MDA), which reflects the extent of oxidative damage to cells. Thus, excess free radicals consume SOD, produce a large amount of MDA, promote the release of inflammatory factors in brain tissue, induce nerve cell apoptosis, and increase permeability of the blood-brain barrier in neonatal HIE. A study of 50 cases of asphyxiated full-term newborns found that serious asphyxia resulting in the death of newborns with HIE was associated with concentrations of MDA and glutathione peroxidase in plasma and CSF that were significantly higher than in infants who survived. In neonatal HIE with epilepsy, serum MDA concentrations were significantly higher than in HIE without seizures. Moreover, serum MDA concentrations were increased with the degree of brain injury, which was confirmed by imaging analysis. Clinical studies found that in the acute stage of HIE, serum SOD concentrations were significantly decreased compared to those of a healthy control group, and MDA concentrations were significantly increased.
Concerning inflammatory markers , related potentially to hypoxic-ischemic or intrauterine infection or both, elevations of certain cytokines (interleukin [IL]-6, IL-10, IL-1beta, IL-18, ICAM-1, P-selectin, and tumor necrosis factor-alpha) have been documented in blood and CSF in both term and preterm infants (see Table 20.10 ). The degree to which the elevations in cytokines are primary or secondary is unclear (see Chapter 13 ). A recent review of the role of inflammation in the exacerbation and recovery from hypoxic-ischemic injury outlines the key mediators.
Concerning brain-specific proteins , specific components of neurons (neuron-specific enolase, neurofilament protein, creatine kinase-BB [CK-BB]) and astrocytes (S-100, glial fibrillary acidic protein, CK-BB) have been studied in blood and CSF to detect evidence of neuronal and glial injury. In general, elevations of these markers in blood or CSF in the first hours of life after perinatal asphyxia have correlated approximately with the severity of clinical and brain imaging findings. However, the value of studies of blood is tempered somewhat by the finding of S-100 and neuron-specific enolase (NSE) in placenta; this suggests that these molecules are not entirely brain specific. A recent study of infants receiving hypothermia treatment for neonatal HIE demonstrated abnormal changes in blood NSE that correlated with brain injury on neuroimaging. However, findings in relation to the severity of the injury remain variable.
Available data suggest that the determination of CK-BB is a very sensitive indicator of brain disturbance. However, the extreme sensitivity of the indicator in blood impairs the specificity of the measure because variable but appreciable proportions of infants with elevated concentrations of CK-BB in cord blood or neonatal blood samples have no evidence of irreversible brain injury and have a normal neurological outcome. However, two studies of the concentrations of CK-BB in CSF suggested greater specificity as well as sensitivity concerning identification of hypoxic-ischemic brain injury than with determination of blood CK-BB concentrations (see Table 20.10 ).
Concerning other markers , elevations of erythropoietin in blood and nerve growth factor and cyclic adenosine monophosphate in CSF have been documented after perinatal asphyxia (see Table 20.10 ). The value of these markers and the significance of their elevations remain to be established.
Currently, none of the markers has been established to be of sufficiently high sensitivity and specificity to be appropriate for general clinical use. However, several appear to be promising (see Review.)
Lumbar Puncture
A lumbar puncture should be performed on any infant with HIE in whom the diagnosis is unclear. It is particularly important to rule out other potentially treatable intracranial disorders (e.g., early-onset meningitis) that may mimic the clinical features of HIE.
Electroencephalogram
The EEG changes in HIE may provide valuable information concerning the severity of the injury. Although a considerable variety of tracings may be observed, the most common evolution of EEG changes in severe HIE is depicted in Fig. 20.1 .

The initial alteration is voltage suppression and a decrease in the frequency (i.e., slowing) into the delta and low theta ranges. Within approximately 1 day, and often less, an excessively discontinuous pattern appears, characterized by periods of greater voltage suppression interspersed with bursts, usually asynchronous, of sharp and slow waves. Some infants exhibit multifocal or focal sharp waves or spikes at this time, often with a degree of periodicity. Over the next day or so, the excessively discontinuous pattern may become very prominent, with more severe voltage suppression and fewer bursts, now characterized by spikes and slow waves. This burst-suppression pattern is of ominous significance, especially in the full-term infant (see Chapter 10 ). However, it is critical to recognize that excessively discontinuous patterns with prolonged interburst intervals (IBIs), which are not as severe as classic burst-suppression patterns, nevertheless also are associated with an unfavorable outcome (see the later section on prognosis and Chapter 10 ). Indeed, in one large series of infants, only 16% of excessively discontinuous tracings (in patients with a generally unfavorable outcome) exhibited burst-suppression patterns by classic definition. Notably, however, as many as 50% of asphyxiated term infants with a burst-suppression pattern identified by amplitude-integrated EEG (aEEG) in the first hours of life develop normal or nearly normal tracings within 24 hours (see later). In the severely affected infant, the excessively discontinuous EEG may then evolve into an isoelectric tracing and a hopeless prognosis. Caution in the interpretation of apparent isoelectric tracings in the newborn not undergoing hypothermia therapy, especially in the first 10 hours of life , is indicated by the findings of Pezzani and co-workers, which showed that of 17 asphyxiated newborns with isoelectric or “minimal” background activity in the first 10 hours, one was normal and one exhibited only epilepsy on follow-up (15 of the 17 died in the neonatal period). In general, those asphyxiated infants whose EEG tracings revert to normal within approximately 1 week have favorable outcomes.
aEEG is a commonly applied method for continuous monitoring of electrical activity in the newborn (see Chapter 10 ) and has considerable value in the assessment of the encephalopathic term newborn ( Fig. 20.2 ). This approach has been crucial in the selection of infants for treatment with mild hypothermia (see later). The most useful tracings for detection of severe encephalopathy have been continuous low-voltage, flat, and burst-suppression tracings. Positive predictive values (PPVs) for an unfavorable outcome with such tracings in the first hours of life are 80% to 90% (see the later section on prognosis ). Of infants with these marked background abnormalities, 10% to 50% may normalize within 24 hours. Rapid recovery is associated with a favorable outcome in 60% of cases.

Although the aEEG acquired within the first 6 hours of age has been considered one of the best predictors of neurological outcome at 18 months in infants with neonatal HIE who did not receive hypothermia therapy, since the widespread use of hypothermia therapy, the predictive value of early aEEG has changed, and infants have been shown to have a normal neurological outcome if the aEEG background voltage activity recovers by 48 hours. In a recent meta-analysis of nine studies with 520 infants treated with therapeutic hypothermia for moderate or severe HIE, the predictive value of an abnormal tracing on aEEG, acquired at 6, 24, 48, and 72 hours of age, was examined. The authors found that (1) a persistent, severely abnormal aEEG background at 48 hours of age or beyond predicted an adverse outcome (PPV value 85% and diagnostic OR 67 at 48 hours); and (2) at 6 hours of age, the aEEG background in hypothermia-treated infants had a good sensitivity at 96% (95% confidence interval [CI], 89% to 97%) but low specificity at 39% (95% CI, 32% to 45%).
Continuous monitoring of conventional EEG with portable equipment has been found to be particularly useful in the identification of seizure activity (see Chapter 12 ). Early detection of the seizures and the evaluation of response to anticonvulsant therapy are facilitated by modern portable monitoring systems. EEG data can assist in the determination of whether clinical events are correlated with electrical seizures requiring anticonvulsant medication or with nonepileptic events in which anticonvulsant medication administration can be avoided. As discussed earlier, some seizures have readily identifiable clinical manifestations (i.e., clonic or tonic components), while many seizures have more subtle manifestations (i.e., orolingual, ocular, or autonomic). The most comprehensive guideline on continuous EEG monitoring in the newborn was produced in 2011 by the American Clinical Neurophysiology Society. The guideline was created to standardize care and define the best neuromonitoring practices in the neonatal population, while recognizing that not all recommendations would be feasible or applicable across institutions. The guideline recommendations included that (1) electrodes be placed using the International 10 to 20 system with additional electrocardiogram, respiratory, eye, and electromyography leads; (2) at least 1 hour of recording be assessed to adequately assess cycling through wakefulness and sleep; (3) high-risk newborns be monitored for at least 24 hours to screen for the presence of electrographic seizures; and (4) in newborns with seizures, monitoring occur during seizure management and for an additional 24 hours after the last electrographic seizure. Video EEG recording was recommended for 24 hours rather than a briefer EEG recording because many newborns will not have seizures in the first hour of recording but will experience electrographic seizures within the first day. A statement from the American Academy of Pediatrics recommends that centers performing therapeutic hypothermia in newborns with HIE have either aEEG or conventional EEG available for seizure identification. This approach provides insight not only into potentially treatable conditions (frequent, clinically silent seizures) but also into the status of the cerebral hemispheres in an infant who is heavily sedated or therapeutically paralyzed.
The type of EEG abnormality may indicate a specific pathological variety of hypoxic-ischemic brain injury ( Table 20.11 ).
EEG PATTERN | TYPE OF HYPOXIC-ISCHEMIC BRAIN INJURY |
---|---|
Excessive discontinuity, burst suppression, persistent marked voltage suppression, isoelectric EEG pattern | Diffuse cortical and thalamic neuronal necrosis |
Excessive sharp waves: positive vertex or rolandic, positive frontal, and negative occipital sharp waves | Periventricular leukomalacia (also periventricular hemorrhagic infarction; see Chapters 16 and 24 ) |
Focal periodic lateralized epileptiform discharges | Focal cerebral ischemic necrosis (infarction) |
Diffuse and severe abnormalities (excessive discontinuity with prolonged IBI, burst suppression, marked voltage suppression, isoelectric EEG) are observed most commonly with diffuse cortical neuronal necrosis. Involvement of thalamus may also be important (see Chapter 10 ). a
a References .
The particular value of serial EEG in assessment of the asphyxiated infant is pronounced. A single EEG study, particularly during the acute phase of the disease, may suggest a more ominous outcome than do subsequent EEG studies (see Chapter 10 ). Focal periodic epileptiform discharges are characteristic of focal cerebral infarction ; in one series, approximately 90% of infants with such discharges had infarctions (see Chapter 21 ).
The role of the EEG in the assessment of brain death in the asphyxiated newborn has not been delineated decisively. Thus, an isoelectric EEG can be observed in infants with cerebral neuronal necrosis but not death of the entire brain (i.e., brain death). Conversely, persistent EEG activity for many days has been documented in infants with clinical and radionuclide evidence of brain death. Currently, the guidelines of the Task Force for the Determination of Brain Death in infants between the ages of 7 days and 2 months requires two clinical examinations indicative of loss of all cerebral and brain stem function and two isoelectric EEG tracings carried out according to standardized techniques separated by 48 hours. Although data are limited, a 72-hour observation period for term infants less than 7 days of age appears warranted in most cases and only when the cause of the coma is unequivocally established.
Neuroimaging Evaluation
Neuroimaging is used to identify the key neuropathologies, as outlined in Chapter 18 . These include: (1) selective neuronal necrosis, including three basic patterns, that is, diffuse injury, cerebral cortex-deep nuclear injury, and deep nuclear-brain stem injury; (2) parasagittal cerebral injury; (3) periventricular leukomalacia (see Chapter 16 ); and (4) focal ischemic necrosis and stroke (see Chapter 21 ). The accurate diagnostic application of neuroimaging modalities in the newborn infant with HIE is related to the level of radiological expertise for the acquisition and interpretation of the studies, regardless of the neuroimaging method. The methods of acquisition and level of experience in the interpretation of neuroimaging studies in the newborn can vary greatly between institutions. Integration of neonatal, neuroradiological, and radiological physicians by joint conference and review of case materials on a regular basis (e.g., weekly) will assist in improved communication and the application of modern neuroimaging methods. In addition, expert interpretation at a center of excellence in perinatal and neonatal neurology should be requested.
Cranial Ultrasound
Cranial ultrasound remains the most commonly used neuroimaging modality in the neonatal intensive care unit (NICU) setting, and is commonly applied to the term infant with neonatal encephalopathy. In the Vermont Oxford Neonatal Encephalopathy Registry, cranial ultrasound was acquired in nearly 40% of all infants with neonatal encephalopathy on a median of day 4 of life. Cranial ultrasound may be the only imaging modality possible if an infant is too clinically unstable to transport from the neonatal intensive care unit. Cranial ultrasound is sensitive for parenchymal hemorrhage, ventricular size, gross brain malformations, and cystic changes in the brain parenchyma (see Chapter 10 ). It is less sensitive for smaller and more subtle abnormalities within the brain, including cerebral cortical or brain stem neuronal disease, noncystic white-matter abnormalities, and minor cerebral dysgenesis. It is a very useful screening evaluation in the term infant, with encephalopathy; cerebral dysgenesis has been identified in approximately 2% to 4% of infants who had been diagnosed with hypoxic-ischemic injury ( Table 20.12 ). Cranial ultrasound is often used to assess the presence of slit-like ventricles, or sulcal effacement related to cerebral edema, and hemispheric or basal ganglia echodensity. Cranial ultrasound also can detect severe deep nuclear gray matter injury ( Figs. 20.3 and 20.4 ). However, cranial ultrasound lacks sensitivity in defining the full extent of the cerebral lesions, even in severe encephalopathy, and particularly in the first 24 hours of life. Because of the delay in the evolution of cranial ultrasound abnormalities by a minimum of 48 hours, detection of pronounced abnormalities on the first day of life may assist in identifying established severe HIE originating before the onset of labor.
STUDY | COWAN 2003 | MILLER 2005 | OKEREAFOR 2008 | CHAU 2009 | STEINMAN 2009 | RUTHERFORD 2010 | VERMONT OXFORD 2014 | CHEONG 2012 | MARTINEZ-BIARGE 2010 2011 | |
---|---|---|---|---|---|---|---|---|---|---|
Inclusion criteria | Either fetal heart rate monitoring abnormalities or cord pH <7.1 or delayed respiration or 5-min Apgar <7 or Multiorgan failure | One of: Apgar score ≤5 at 5 min, pH <7.1, BD >10 | Acute sentinel event: umbilical cord prolapsed (19%), uterine rupture (23%), placental abruption (46%) | Neonatal encephalopathy and one of “fetal distress” cord acidemia, 5-min Apgar ≤5, or both | One of: Apgar score ≤5 at 5 min, pH <7.0, BD >10 | Neonatal encephalopathy and abnormal EEG and cord pH <7.0 or delayed respiration or 10-min Apgar <5, or both | Seizures or stupor Coma or hypothermia or Apgar at 5 min <3 | Two of: Apgar score ≤5 Need for mechanical ventilation at 10 min pH <7.0, BD >12 | Term neonatal encephalopathy and MRI in first 6 weeks Apgar score ≤5 at 5 min or pH <7.1, or both “fetal distress” | |
Number of infants | 245 | 173 | 48 | 48 | 64 | 67 no hypothermia | 1,074 | 61 no hypothermia | 425 | |
Day of MRI scan (range) | 14 days of birth | 6 days (1–24) | 10 days | 72 h | 14 days | 8 days (2–30) | 7 days | 6 days (3–8) | 10 days (2–42) | |
No abnormality | 16% | 30% | 33% | 40% | 20% | 18% | 13% | See cortical injury | ||
Basal ganglia/thalamus | 80% | 25% | 75% any 65% moderate-severe | 31% | 33% | 80% any 58% moderate-severe | 30% | 36% moderate-severe | 41% | |
Cortical injury | 10% | 45% | 58% | 36% | 45% | 63% | 15% | 28% | 44% ( n = 186) normal or white matter/cortical | |
White-matter injury | 5% | Not recorded | Not recorded | 21% | 15% | 84% any 13% severe | 25% | 23% | See cortical injury | |
Other diagnosis | 4% | None noted | None noted | Arterial infarction in 10% | 4% | 2% | 11% from total of 555 |


Computed Tomography
Computed tomography (CT), in medical centers without ready access to MRI, has some value in the initial evaluation of the infant with HIE. As we discuss later, MRI is far preferable. CT does have several useful characteristics, including high sensitivity for the detection of acute hemorrhage and bone abnormalities, a short examination time, and wide availability. These features are useful in the evaluation of acute brain pathology, particularly in the setting of traumatic brain injury. However, in the setting of HIE, MRI is more sensitive, particularly for injury to the cerebral cortex, deep nuclear gray matter, and cerebral white matter. These characteristics are demonstrated by the results from neuroimaging of 1421 term infants with neonatal encephalopathy in the Vermont Oxford Neonatal Encephalopathy Registry from 2006 to 2008 ( Table 20.13 ).
ULTRASOUND | CT | MRI | |
---|---|---|---|
Number of infants | 2006/4111 (48.8) | 933/4107 (22.7) | 2690/4109 (65.5) |
Day of life at first scan, median (interquartile range) | 2 (1–3); N = 2001 | 2 (2–3); N = 928 | 6 (4–8); N = 2682 |
Any reported abnormality | 642/1985 (32.3) | 552/930 (59.4) | 1798/2676 (67.2) |
Intraventricular hemorrhage | 171/2001 (8.5) | 110/930 (11.8) | 220/2686 (8.2) |
Extraaxial hemorrhage | 59/2003 (2.9) | 321/927 (34.6) | 487/2686 (18.1) |
Parenchymal hemorrhage | 90/2001 (4.5) | 125/929 (13.5) | 292/2687 (10.9) |
Deep nuclear gray matter abnormality | 140/1994 (7.0) | 65/926 (7.0) | 603/2671 (22.6) |
Cystic white matter injury | 43/1997 (2.2) | 24/928 (2.6) | 131/2677 (4.9) |
Diffuse white matter injury | — | — | 628/2674 (23.5) |
Venous or arterial occlusion | 22/1980 (1.1) | 54/925 (5.8) | 183/2657 (6.9) |
Ventriculomegaly | 84/2004 (4.2) | 39/929 (4.2) | 92/2687 (3.4) |
Cerebellar injury | 21/1986 (1.1) | 29/929 (3.1) | 137/2677 (5.1) |
Brain stem injury | — | 7/927 (0.8) | 126/2677 (4.7) |
Diffuse cortical signal abnormality | — | — | 572/2673 (21.4) |
Parasagittal watershed injury | — | — | 285/2665 (10.7) |
Absent posterior limb of the internal capsule | — | — | 114/2659 (4.3) |
Other abnormality | 329/2000 (16.5) | 190/931 (20.4) | 588/2686 (21.9) |
The advantage of MRI, relative to CT, was further demonstrated by a comparison of the same infants undergoing direct imaging comparisons on the same day in this cohort ( Table 20.14 ). Particularly apparent is the superiority of MRI in the diagnosis of deep gray matter injury and cerebral parenchymal injury. These data are consistent with another report comparing CT scans with MRI on day 3 of life in the setting of acute neonatal encephalopathy ( Fig. 20.5 ). In this study of 48 term born infants with HIE, it was noted that the extent of cortical injury and focal-multifocal lesions, such as strokes and white matter injury, was less apparent on CT than MRI, particularly diffusion-weighted MRI (DWI). Of particular importance, in addition to its limited diagnostic role, there are increasing concerns regarding the impact of radiation exposure from CT on the developing brain (i.e., the risk of future malignancy and later cognitive impairments). These concerns are outlined in Chapter 10 . Until further data are available, this neuroimaging technique should be restricted to select settings in which the information obtained from the imaging study is clearly of benefit to the patient and cannot be readily obtained with some other modality. Examples would include infants with severe head trauma at risk for major epidural bleeding and those who require more definitive imaging in a very brief period because of severe clinical instability.
ULTRASOUND VERSUS CT | ULTRASOUND VERSUS MRI | CT VERSUS MRI | ||||
---|---|---|---|---|---|---|
ULTRASOUND | CT | ULTRASOUND | MRI | CT | MRI | |
Intraventricular hemorrhage | 2/43 (5) | 4/43 (9) | 3/47 (6) | 5/47 (11) | 8/70 (11) | 9/70 (13) |
Extraaxial hemorrhage | 2/42 (5) | 10/43 (23) | 1/46 (2) | 11/47 (23) | 17/69 (25) | 14/70 (20) |
Parenchymal hemorrhage | 5/42 (12) | 7/43 (16) | 3/46 (7) | 6/47 (13) | 10/69 (14) | 13/70 (19) |
Subependymal hemorrhage | 1/42 (2) | 2/43 (5) | 1/46 (2) | 2/47 (4) | 0/69 (0) | 1/70 (1) |
Deep nuclear gray matter abnormality | 1/42 (2) | 4/42 (10) | 4/46 (9) | 11/47 (23) | 8/69 (12) | 18/70 (26) |
Cystic white matter injury | 0/42 (0) | 2/42 (5) | 0/46 (0) | 4/47 (9) | 1/69 (1) | 2/70 (3) |
Venous or arterial occlusion | 0/42 (0) | 1/42 (2) | 0/46 (0) | 2/47 (4) | 12/69 (17) | 13/70 (19) |
Cerebellar injury | 1/42 (2) | 3/43 (7) | 0/46 (0) | 4/47 (9) | 1/69 (1) | 5/70 (7) |
Brain stem injury | 6/69 (9) | 6/70 (9) |

Magnetic Resonance Imaging
MRI provides the highest sensitivity for both anatomical and functional detail and also offers an array of imaging options that can be tailored to the specific clinical question (see later). MRI, however, does have some drawbacks compared with other imaging modalities, particularly in the neonatal period. Unlike cranial ultrasound, patients must typically be transported to a radiology suite from the neonatal intensive care unit for MRI, which may pose some risk to those infants who are unstable. Safely imaging encephalopathic neonates is a unique challenge. Studies have shown that at least 20% of term-born infants with severe HIE cannot be safely transported to the MRI scanning suite because of the severity of their illness. Further information on MRI in infants and the techniques applied in MRI are detailed in Chapter 10 .
MRI has been used in a large number of studies of neonatal HIE. The entire spectrum of hypoxic-ischemic brain injury has been demonstrated ( Table 20.15 ). The major findings by MRI are outlined in Table 20.16 .
DIAGNOSTIC TECHNIQUE | |||
---|---|---|---|
NEUROPATHOLOGICAL TYPE | MRI | CT | ULTRASOUND |
Selective neuronal necrosis: cerebral cortical | ++ | + | – |
Selective neuronal necrosis: basal ganglia and thalamus | ++ | + | + |
Selective neuronal necrosis: brain stem | ++ | ± | – |
Parasagittal cerebral injury | ++ | + | – |
Focal and multifocal ischemic brain injury | ++ | ++ | + |
Periventricular leukomalacia | ++ | + | ++ a |
a Very useful for detection of focal component; not useful for detection of diffuse component or “noncystic periventricular leukomalacia” (see text).
Major conventional MRI findings in first week |
|
a See text for references and more details concerning timing of findings.
Conventional MRI shows the abnormalities in the first 3 to 4 days, but generally not on the first day. However, DWI, based on the molecular diffusion of water, is not only more sensitive than conventional MRI, but also shows abnormalities earlier, often in the first 24 to 48 hours after birth (see later discussion, Fig. 20.6 ). The correlates of the MRI findings with the neuropathological states described in Chapter 18 are apparent ( Figs. 20.7 to 20.14 ). Thus, selective cerebral cortical neuronal injury is manifested by loss of the cerebral gray-white matter differentiation and by cortical high signal (highlighting) on T1-weighted (T1W) or fluid-attenuated inversion recovery (FLAIR) images at the sites of particular predilection, the parasagittal perirolandic cortex, and the depths of sulci (see Fig. 20.7 ).









Decreased diffusion (increased signal) is seen on DWI (see Fig. 20.8 ).
Selective cerebral cortical neuronal injury is usually accompanied by involvement of basal ganglia (especially dorsal putamen) and thalamus (especially lateral thalamus; see Fig. 20.9 ). In the unusual cases of principally deep nuclear and brain stem involvement, as with severe, acute asphyxial insults, high signal (T1W or FLAIR) is seen in the brain stem tegmentum as well as in the basal ganglia. DWI is more sensitive for detection of cerebral cortical and deep nuclear involvement (see Fig. 20.10 ).
In one series of 173 encephalopathic term newborns, predominant involvement of perirolandic cortex and basal ganglia/thalamus was observed in 44 (25%) and in an additional 24 (14%) in association with predominant involvement of parasagittal regions. Parasagittal cerebral injury is seen readily as areas of increased signal (T1W and FLAIR) in the parasagittal cerebral cortex and subcortical white matter (see Figs. 20.11 and 20.12 ).
The relative distribution of the abnormalities among many large-scale MRI studies has varied somewhat because of different schemes for analysis (see Table 20.12 ). a
a References .
In general, approximately 15% to 30% of MRI scans have been normal. Lesions in basal ganglia/thalamus, either predominantly or more commonly accompanying other areas of involvement, are present in approximately 40% to 80% of cases. Because the lesions in basal ganglia and thalamus often are microscopic in size, some instances of involvement likely may not be detected by MRI. Abnormalities of parasagittal (watershed) white matter and cortex are present in approximately 40% to 60%. The involvement of cortex in the watershed lesions also is likely an underestimate, because the cortical involvement typically is laminar (especially layers 3 and 5), and the entire cortical thickness in the human newborn is only approximately 2 mm. In more severe cases the classic watershed parasagittal cerebral injury involving cortex and subcortical/central white matter is readily apparent. Involvement of basal ganglia and brain stem preferentially occurs in approximately 10% to 20% of cases, usually following a catastrophic sentinel event (see later). Preferential involvement of periventricular/central white matter, similar to periventricular leukomalacia of premature infants, is noted as a dominant feature in only approximately 15% of cases, and occurs especially in infants of somewhat lower gestational age (late preterms), or in the context of hypoglycemia or prolonged cardiovascular instability (e.g., congenital heart disease).Although many of the lesions just discussed are visualized well by conventional MRI, they are visualized better and, importantly, earlier by DWI (see Fig. 20.14 ). Increased signal on DWI, indicative of decreased water diffusion, has been shown in experimental models of focal cerebral ischemia and in adult stroke in the first 1 to 2 hours after the insult. Many studies of newborns with hypoxic-ischemic disease have demonstrated the superior sensitivity of DWI versus conventional MRI in delineating the site and extent of tissue injury early in the neonatal period. a
a References .
The DWI signal in neonatal HIE is influenced greatly by the timing of the scan and the region studied. The timing of DWI abnormality in asphyxiated term infants with presumed selective neuronal necrosis or parasagittal cerebral injury, or both, is shown in Fig. 20.15 .
Thus, although some infants exhibit abnormality on the first day, injury is generally underestimated at that time. The nadir for diffusion occurs between the second and third days. By 7 to 8 days, pseudonormalization is apparent and is probably related to recovery processes that ultimately lead to angiogenesis and other factors causing increased diffusion. Thus, the optimal time for detection of DWI abnormality in the most common varieties of hypoxic-ischemic disease in the term newborn is approximately 2 to 3 days (see Fig. 20.15 ). In adult human stroke (i.e., permanent occlusion), diffusion is decreased in the first hours after the insult and remains low until pseudonormalization occurs at 7 to 9 days. This time course is similar to that observed in animal models of permanent vascular occlusion. By contrast, in newborns, the insult usually is transient and is followed by reperfusion. In animal models of transient occlusion, during the occlusion diffusion decreases, whereas on reperfusion, diffusion recovers before a secondary decline many hours later, as in the usual asphyxiated human infant (see Fig. 20.15 ). The evolution of these diffusion changes appears to be altered by the commencement of therapeutic hypothermia , which is associated with a more protracted pseudonormalization of the diffusion coefficient, with full normalization requiring greater than 10 days. This more protracted course may reflect a slower evolution to cell death and thereby provide a longer window for a second neuroprotective agent. Further studies are needed to confirm this evolution of the diffusion findings in the setting of therapeutic hypothermia.
The importance of the region injured in the evolution of changes in diffusion is illustrated by the scans shown in Fig. 20.16 . Thus, in this unusual example of precise knowledge of the timing of the insult (postnatal cardiac arrest), decreased diffusion in basal ganglia and thalamus was apparent at 6 hours of age, but decreased diffusion did not appear in the cerebral cortex until 32 hours. Other investigators showed that although severe white matter injury is associated with early decreased diffusion, with more moderate white matter injury diffusion is normal or slightly increased early and increases in the ensuing days ( Fig. 20.17 ). A similar increase in white matter diffusion was observed in cerebral hypoxia–ischemia in the neonatal rat.


To summarize , MRI clearly provides superior imaging resolution for delineation of all hypoxic-ischemic lesions, both in the neonatal period and on follow-up (see Tables 20.15 and 20.16 ). DWI provides the capability for identification of injury by 24 to 48 hours after asphyxia in the term infant to assist in the early delineation of the nature and severity of cerebral injury.
Cerebral Metabolic-Hemodynamic Neurodiagnostic Studies
Neurodiagnostic studies that address changes in metabolism and physiology after perinatal hypoxic-ischemic insults include MR spectroscopy, positron emission tomography (PET), near-infrared spectroscopy, and other measures of the cerebral circulation (see Chapter 10 ). Of these, MR spectroscopy has proven most useful for diagnostic assessment and is emphasized here.
Magnetic Resonance Spectroscopy
MR spectroscopy has proven to be a diagnostic modality of particular importance in the evaluation of the infant with perinatal hypoxic-ischemic brain injury. Both phosphorus and proton MR spectroscopy are useful, although currently the more readily available proton MR spectroscopy is used most widely. Indeed, over the past few years at our institution, proton MR spectroscopy has joined DWI as part of the standard evaluation of infants evaluated by MR techniques for hypoxic-ischemic disease. The basic principles of phosphorus and proton MR spectroscopy and the normative data obtainable are described in Chapter 10 . The value of these techniques in the assessment of HIE in the term infant is summarized in Table 20.17 .
Phosphorus magnetic resonance spectroscopy |
|
Proton magnetic resonance spectroscopy |
|
Phosphorus Magnetic Resonance Spectroscopy.
Multiple studies of infants who sustained perinatal asphyxia, especially intrapartum, have focused on phosphorus-31 ( 31 P) spectra. The sequence of findings has been initially normal spectra (concentrations of phosphocreatine [PCr], inorganic phosphate [P i ], and adenosine triphosphate [ATP]) in the first hours after birth, followed by a decline in concentration of PCr and a rise in that of P i (and thus a decline in the PCr/P i ratio) over approximately the next 24 to 72 hours ( Fig. 20.18 ; see Table 20.17 ). In the most severely affected infants, ATP concentrations also decline at this time. Subsequently, spectra return to normal over the ensuing weeks, although the total 31 P signal may be reduced when marked loss of brain tissue has occurred. This sequence of events is directly reminiscent of the progression of the “delayed energy failure” described in Chapter 13 . This secondary energy failure correlates directly with the ultimate degree of cell death. Consistent with the experimental data, the severity of this delayed energy failure in human infants correlates closely with the severity of the neonatal neurological syndrome ( Fig. 20.19 ) and with the subsequent occurrence of neurological deficits (see later). The findings not only demonstrate the value of phosphorus MR spectroscopy in the early delineation of impairments of energy metabolism in the asphyxiated infant but also provide important prognostic information (see the section on prognosis ).


Phosphorus MR spectroscopy also is valuable in detection of a paradoxical postischemic increase in intracellular pH (pH i ). The evolution of this increase in the days to weeks after hypoxia-ischemia correlates with the degree of brain injury. This postischemic alkalinization may lead to cellular injury and appears related, in considerable part, to postischemic activation of the neuronal and glial sodium-hydrogen transporter. Consistent with this formulation, experimental data indicate a neuroprotective role for amiloride, a sodium-hydrogen exchange blocker, when administered after ischemia (see Chapter 13 and later).
Proton Magnetic Resonance Spectroscopy.
Proton MR spectroscopy (see Chapter 10 ) has been applied extensively to the study of infants with HIE. Although not all the reported observations are entirely consistent, important and consistent findings can be recognized (see Table 20.17 ). First, in the acute period, as early as a few hours after birth, elevation in cerebral lactate , often expressed as the ratio of lactate to N -acetylaspartate (NAA), creatine, or choline, can be detected (see Fig. 20.10A ). Indeed, detection of lactate by proton MR spectroscopy is a more consistent indicator of brain injury than is DWI (or other imaging modality) in the first hours after hypoxic-ischemic injury. Currently, MR spectroscopy may be considered as the most sensitive modality for detection of neonatal brain disturbance in the acute period. More data regarding sensitivity and specificity for structural injury are needed. During this early period, ratios of NAA to choline or creatine have been either unchanged or only slightly decreased. The elevated lactate is most pronounced in deep nuclear structures, especially basal ganglia and thalamus, with their high metabolic rate and propensity for hypoxic-ischemic injury. The acutely elevated lactate correlates with the severity of the neonatal neurological syndrome, the subsequent delayed energy failure ( Fig. 20.20B ), and the neurological deficits on follow-up (see the section on prognosis ). Lactate levels may remain elevated for weeks, perhaps in part because of enhanced glycolysis and lactate production by astrocytes. Second, after days to weeks, ratios of NAA to choline or creatine decline and reflect tissue injury. Recall from Chapter 10 that NAA is contained in neurons (and presumably axons) and in oligodendroglial precursors. Thus, the declines in NAA in both gray and white matter are not surprising. The severity of the decline in NAA correlates with the severity of subsequent neurological deficits. Glutamate levels also have been shown to be elevated in the first days of life in infants with severe HIE. This determination is more difficult than that for lactate or NAA and may not be as useful.

Positron Emission Tomography
Although PET is not a routine diagnostic procedure (for the reasons described in Chapter 10 ), the technique has provided major insight into the frequency, basic nature, and probable pathogenesis of the cerebral injury observed in asphyxiated term infants. Because experience with adult patients had indicated that measurements of regional cerebral blood flow (CBF) provided critical information concerning the topography of hypoxic-ischemic cerebral injury, PET was studied in a series of 17 asphyxiated term infants with the H 2 15 O technique to measure regional CBF. The infants had experienced primarily intrapartum asphyxia, exhibited the clinical syndrome described earlier, including proximal limb weakness, and were evaluated by PET during the acute period of their illness (i.e., the first week). The disturbance of regional CBF in the asphyxiated infants constituted a continuum of deviation from the normal (or nearly normal) pattern. The consistent and apparently unifying abnormality was a relative decrease in CBF to parasagittal regions, generally symmetrical and more marked posteriorly than anteriorly. The extent of the relative decreases in parasagittal CBF correlated directly with the severity of clinical manifestations.
The structural correlates of the decrease in CBF in parasagittal regions were elucidated in four infants by technetium brain scan and by neuropathology and consisted of parasagittal cerebral injury. Thus, our CBF findings by PET indicated that parasagittal cerebral injury is a common feature in neonatal HIE, at least in patients who survive the perinatal insult. This observation has been confirmed and amplified by subsequent studies with MRI (see earlier discussion).
Clinicopathological Correlations
The neurological correlates of HIE, as observed in the neonatal period and subsequently, are understood when one recalls the topography of the neuropathological lesions. Considerably more is known about the long-term neurological correlates of the several lesions than about the correlates in the newborn period. Indeed, in the latter instance, correlates must be made with some reservation. The reasons for the difficulty in establishing correlations relate primarily to the large degree of overlap in the occurrence of the basic lesions and to the heretofore imperfect definition of topography by available imaging studies. Although the overlap of the various lesions will be a persistent confounder, improvements in imaging, especially the use of MRI, have allowed better definition of the topography of the brain injury in the neonatal period. The latter now has allowed certain probable correlations to be made. In the following discussion, we review the major neuropathological lesions in terms of the neurological correlates in the newborn period and subsequent periods (i.e., neurological sequelae).
Selective Neuronal Necrosis
Neonatal Correlates
The neurological correlates in the neonatal period are diverse, as is the topography of the major neuronal injury ( Table 20.18 ).
TOPOGRAPHY OF THE MAJOR INJURY | NEUROLOGICAL FEATURES b | |
---|---|---|
NEONATAL PERIOD | LONG-TERM SEQUELAE | |
Cerebral cortex; basal ganglia; thalamus; reticular formation; brain stem nuclei, including inferior colliculus, cochlear nuclei, and motor nuclei of cranial nerves; cerebellum; anterior horn cells | Stupor and coma | Cognitive deficits c |
Seizures | Spastic quadriparesis | |
Hypotonia | Choreoathetosis c | |
Hypertonia-dystonia c | Dystonia c | |
Oculomotor disturbances d | Seizure disorder | |
Disturbed sucking, swallowing, and tongue movements d | Ataxia | |
Bulbar and pseudobulbar palsy d |
a As discussed in the text, three major forms of selective neuronal necrosis should be recognized: diffuse, cerebral cortical–deep nuclear, and deep nuclear–brain stem.
b All the neurological features may be seen to varying degrees in the diffuse form of selective neuronal necrosis.
c Common abnormalities in those infants with involvement of basal ganglia and thalamus.
d Common additional abnormalities in those infants with involvement of brain stem tegmentum (and usually associated with deep nuclear involvement).
Of the major varieties of selective neuronal necrosis (diffuse, cerebral cortical–deep nuclear, deep nuclear–brain stem, and pontosubicular necrosis [see Chapter 18 ]), tentative clinical correlates can be established for the first three. In the diffuse variety of selective neuronal necrosis , associated with very severe and prolonged insults, all levels of the neuraxis are affected. With this variety, we have attributed the derangement of the level of consciousness to the involvement of the bilateral cerebral hemispheres or the reticular activating system in the upper brain stem and diencephalon, including the thalamus. Indeed, in one careful series studied by MRI, the involvement of basal ganglia and thalamus was associated strongly with severe encephalopathy, including a decreased level of alertness. Seizures appear to relate to cerebral cortical injury, although some of the seizure phenomena, especially some of the tonic phenomena, may emanate from subcortical nuclear structures in basal ganglia, thalamus, or midbrain. The uncommon but dramatic occurrence of the syndrome of inappropriate ADH secretion or diabetes insipidus presumably relates to hypothalamic neuronal involvement. The hypotonia could relate to cerebral cortical or anterior horn cell disturbances or combinations of both. Electrophysiological evidence (e.g., fibrillations), as well as clinical data (hypotonia, absent deep tendon reflexes, weakness), support a role for anterior horn cell involvement, especially in severe, diffuse disease. The oculomotor abnormalities presumably relate primarily to the disturbance of cranial nerve nuclei (III, IV, and VI). The impairments of sucking (V), swallowing (IX and X), and tongue movements (XII) also are probably largely the basis of brain stem cranial nerve nuclear involvement. However, a contribution of corticobulbar disturbance to these deficits is possible. The facial appearance of an infant with striking brain stem involvement, proven neuropathologically, is shown in Fig. 20.21 .

With the cerebral cortical–deep nuclear variety of selective neuronal necrosis , associated with moderate to severe and relatively prolonged insults, the involvement of the cerebral cortex, the basal ganglia (especially the putamen), and the thalamus predominates (see Chapter 18 ). The major clinical difference from the syndrome just described is the occurrence of increased tone in many such affected infants. The hypertonia often increases with stimulation, especially manipulation, and has characteristics of dystonia. We have attributed this finding to extrapyramidal involvement, perhaps unmasked by the less severe injury to the pyramidal system that occurs with the diffuse variety of selective neuronal injury.
With the deep nuclear–brain stem variety of selective neuronal necrosis , associated with severe and abrupt insults, the involvement of the basal ganglia, thalamus, and brain stem tegmental neurons occurs, with relative sparing of the cerebral cortex. The major additional clinical correlates relate to brain stem injury and include ptosis, oculomotor disturbances, facial diparesis, ventilatory disturbances, and impaired sucking and swallowing.
Long-Term Correlates
The long-term neurological sequelae depend on the topography of the neuronal injury. With the diffuse variety of selective neuronal necrosis , intellectual retardation is nearly uniform and is the consequence principally of cerebral cortical injury (see Table 20.18 ). However, injury to the basal ganglia, thalamus, and cerebellum (see later) could play a role. (The possibility of impairment of subsequent cortical neuronal differentiation is raised by experimental data, but studies of human infants are lacking.) The spastic motor deficits could relate to cortical injury, although the relative roles of concomitant ischemic parasagittal cerebral injury and cerebral white matter injury/periventricular leukomalacia have not been elucidated. Seizure disorders, which develop in approximately 10% to 30% of infants with HIE (see Chapter 12 ), probably relate to cerebral cortical injury. Impairment of cortical visual functions occurs in severely affected infants, and cerebral cortical atrophy was reported to be the principal finding on CT in approximately 60% of such patients. MRI studies have emphasized the association of basal ganglia or thalamic lesions and cerebral white matter injury with impaired visual function in such infants. (The improvement of vision in as many as 50% of such infants over the first 2 years of life may reflect the operation of cortical organizational events, i.e., brain plasticity, as outlined in Unit I.) Disturbances of hypothalamic neurons presumably underlie the early sexual maturation that occurs in 10% of term asphyxiated infants with other signs of neurological disturbance. Impairments of sucking, swallowing, and facial movement may relate to nuclear injury (i.e., bulbar palsy), although some infants also exhibit the features of upper motor neuron injury (i.e., pseudobulbar palsy), probably cerebral in origin, such as “all-or-none smile” and fixed facial expression with drooling. Hyperactivity and impaired attentive capacities, particularly observable (unmasked?) in less affected patients, may relate to involvement of neurons of the reticular activating system, the basal ganglia, or the cerebellum. The substantial minority of infants with hearing deficits presumably may have involvement of dorsal cochlear nuclei (which subserve perception of higher frequency sounds) or of cochlea, or both. The involvement of the superior olivary nucleus and the inferior colliculus may contribute. Finally, the involvement of anterior horn cells may explain the characteristic persistence of hypotonia in the first months of life, and when it is severe, this topography of involvement may explain the unusual persistence into childhood of hypotonia and weakness (i.e., atonic quadriparesis or “atonic cerebral palsy”).
With the cerebral cortical–deep nuclear variety of selective neuronal necrosis , the major clinical features include not only the deficits attributable to cerebral cortical neuronal injury but also those related to the involvement of the basal ganglia and the thalamus, which are discussed separately later. With the deep nuclear–brain stem variety of selective neuronal necrosis , the additional clinical features relate not only to the basal ganglia–thalamic involvement, discussed separately later, but also to the brain stem injury. All surviving infants with this injury have prolonged difficulties with feeding, usually for many months and often requiring tube feeding. Approximately 20% to 30% require gastrostomy for feeding (see the later section on prognosis ). However, because of the relative sparing of cerebral cortex with this variety of selective neuronal injury, up to 50% of these patients have been found to exhibit normal cognition.
With involvement of basal ganglia and thalamus , whether as a component of the cerebral – deep nuclear variety of selective neuronal necrosis or the deep nuclear syndrome with brain stem involvement, subsequent extrapyramidal abnormalities are not uncommon. The neurodevelopmental, motor, and feeding outcomes of a large series of infants with basal ganglia and thalamic injury on MRI have been recently reviewed (see Prognosis ). Unknown numbers of such infants develop the neuropathological lesion status marmoratus (see Chapter 18 ), but the fundamental clinicoanatomical correlate is neuronal loss in the putamen and the thalamus, whether or not the final pathological appearance is that of status marmoratus. The essential anatomical combination for choreoathetosis and dystonia appears to be bilateral involvement of the basal ganglia and intact pyramidal tracts because, in one neuropathological series, the several patients without choreoathetosis had unilateral or bilateral sparing of the basal ganglia or degeneration of the pyramidal tracts. The thalamoputaminal involvement is different from the subthalamic nucleus and globus pallidus distribution in bilirubin encephalopathy, the other major neonatal disorder with subsequent choreoathetosis (see Chapter 26 ). Careful studies using MRI in infants with “dyskinetic” or “athetoid” cerebral palsy demonstrated the thalamoputaminal predilection in hypoxic-ischemic disease. Indeed, the thalamus was affected without putaminal involvement as often as with putaminal involvement, and more often than with the involvement of the putamen alone. Of particular interest and not readily explicable is the finding that the onset of the extrapyramidal abnormalities is not clearly apparent until after 6 to 12 months and often much later. Thus, most such infants develop overt choreoathetosis or dystonia, or both, between 1 and 4 years of age. Abnormal motor development and hypertonia commonly are obvious before this time (i.e., as early as 6 months of life).
An important percentage of children will not develop abnormal movements until as late as 7 to 14 years of age (see Table 20.19 ). In the largest reported series of the delayed-onset syndrome , the mean age of onset of choreoathetosis and dystonia was 12.9 years, and the mean duration of progression was 7 years ( Table 20.19 ). Four of the 10 patients studied had attained early developmental milestones at ages within the normal range. Although the children ultimately had mild to moderate motor disability, all were ambulatory. The only class of drugs with clear benefit was anticholinergic medication, perhaps reflecting that the relatively spared cholinergic neurons were responsible for the development of the extrapyramidal clinical phenomena.
|
Intellectual function often is relatively preserved in those infants with choreoathetosis. Thus, in the older literature, intellectual function in infants with “athetoid cerebral palsy,” presumably many or most of whom had putaminothalamic injury to a varying degree, was not noted to be markedly affected consistently. In the largest reported series of the delayed-onset extrapyramidal syndrome, 8 of 10 individuals had a normal intelligence quotient. The pathological substrate for the intellectual failure with injury to the basal ganglia and the thalamus presumably relates, in considerable part, to any associated hypoxic-ischemic cerebral cortical neuronal injury; indeed, in the large neuropathological series of Malamud, approximately 50% of patients with severe involvement (i.e., the pathological features of status marmoratus) exhibited neuropathological signs of cerebral cortical injury. However, these latter patients also manifested thalamic injury, and approximately one-third of patients with pathologically proven status marmoratus, and with impaired intellect, exhibited thalamic injury without significant involvement of the cerebral cortex. This finding suggests that the thalamic injury can play an important role in causing the intellectual deficits. MRI data support this contention (see the section on prognosis ). These observations have major implications for the role of the thalamus in the development of intellectual function .
The clinical correlates of the cerebellar vermian atrophy/hypoplasia that is a common sequela in term infants with hypoxic-ischemic disease (see Chapter 18 ) remain to be defined. This abnormality may contribute to varying degrees of motor incoordination, occasionally including overt ataxia. Cognitive and behavioral deficits also could be correlates of the cerebellar involvement (see later).
Parasagittal Cerebral Injury
Neonatal Correlates
The neurological correlates in the neonatal period include particularly weakness of proximal limbs, which is consistently more prominent in the upper rather than the lower extremities ( Table 20.20 ).
NEUROLOGICAL FEATURES | ||
---|---|---|
TOPOGRAPHY OF THE MAJOR INJURY | NEONATAL PERIOD | LONG-TERM SEQUELAE |
Cerebral cortex and subcortical white matter, superomedial (parasagittal) convexities, and posterior > anterior cerebrum | Proximal limb weakness upper > lower | Spastic quadriparesis |
Intellectual deficits (often “specific”) |
This pattern of weakness is readily predicted from the topography of the lesion ( Fig. 20.22 ). The topographical representation of the homunculus on the motor cortex indicates that the proximal extremities, upper more than lower, lie within the distribution of the necrosis of the cerebral cortical neurons and their descending corticospinal tract fibers in subcortical white matter. Other deficits referable to parasagittal cerebral injury are likely, but ready detection of such deficits in the newborn requires specialized clinical techniques. For example, careful analysis of “cortical” somesthetic-visual-auditory associations, functions residing within the areas of posterior cerebrum especially affected in parasagittal necrosis, has not been accomplished in the newborn. This topic is clearly important for future clinical research. The advances in electrophysiological, behavioral, and functional MR techniques for assessing such associative functions in the newborn (see Chapters 9 and 10 ) could be used effectively in this clinical setting. Indeed, the disturbances of visual- and somatosensory-evoked responses observed in asphyxiated infants appear to correlate with cerebral injury in the parasagittal cerebral distributions, affecting parieto-occipital regions in the former instance (visual-evoked responses) and parietal regions in the latter instance.

Long-Term Correlates
The long-term sequelae of parasagittal cerebral injury relate primarily to motor and cognitive function, particularly the latter. However, in general, subsequent deficits are less common than in infants who also exhibit deep nuclear injury (see the section on prognosis later). The motor deficits, when present, include particular involvement of proximal limbs, upper more than lower, as in the neonatal period (see Table 20.20 ). Although severely affected infants exhibit multiple cognitive deficits, many infants have exhibited “specific” intellectual deficits, such as disproportionate disturbances in the development of language or of visual-spatial abilities, or both. We believe these discrete intellectual deficits to relate particularly to the larger, posteriorly located lesions (i.e., in posterior parietal-occipital-temporal regions) that reside within areas of critical importance for many associative functions, especially those relating to auditory and visual input and output, and to a variety of visual–motor phenomena.
Prognosis
Precise determination of the prognosis in the term newborn who sustains a hypoxic-ischemic insult is hindered by the difficulties in determining the severity of the insult. As indicated earlier, most of the primary insults occur in utero, and the difficulties of determining the degree of hypoxemia and ischemia in the fetus are obvious. The value of electronic fetal monitoring and associated fetal blood sampling may be appreciable, but further advances in monitoring the status of the fetal brain clearly are needed (see Chapter 17 ). Because significant intrauterine (particularly intrapartum) hypoxic-ischemic insult is usually associated with depressed Apgar scores, correlation of outcome with the Apgar score also has been used for assessing the prognosis. The presence of a neonatal neurological syndrome is a crucial indicator of a perinatal insult with the potential to cause neurological injury. Moreover, certain specific aspects of the neurological syndrome (e.g., seizures and duration of abnormalities) are useful in estimating outcome. Finally, selected neurodiagnostic studies, such as EEG, evoked potentials, ultrasound, CT, and MRI, are also of proven prognostic value. Value for MR spectroscopy is also indicated by more recent data. In the following discussion, the relative value of each of these factors in estimating outcome is evaluated.
An important general question to consider is the spectrum of neurological deficits observed subsequently, and specifically, whether impairment of cognitive functions can occur in the absence of prominent motor deficits (i.e., cerebral palsy) . Large-scale studies show that cognitive impairment without overt cerebral palsy is not uncommon after neonatal HIE. In the largest available series, among survivors of HIE, 9% of children without cerebral palsy had an IQ at 6 to 7 years of less than 70 and fully 31% had scores ranging from 70 to 84. Children with cerebral palsy did have a poorer cognitive outcome (i.e., 96% had an IQ < 70). Overall, 14% of infants treated with hypothermia developed cerebral palsy, versus 28% of those not treated with hypothermia, and the rates of occurrence of cognitive impairment without cerebral palsy were approximately similar in the two treatment groups.
Apgar Scores, Fetal Acidosis, and Neonatal Resuscitation
Because hypoxic-ischemic injury is one cause of depressed Apgar scores , and because depressed Apgar scores imply the possibility of an ongoing hypoxic-ischemic insult, correlation of outcome with such scores has been attempted for many years. This approach is fraught with hazards for several reasons. First, precise quantitation of the Apgar score varies among observers, sometimes considerably. Second, each of the five factors that make up the score is given equal weight, and clearly the importance of each for central nervous system integrity differs greatly. Third, causes of the depressed scores, other than hypoxic-ischemic insult, include laryngeal inhibition (e.g., caused by aspiration of a small amount of amniotic fluid or by oronasopharyngeal–laryngeal stimulation from suction catheters), maternal medications or anesthesia, and prematurity, and are associated with generally favorable prognoses unless additional postnatal insults occur. In a population-based cohort study of 235,165 term infants, of the 292 with a 5-minute Apgar score of 0 to 3, only 16, or 6.8%, later exhibited cerebral palsy. Similarly, in another series of 1200 consecutive deliveries, only 20% of infants with a 5-minute Apgar score of less than 7 had acidosis with a pH of 7.10 or less (umbilical artery). This tenuous relationship between apparent fetal asphyxia and low Apgar scores has been confirmed.
The value of the “extended” Apgar score (i.e., the score after 5 minutes) was demonstrated initially by data from the Collaborative Perinatal Project of the National Institutes of Health and was published over 35 years ago ( Table 20.21 ). The likelihood of cerebral palsy in infants weighing 2500 g or more increased dramatically with the increasing duration of Apgar scores of 3 or less, especially after 15 minutes. Infants with such scores experienced a progressive increase in mortality rate, so that almost 60% of those with Apgar scores of 0 to 3 after 20 minutes subsequently died. Similarly, premature infants also exhibited a distinctly worsening prognosis with low “extended” Apgar scores. It is likely that the major determinant of the poor outcome with longer duration of a low Apgar score in both premature and full-term infants was, in the largest part, the severity of the initial intrauterine insult. However, even though low Apgar scores for as long as 15 minutes are associated with high mortality rates, the majority of survivors escaped major neurological injury (see Table 20.21 ). It is important to note that neurological sequelae less severe than cerebral palsy were not quantitated in this earlier study. Even with the worst of Apgar scores at 1 minute of age (i.e., 0 or apparent stillbirth), in a large series ( n = 93), of the 40% of infants who survived, approximately 60% had a normal outcome. However, of the 58 infants whose Apgar score still was 0 at 10 minutes of age, 57 died, and the sole survivor had an abnormal neurological outcome.
APGAR SCORE OF 0–3 | DEATH IN FIRST YEAR (%) | CEREBRAL PALSY IN SURVIVORS (WITH KNOWN OUTCOME) (%) b |
---|---|---|
1 min | 3 | 1 |
5 min | 8 | 1 |
10 min | 18 | 5 |
15 min | 48 | 9 |
20 min | 59 | 57 |
b Neurological sequelae less pronounced than cerebral palsy were not quantitated.
More recent data in the era of hypothermia treatment suggest value for the 10-minute Apgar score. Thus, in a substudy of 174 infants in the NICHD randomized, controlled trial of therapeutic hypothermia, 64/85 (75%) of those with a 10-minute Apgar score of 0 to 3 had death/disability compared with 40/89 (45%) of those with scores greater than 3. Each point increase in the 10-minute Apgar scores was associated with a significantly lower adjusted risk of death/disability, death, death/IQ < 70, death/cerebral palsy (CP), and disability/IQ < 70 and CP among survivors (all P < 0.05). Among the 24 children with a 10-minute Apgar score of 0, five (21%) survived without disability. The risk-adjusted probabilities of death/disability were significantly lower in cooled infants with Apgar scores of 0 to 3. The authors concluded that although the low 10-minute Apgar scores were associated with poorer school-age outcomes, approximately 20% of infants with a 10-minute Apgar score of 0 survived without disability to school age.
Finally, a novel method of adapting the original Apgar score to provide a combined score that includes elements of respiratory management, chest compressions and the administration of drugs, in addition to the elements of the traditional Apgar score, has been shown to improve the short-term predictive power of this resuscitative evaluation ( Table 20.22 ). Future data may show that this combined score better correlates with outcomes.
Expanded Apgar | |
C | Continuous positive airway pressure a |
O | Oxygen |
M-B | Mask and bag ventilation b |
I | Intubation and ventilation |
N | Neonatal chest compression |
E | Exogenous surfactant |
D | Drugs |
Scoring each item of expanded Apgar: 0 = intervention was performed; 1 = no intervention was performed | |
a : score 0 if “mask and bag” or “intubation and ventilation” is scored 0 b : score 0 if “intubation and ventilation” is scored 0 | |
Traditional Apgar | |
A | Appearance (skin color) 2 = completely pink 1 = centrally pink with acrocyanosis 0 = centrally blue or pale |
P | Pulse (HR) 2 = > 100 beats per minute 1 = < 100 beats per minute 0 = no heart beat |
G | Grimacing (reflex) 2 = Appropriate for gestational age 1 = Reduced for gestational age 0 = No reflex response |
A | Activity (muscle tone) 2 = Appropriate for gestational age 1 = Reduced for gestational age 0 = No reflex response |
R | Respiration (chest movement) 2 = Regular chest movement 1 = Small or irregular chest movement 0 = No chest movement |
The severity of fetal acidosis , as determined by the measurement of umbilical arterial pH and base deficit, is a useful reflection of the severity and duration of intrauterine hypoxia–ischemia. The relationship between the severity of fetal acidosis and neonatal neurological features as well as neurological outcome is reviewed in Chapter 17 .
Certain aspects of the neonatal resuscitation , and particularly the need for positive pressure ventilation and more intensive cardiopulmonary resuscitation efforts (e.g., chest compressions), are predictive of an unfavorable outcome. Hence, as outlined earlier the Combined Apgar score uses this information in addition to the parameters of the traditional Apgar score for prognostication (see Table 20.22 ). In one careful study, when the need for cardiopulmonary resuscitation was associated with evidence of fetal acidemia (pH < 7.00), five of five infants either died in the neonatal period or exhibited neonatal seizures, whereas of 10 infants requiring such resuscitation measures, but without evidence of an appreciable intrauterine insult (cord pH normal), all 10 had a normal outcome. In a later study, the requirement for intubation in full-term infants with severe fetal acidemia (i.e., umbilical arterial pH ≤ 7.0) was associated with a 6.4-fold increase in abnormal neurological outcome. The importance of the duration of delayed onset of breathing, also presumably reflecting the severity of the intrauterine insult, was emphasized by a study of 165 infants who exhibited “postasphyxial encephalopathy.” Thus, the rate of death or subsequent neurological deficits was 42% with delayed onset of breathing for 1 to 9 minutes, 56% for 10 to 19 minutes, and 88% for more than 20 minutes. An additional prognostic feature of the first 30 minutes of life in asphyxiated term infants with severe fetal acidemia (umbilical arterial pH ≤ 7.0) is the occurrence of hypoglycemia. Thus, the 15% of acidemic infants in this study with an initial blood glucose of 40 mg/dL or lower had an 18.5-fold increased risk for death or moderate to severe encephalopathy than those with a glucose concentration higher than 40 mg/dL.
A recent study of more than 300 term infants with apparent intrapartum asphyxia and HIE identified three key variables that together provided a strong prediction of a serious adverse outcome (death or severe neurological sequelae) ( Table 20.23 ). The data suggested that the combination of need for chest compressions for more than 1 minute, a base deficit of 16 or greater, and age at onset of respiration at 30 minutes or greater was associated with a 93% risk of serious adverse outcome. The findings could be useful not only for early prognostication but also perhaps for decision-making concerning neuroprotective therapies. The additions of pH and arterial carbon dioxide pressure (PCO 2 ) of cord blood gas measurements also provide predictive accuracy.
PROBABILITY OF SEVERE OUTCOME a | ||
---|---|---|
VARIABLES | PERCENTAGE OF TOTAL | 95% CONFIDENCE INTERVAL |
None | 46 | 33–58 |
One variable | ||
CC | 69 | NA |
Resp. | 67 | NA |
BD | 6 | NA |
Overall | 64 | 54–73 |
Two variables | ||
CC and Resp. | 67 | NA |
CC and BD | 77 | NA |
BD and Resp. | 81 | NA |
Overall | 77 | 66–85 |
Three variables | ||
CC and Resp. and BD | 93 | 81–99 |
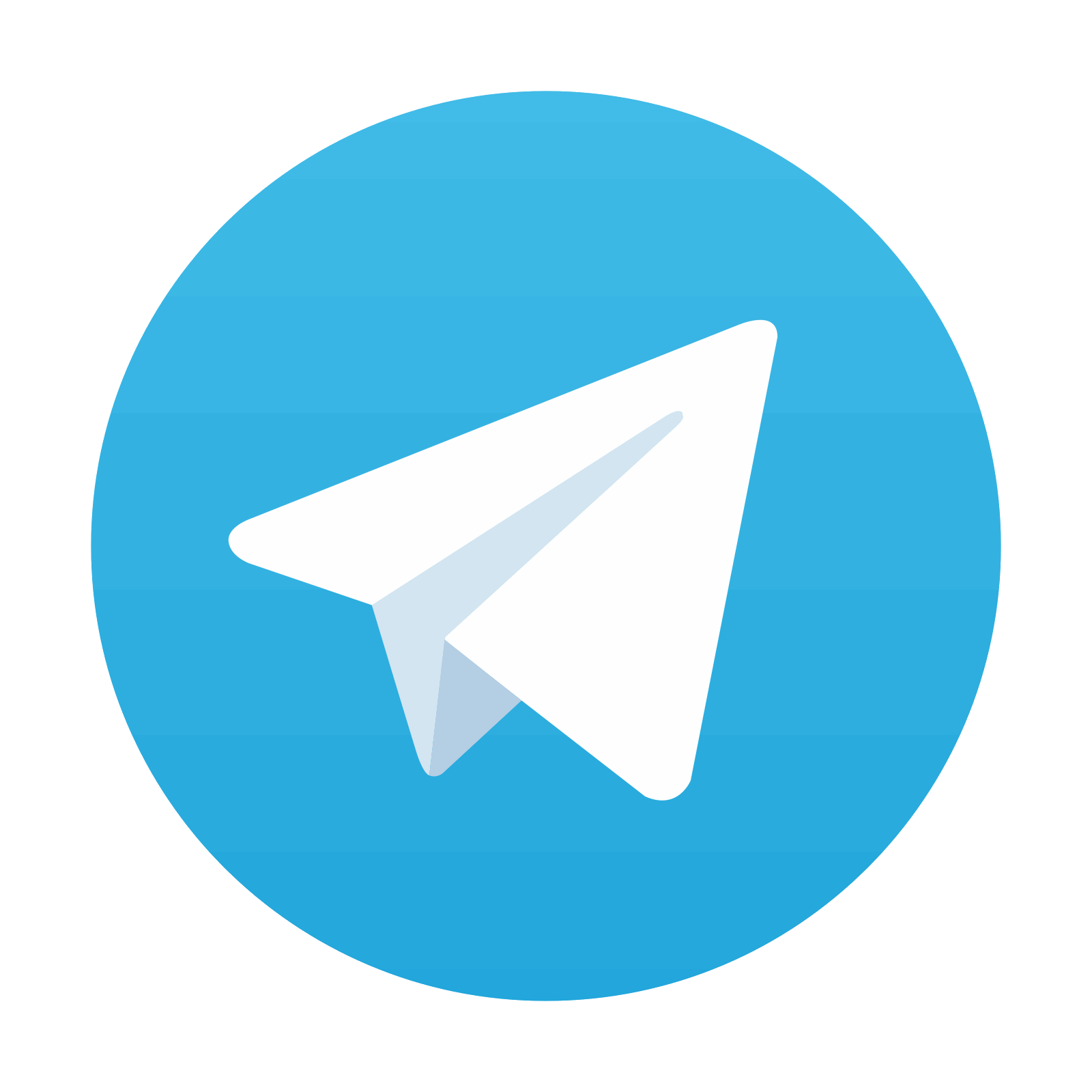
Stay updated, free articles. Join our Telegram channel

Full access? Get Clinical Tree
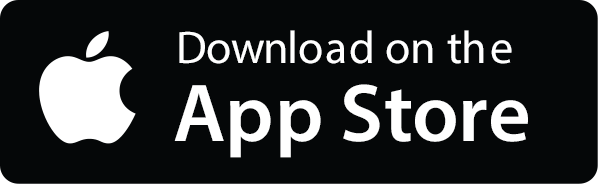
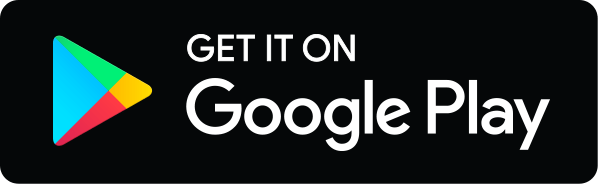
