Abstract
This chapter addresses the pathophysiology of hypoxic-ischemic injury. The neuropathology of this injury includes three principal lesions: selective neuronal necrosis, parasagittal cerebral injury, and cerebral white matter injury. Several recurring pathogenetic themes include initiating factors, principally ischemia, impinging on specific regional anatomical and metabolic/cellular characteristics that underlie a maturation-dependent vulnerability. Ischemia is linked to deleterious perinatal events (see Chapter 17 ) and to impaired cerebrovascular autoregulation. Selective neuronal necrosis, the most common variety of injury, refers to necrosis of neurons in a characteristic distribution (see Chapter 18 ). Three basic patterns are: Diffuse neuronal injury (cerebral cortical, deep nuclear, brain stem) occurs with very severe, relatively prolonged insults; a cerebral cortical–deep nuclear neuronal predominance occurs with moderate to severe, relatively prolonged insults; deep nuclear–brain stem neuronal predominance occurs with severe, relatively abrupt insults. The principal pathogenetic themes are ischemia and regional metabolic and cellular factors. The most important of the latter is the regional distribution of excitatory amino acid receptors. Parasagittal cerebral injury refers to necrosis of neurons and pre-oligodendrocytes in a characteristic distribution, particularly involving superomedial aspects of the cerebral cortex. The principal pathogenetic themes are ischemia, regional vascular factors, and regional cellular/metabolic factors. Regarding vascular and cellular factors, the lesions occur in the distal fields and border zones of major cerebral vessels, and affected neurons and pre-oligodendrocytes exhibit exuberant expression of excitatory amino acid receptors. Cerebral white matter injury is similar, albeit less severe, to the white matter injury of premature infants. The principal pathogenetic themes are ischemia and intrinsic vulnerability of pre-oligodendrocytes (see Chapter 15 ).
Keywords
Ischemia, neurons, pre-oligodendrocytes, regional vascular factors, excitatory amino acid receptors
This chapter addresses the pathophysiology of hypoxic-ischemic injury. The neuropathology of this injury, just discussed in detail in Chapter 18 , includes three principal lesions, that is, selective neuronal necrosis , parasagittal cerebral injury , and cerebral white matter injury . I will discuss each of these lesions separately, because in the infant with hypoxic-ischemic disease one or the other tends to predominate. However, magnetic resonance imaging (MRI) studies of such infants do show considerable overlap in the occurrence of the lesions (see Chapter 18 ). Additionally, although we will discuss pathophysiology for these three categories of injury separately, several pathogenetic themes recur. These themes include initiating factors, principally ischemia, impinging on specific anatomical and metabolic cellular and regional characteristics that underlie a maturation-dependent vulnerability. Ischemia is linked to a variety of deleterious perinatal events (see Chapter 17 ) and to impaired cerebrovascular autoregulation. Regional patterns of ischemic injury occur especially in vascular border zones and end zones. At the cellular level, ischemia leads to cell death, both neuronal and oligodendroglial, via a cascade of events that particularly includes excitotoxicity. The pathophysiological events and the cascade to cell death most often occur over relatively protracted temporal periods and thereby afford opportunities for preventative/ameliorative interventions (see Chapter 20 ).
Selective Neuronal Necrosis
Selective neuronal necrosis is the most common variety of injury observed in neonatal hypoxic-ischemic encephalopathy and refers to necrosis of neurons in a characteristic, although often widespread, distribution (see Chapter 18 ). MRI studies show an overall incidence of approximately 80% in infants with hypoxic-ischemic disease (see Chapter 18 ). Neuronal necrosis often coexists with other distinctive manifestations of neonatal hypoxic-ischemic encephalopathy (see later sections), and in fact it is very unusual to observe one of the other varieties of neonatal hypoxic-ischemic encephalopathy without some degree of selective neuronal injury as well. The topography of the neuronal injury depends in considerable part on the severity and temporal characteristics of the insult and on the gestational age of the infant. Three basic patterns derived primarily from correlative clinical and brain imaging findings, and observed best in term infants, can be distinguished ( Table 19.1 ). Diffuse neuronal injury (cerebral cortical, deep nuclear, brain stem) occurs with very severe, relatively prolonged insults in both term and premature infants; a cerebral cortical–deep nuclear neuronal predominance occurs in primarily term infants with moderate to severe, relatively prolonged insults. The deep nuclear involvement includes basal ganglia (especially putamen) and thalamus. Deep nuclear–brain stem neuronal predominance occurs in primarily term infants with severe, relatively abrupt insults. Two other patterns, pontosubicular neuronal injury and cerebellar injury, are uncommon and will be noted only briefly in the following discussion.
PATTERN a | SEVERITY AND TIMING OF USUAL INSULT b |
---|---|
Diffuse (cerebral cortex, deep nuclear, brain stem) | Severe, prolonged |
Cerebral cortex–deep nuclear b | Moderate, prolonged |
Deep nuclear c –brain stem | Severe, abrupt |
a The patterns reflect areas of predominant neuronal injury; considerable overlap is common.
b Severity and timing of insult are estimates, based on clinical and experimental studies (see text).
c Deep nuclear: basal ganglia (especially putamen) and thalamus.
Pathogenesis
Cerebral Ischemia, Impaired Cerebrovascular Autoregulation, and Pressure-Passive Cerebral Circulation
Cerebral ischemia, with deprivation of oxygen and glucose, followed by reperfusion and the cascade of metabolic events described in Chapter 13 , is the likely pathogenetic sequence in selective neuronal necrosis ( Box 19.1 ). Although the causative relation between cerebral ischemia and both selective neuronal necrosis and parasagittal cerebral injury (see later) has been established in several excellent perinatal animal models (see previous section), studies in human infants also provide excellent support for the role of diminished cerebral blood flow (CBF) secondary to systemic hypotension. Thus, as discussed in detail in Chapter 13 , because of the impaired vascular autoregulation in asphyxiated infants, CBF becomes passively related to arterial blood pressure ( Fig. 19.1 and see Box 19.1 ). The impaired vascular autoregulation has been documented in the hours to days after the insult and, by extrapolation from experimental data (see Chapter 13 ), is presumed to begin during the insult, when hypotension is most pronounced. This situation makes the infant exquisitely vulnerable to the diminutions in blood pressure characteristic of severe asphyxia, and those regions most vulnerable are in the distribution of selective neuronal necrosis as well as the watershed, that is, parasagittal, distributions of the cerebrum (see later). The data of Pryds and co-workers show clearly that those asphyxiated term infants with impaired autoregulation have the poorest neurological outcome (see Fig. 19.1 ).
Cerebral ischemia
Impaired cerebrovascular autoregulation with pressure-passive cerebral circulation
Systemic hypotension
Regional vascular factors
Regional metabolic factors
Regional distribution of excitatory (glutamate) receptors on neurons a
a Single most important factor for determining regional distribution of selective neuronal necrosis.
Factors related to the hypoxic-ischemic insult
Severity and temporal characteristics
Preceding/concomitant infection/inflammation

The causes of the pressure-passive circulation in the asphyxiated newborn could relate to such factors as (1) the hypoxemia or hypercarbia or both of the primary asphyxial insult, (2) the postasphyxial impairment in vascular reactivity observed in experimental models of perinatal asphyxia and presumably related to the effect of one or more of the vasodilatory compounds that accumulate secondary to ischemia-reperfusion (see Chapter 13 ), (3) an “immature” autoregulatory system with limited capacity for reactivity because of the deficient arteriolar muscular lining of penetrating cerebral arteries and arterioles in the third trimester, (4) an autoregulatory system with a lower limit so close to the range of normal blood pressure that even slight hypotension places CBF on the down slope of the curve (see Chapter 15 ), or (5) a combination of these factors. Whatever the mechanisms, the clinical implications are enormous. Falls in arterial blood pressure lead to decreases in CBF and injury to certain vulnerable brain cells , that is, neurons in the distribution of selective neuronal necrosis, and regions , that is, parasagittal cerebrum (see later).
Regional Vascular Factors
The reasons for the selective vulnerability of neuronal groups in the central nervous system have become increasingly clear in recent years. Regional vascular factors certainly can play a role because neuronal injury is more marked in vascular border zones and end zones (see Box 19.1 ). The vulnerable vascular zones include, for depths of cortical sulci, end zones of short penetrating vessels; for basal ganglia and thalamus (including posterior limb of the internal capsule), end zones of lenticulostriate, Heubner and posterior cerebral arteries; and for brain stem, end zones and border zones in the vertebrobasilar system. The predilection of cerebral cortical neuronal injury for parasagittal regions likely relates to their occurrence within the border zones of all three major cerebral vessels (see later).
The role of vascular factors in pathogenesis of pontosubicular necrosis is likely complex. Thus, the documented relationship of the lesion to hypocarbia and to hyperoxemia suggests a role for cerebral vasoconstriction. However, possibly additionally important, the rapid neuronal maturation in the pons and subiculum during the time of occurrence of this lesion suggests that vulnerability of this region may relate in part to the simultaneous occurrence of neuronal differentiation and a propensity of these neurons to undergo apoptosis.
Regional Metabolic Factors
Regional metabolic factors could play a central role (see Box 19.1 ). Those factors that lead to hypoxic cell death in experimental systems (see Chapter 13 ) raise the possibilities of regional differences in anaerobic glycolytic capacity, energy requirements, lactate accumulation, mitochondrial function, calcium influx, nitric oxide synthesis, and free radical formation and scavenging capacity. For example, the high metabolic rate and energy utilization of deep gray matter may contribute to the especial vulnerability of these neurons to the severe, abrupt ischemic insults that lead to particular injury to these neuronal structures (see later). Although relatively little is known about these regional metabolic issues in human brain, recent data suggest a role for impaired antioxidant defenses (see Chapters 14 and 18 ).
Regional Distribution of Excitatory (Glutamate) Receptors
The regional distribution of glutamate receptors, particularly of the N-methyl- d -aspartate (NMDA) and alpha-amino-3-hydroxy-5-methyl-4-isoxazole propionic acid (AMPA) types, now appears to be the single most important determinant of the distribution of selective neuronal injury (see Box 19.1 ). As discussed in detail in Chapter 13 , the topography of hypoxic-ischemic neuronal death in vivo is similar to the topography of glutamate synapses; the particular vulnerability of certain neuronal groups in the perinatal period correlates with a transient, maturation-dependent density of glutamate receptors; extracellular glutamate increases dramatically at such receptors with hypoxia-ischemia; and hypoxic-ischemic neuronal death in vivo can be prevented by administration of blockers of the NMDA receptor-channel complex and, to a considerable extent also, of non-NMDA receptors, especially Ca 2+ -permeable AMPA receptors (see Chapter 13 ). The demonstration that the molecular mechanisms by which activation of the glutamate receptors leads to cell death operate over hours after termination of the insult and that prevention or amelioration of such excitotoxic injury can be effected by glutamate receptor blockers administered also after termination of the insult has profound and obvious clinical implications (see Chapters 13 and 20 ).
The importance of the regional distribution of glutamate receptors in the determination of regional selectivity of neuronal injury is particularly apparent in basal ganglia and thalamus. Thus, first, it is clear that there is a transient, dense glutamatergic innervation of the basal ganglia and thalamus in the perinatal period, both in experimental models (see Chapter 13 ) and in the human. Second, the development of vulnerability of striatum and thalamus to hypoxic-ischemic injury parallels the expression of glutamate receptors and the vulnerability to direct injections of glutamate (see Chapter 13 ). Third, extracellular glutamate levels have been shown to rise in perinatal models of hypoxic-ischemic striatal injury (see Chapter 13 ). Fourth, a highly effective blocker of perinatal hypoxic-ischemic deep nuclear is MK-801, a specific blocker of the NMDA receptor-channel complex (see Chapter 13 ). Fifth, there is a specific hierarchy of potency of glutamate receptor agonists for production of striatal injury, and this potency parallels the expression of receptor subtypes and the inhibitory capabilities of specific receptor antagonists (see Chapter 13 ).
As noted earlier, neuronal injury in brain stem often accompanies such injury in basal ganglia and thalamus. Notably, studies of the developmental profiles of glutamate receptor subtype binding in the human brain stem have shown transient elevations in inferior olive and basis pontis of NMDA and kainate receptors in early infancy. The likely importance of the development of glutamate receptor expression in vulnerable brain stem nuclei is also discussed in Chapter 18 .
Perhaps related to the role of glutamate receptors of the NMDA type in pathogenesis of selective striatal neuronal injury is a relative sparing of nicotinamide adenine dinucleotide phosphate (NADPH)–diaphorase neurons in hypoxia-ischemia. NADPH diaphorase has been shown to be identical to nitric oxide synthase, and generation of nitric oxide has been shown to be one mechanism whereby activation of NMDA receptors (which contact NADPH-diaphorase neurons) leads to striatal neuronal death (see also Chapter 13 ). Since nitric oxide synthase is activated by Ca 2+ , and Ca 2+ influx follows activation of the NMDA receptor (see Chapter 13 ), the data suggest a sequence of NMDA receptor activation, activation of nitric oxide synthase, generation of nitric oxide, and diffusion of nitric oxide (a highly reactive molecule that can generate free radicals) to adjacent neurons, and free radical–mediated cell death. It is noteworthy that the peak period of vulnerability of striatal neurons in the immature rat corresponds to the peak periods of sparing of NADPH-diaphorase neurons and of hypoxic-ischemic vulnerability. The reason for the relative sparing of NADPH-diaphorase neurons remains unclear, but this sparing may contribute importantly to perinatal striatal neuronal death. Currently, it is not known whether a similar sparing of nitric oxide–synthesizing neurons contributes to selective neuronal injury in cerebral cortical and other areas vulnerable in hypoxia-ischemia in the neonatal human. However, potential importance for neuronal nitric oxide synthase in mediation of such hypoxic-ischemic neuronal injury is suggested by the results of a study of the development of neuronal expression of the enzyme in human brain. The striking findings were a higher density of nitric oxide synthase–positive neurons in late fetal human brain than in adult brain and a concentration of such neurons in areas known to be injured in selective neuronal necrosis, that is, deeper layers of cerebral cortex, striatum, and brain stem tegmentum.
Recent studies of glutamate receptors in developing human cerebral cortex suggest that a transient expression of Ca 2+ -permeable AMPA receptors and NMDA receptors occurs around the time of term birth ( Fig. 19.2 ). Thus, the theme is similar to that for basal ganglia and brain stem, that is, a maturation-dependent exuberant expression of Ca 2+ -permeable glutamate receptors becomes lethal to neurons with excessive activation, as occurs with cerebral ischemia.

Factors Related to the Hypoxic-Ischemic Insult
Severity and Temporal Characteristics.
Factors related to the severity and the temporal characteristics of the insult appear to be of particular importance in determining the major pattern of selective neuronal injury in the newborn (see Table 19.1 ). Severe and prolonged insults result in diffuse and marked neuronal necrosis, involving the many levels of the neuraxis described earlier as the diffuse pattern of injury. The cerebral–deep nuclear pattern of neuronal injury appears to be related to insults that are less severe and prolonged, often termed partial, prolonged asphyxia . The deep nuclear–brain stem pattern of injury to basal ganglia–thalamus–brain stem has been described in human infants with a severe, abrupt event, often termed total asphyxia . It is postulated that the severe, abrupt event prevents the operation of major adaptive mechanisms normally operative with asphyxial events (see Chapter 13 ). The most important of these may be the diversion of blood from the cerebral hemispheres to the vital deep nuclear structures. Because the latter have high rates of energy utilization and also a high content of glutamate receptors, these nuclei are particularly likely to be injured. In the more prolonged and less severe insults, the diversion of blood to deep nuclear structures occurs at least to a degree, and thus the cerebral regions are more likely to be affected. Studies in the near-term fetal lamb indicate that the severe terminal insult that results in injury to deep nuclear structures especially may be likely to occur after brief, repeated hypoxic-ischemic insults first cause a cumulative deleterious effect on cardiovascular function that presumably then can result in a severe late insult.
Difficulty of Determining Timing and Duration of Insult(s).
In the clinical setting available methods are not ideal for determining timing and duration of hypoxic-ischemic insults. As delineated in Chapter 17 , the large majority of insults occur in the late intrauterine/intrapartum period. In the living infant MRI studies can be valuable in delineating approximate timing, albeit not with extreme accuracy (see Chapter 20 ).
Classic studies with term fetal monkeys by Myers and co-workers provide insight into the relation of timing and duration of hypoxic-ischemic insults to certain patterns of injury. For example, the deep nuclear-brain stem pattern was produced by total asphyxia in the term fetal monkey. The sequence of events was rapidly progressive fetal hypoxia, hypercarbia, acidosis, hypotension, and brain injury within approximately 10 to 15 minutes. The topography of the neuropathology is markedly similar to that in the human fetus subjected to apparent total asphyxia, for example, with cord prolapse or uterine rupture. In one carefully documented human study, this neuropathology was identified by neuroimaging after such sentinel events occurring generally from 10 to 46 minutes before delivery.
Term fetal monkeys subjected to a partial rather than total interference with respiratory gas exchange (produced by halothane-induced hypotension) developed physiological derangements over longer periods), so-called prolonged partial asphyxia. The maternal event resulted in more slowly evolving hypoxia and acidosis, followed by late decelerations of the fetal heart rate, diminished cardiac output, hypotension, and evidence for cerebral ischemia. Brain injury became apparent after several hours, and the topography involved cerebral cortex (especially in paracentral areas), basal ganglia, and thalamus, as in human infants (see Table 19.1 ) If the insults were especially severe and prolonged, brain stem was also involved, as in the diffuse pattern noted in Table 19.1 .
As noted earlier, determination of timing and severity of insult in the perinatal clinical setting is hindered by the lack of available methods, whether they be real-time fetal monitoring or retrospective estimates by various magnetic resonance determinations. Indeed, in the 80% to 90% of cases of infants with hypoxic-ischemic disease, in which an overt fetal sentinel event is not present, the uncertainty in timing is often measured in hours, often many hours or more, and not minutes. These issues are discussed in detail in Chapter 17 .
Preceding or Concomitant Infection/Inflammation.
As discussed in Chapter 13 , experimental data demonstrate the potentiation of hypoxic-ischemic insults by preceding or concomitant infection/inflammation . Thus, hypoxic-ischemic insults not severe enough to cause injury alone can be rendered seriously injurious if the fetus or infant is exposed to inflammatory factors associated with intrauterine or postnatal infection. This phenomenon could underlie, at least in part, the accentuated risk of apparent hypoxic-ischemic brain injury observed in infants who sustain their insults in association with chorioamnionitis and/or who have elevated levels of cytokines in blood or cerebrospinal fluid. However, it should be emphasized that the vast majority of term infants exposed to chorioamnionitis have an uncomplicated neonatal course and neurologic outcome, and overall, histologic chorioamnionitis does not appear to increase the risk of adverse outcome even in infants with hypoxic-ischemic encephalopathy. Nevertheless, in the subset of infants with histologic chorioamnionitis and evidence of a fetal placental response, for example, villitis, adverse outcome is increased. The presence or absence of a fetal inflammatory response may be most critical, perhaps both by local placental vascular obliterative/blood flow effects and by potentiation of hypoxic-ischemic effects, as described in Chapter 13 .
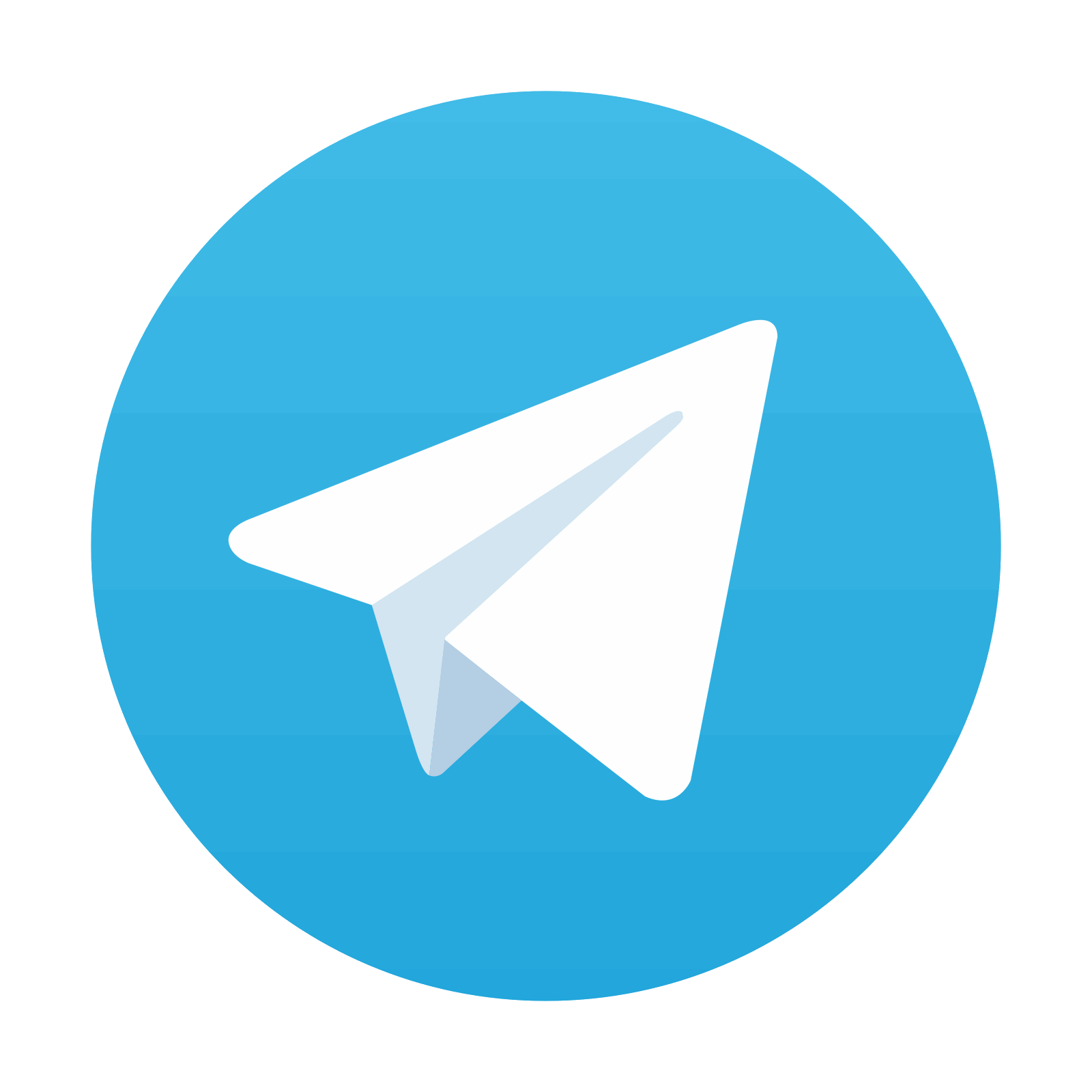
Stay updated, free articles. Join our Telegram channel

Full access? Get Clinical Tree
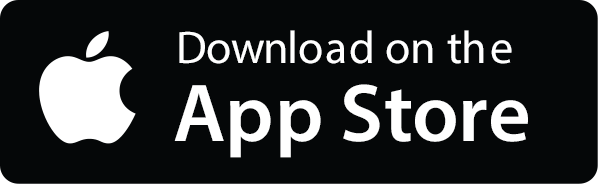
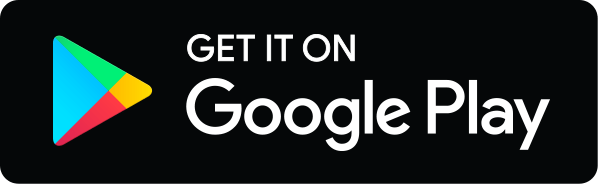