Chapter 7 Luzius A. Steiner Department of Anesthesiology, University Hospital of Basel, Switzerland Injury resulting from traumatic insults to the brain is typically divided into primary and secondary injury. Primary injury occurs at the moment of the trauma and currently cannot be influenced in the clinical setting. In contrast, secondary brain injury occurs at some time after the primary impact as a complication of primary injury and is potentially preventable and treatable. Secondary insults are classified as either intracranial or extracranial (Table 7.1) and have a major impact on outcome. The goal of neuromonitoring and neurological intensive care treatment in patients with traumatic brain injury (TBI) is to prevent, or if that is not possible, to rapidly recognize and treat secondary insults. Table 7.1 Secondary brain insults following TBI. Clinical deterioration is often the first sign of a secondary insult such as a rise in intracranial pressure (ICP) or developing intracerebral hematoma. This underlines the importance of repeated standardized neurological assessments to detect such a clinical deterioration as early as possible. Standardized scoring systems facilitate quantitative reporting of the neurological status and are indispensable if the neurological status needs to be compared to earlier assessments. The most widely used score is the Glasgow Coma Scale (GCS).Of the three components of the GCS, the motor score is considered to be the most important. Recently, a new coma scale has been introduced: the Full Outline of UnResponsiveness (FOUR score) [1]. It addresses some of the shortcomings of the GCS by including brainstem reflexes and respiration, allowing detection of subtle neurological changes and, thus, further classification of deeply comatose patients. A comparison between the GCS and the FOUR score is shown in Table 7.2. While such scores are rapidly administered and have a high interrater reliability, they cannot replace frequent in-depth neurological examinations. Table 7.2 GCS and FOUR score [1]: A comparison. In contrast to most other organs the brain is protected by a stiff skull. A rise in ICP may therefore impede cerebral blood flow (CBF) and may cause ischemia. Elevated ICP is an important secondary insult and a predictor of poor outcome after TBI. Possible causes of raised ICP are intracranial mass lesions, disorders of cerebrospinal fluid (CSF) circulation, or brain edema. ICP is an important treatment endpoint and also used to calculate cerebral perfusion pressure (CPP), defined as the difference between mean arterial pressure (MAP) and ICP (CPP = MAP – ICP). CPP represents the pressure gradient across the cerebral vascular bed and is used as a therapeutic target for patients with TBI in most intensive care units (ICUs). The gold standard for assessing ICP is an intraventricular drain inserted into one of the lateral ventricles and connected to an external pressure transducer [2]. The foramen of Monro or for clinical purposes the external auditory meatus is the reference point for zeroing the transducer. As patients are maintained in a 20–30° head-up position, the zero for arterial pressure should be set at the same height as for ICP to calculate CPP correctly. In addition to monitoring pressure, intraventricular catheters allow withdrawal of CSF to treat raised ICP. The main drawback of intraventricular catheters is the risk of infection that increases over time and may reach 20%. In many units CSF samples are analyzed on a daily basis to detect infection, although daily sampling itself may increase risk of infection. Moreover, the insertion of ventricular catheters may be difficult in patients with severe brain swelling. As an alternative, intraparenchymal probes are used. The infection rate of these probes is very low. An example of such a device is shown in Figure 7.1. The accuracy of subarachnoid, subdural, and epidural devices is lower than that of intraventricular or intraparenchymal sensors, and they are rarely used in patients with TBI. Pressure measured in the lumbar CSF space is not a reliable estimator of ICP in brain-injured patients, and such measurements may be dangerous in patients with space-occupying lesions. ICP cannot be assumed to be evenly distributed in many pathological states and it is important to realize that with a ventricular catheter, uniformly distributed ICP will be observed only when CSF circulates freely between all its natural pools. Significant pressure gradients may exist in patients with intracranial hypertension, and an intraparenchymal probe measures only local pressure, which can be compartmentalized and is not necessarily identical with intraventricular pressure. Bilateral parenchymal ICP monitoring has demonstrated large ICP gradients between the two hemispheres. Figure 7.1 Intraparenchymal ICP monitoring device. 1a, Reference pressure, 1b, measured pressure; 1, reference pressure duct, chip with membrane; 3, catheter attachment; 4, polyurethane catheter; 5, titanium case; 6, connecting wires; 2a, pressure-sensitive resistor; 2b, membrane; 2c, pressure chip (Reproduced with permission from RAUMEDIC AG, Helmbrechts, Germany. ©RAUMEDIC AG). Despite the broadly felt need of demonstrating the benefit of ICP monitoring, until recently, no randomized controlled trial was performed. In 2012, the BEST TRIP trial was published [3]. BEST TRIP is an acronym for Benchmark Evidence from South American Trials: Treatment of Intracranial Pressure.This study in Bolivian and Ecuadorian centers in collaboration with US neurosurgeons was designed to determine whether information derived from the monitoring of ICP in patients with severe TBI improves medical practice and patient outcomes. It was a multicenter, parallel-group trial randomizing patients with severe TBI for ICP monitoring or imaging and clinical examination. Randomization was stratified according to study site, injury severity, and age. The participating hospitals had ICUs that were staffed with intensivists, 24 h computed tomographic (CT) services, and neurosurgery coverage. The inclusion criteria were 13 years of age or older and GCS = 3–8 (with a score on the GCS motor component 1–5 if the patient was intubated) or a higher GCS score dropping to 3–8 within 48 h. In the ICP monitoring group the treatment focused on keeping ICP below 20 mmHg. A strength of this study was that outcome was determined at several time points and on various (as opposed to single) outcome domains. At the time of hospital discharge, measures of survival, duration, and level of impaired consciousness and orientation were obtained. At 3 months, functional status and orientation (GOS-E, the Disability Rating Scale, and GOAT) were measured. And at 6 months, a functional and neuropsychological battery of tests was administered including mental status, working memory, information-processing speed, episodic memory and learning, verbal fluency, executive function, and motor dexterity. A further asset of this study was that trained examiners unaware of the group assignments administered the tests at 3 and 6 months [3]. The results of the study demonstrated no significant differences in the 14-day mortality (30% in the imaging–clinical examination group vs. 21% in the pressure-monitoring group; hazard ratio, 1.36; 95% [CI], 0.87–2.11; P = 0.18) or in the 6-month mortality (41% in the imaging–clinical examination group vs. 39% in the pressure-monitoring group, hazard ratio, 1.10; 95% CI, 0.77 to 1.57; P = 0.60). Hence, the results of this long-awaited and important study do not support the superiority of management guided by ICP monitoring over management guided by neurologic examination and serial CT imaging in patients with severe TBI. Of interest further is that the length of stay in the ICU was similar in the two groups. Importantly, the same treatment options were available to both groups, but the imaging–clinical examination group compared to the pressure-monitoring group received hyperosmolar fluids and hyperventilation during more days (4.8 vs. 3.4, P = 0.002). The distribution of serious adverse events was similar in the two groups [3]. However, this trial should not be used to justify abandoning ICP monitoring. The first author of this important paper points out: “The strongest clinical implication of the BEST TRIP trial is that we need to refine the role of ICP monitoring in sTBI management. Given its established ease and safety, we do not feel that diminished ICP monitoring should follow this report. Instead, clinical methods for interpreting ICP in the setting of individual patients must be developed [4].” An observational study has even suggested that a CPP-/ICP-oriented therapy will increase treatment intensity and respirator days without an improvement in outcome [5]. Nevertheless, based on the available evidence the guidelines of the Brain Trauma Foundation conclude that ICP data are useful for guiding therapy [6]. These guidelines are accessible on the Internet (www.braintrauma.org). ICP monitoring is recommended in salvageable patients with a GCS of 3–8 and an abnormal CT defined as a scan showing hematomas, contusions, swelling, herniation, or compressed basal cisterns. ICP monitoring is also recommended in patients with a GCS of 3–8 and a normal CT scan provided at least two of the following criteria are fulfilled at admission: age greater than 40 years, unilateral or bilateral motor posturing or systolic blood pressure less than 90 mmHg [6]. Some clinicians will choose to adapt these recommendations and, for example, monitor patients with a GCS greater than 8 if they will undergo major noncranial surgery soon after admission. Rapid identification of episodes of low or high CBF would in principle allow timely intervention and possibly result in improved outcome. Methods for quantitative determination of CBF at the bedside based on measurements from the internal jugular vein have been developed but are rarely used [7, 8]. An intraparenchymal probe using thermal diffusion providing continuous quantitative real-time data that are in good agreement with values obtained by xenon CT for a volume of approximately 5 cm3 around the tip of the probe has been used in brain-injured patients [9]. Transcranial Doppler is simple and noninvasive, and can be used repeatedly. Transcranial Doppler measures blood flow velocity in the basal cerebral arteries, not CBF, and the linear relationship between CBF and mean flow velocity is only present if neither the diameter of the insonated vessel nor the angle of insonation changes during the examination. This assumption is probably fulfilled for most situations where examinations of the basal cerebral arteries are performed. Transcranial Doppler is the simplest way to noninvasively obtain repeated real-time estimates of CBF. The clinical usefulness of transcranial Doppler lies mostly in its ability to quantify cerebrovascular pressure autoregulation and CO2-reactivity, both of which have been linked to outcome. The ability of all the aforementioned methods to detect episodes of critically low or excessive CBF is limited for technical reasons but also because the thresholds of ischemia and hyperemia are variable after head injury (Figure 7.2). Furthermore, manipulating CBF is difficult, and adjusting CPP or the arterial partial pressure of CO2 (PaCO2) does not necessarily have the desired effect. There is no convincing evidence that a strategy that attempts to manipulate CBF is superior to an approach using ICP or CPP to guide treatment. CBF monitoring is an option with unclear clinical benefit at the present time [10]. Figure 7.2 Diagram illustrating the relationship between cerebral blood flow (CBF), cerebral metabolic rate of oxygen (CMRO2), and oxygen extraction fraction (OEF). Under physiological conditions, CBF and CMRO2 are tightly coupled and OEF remains more or less stable. In TBI, an uncoupling of CBF and CMRO2 may occur. With low CBF (lower half of diagram), CMRO2 can remain coupled or decrease or increase. Accordingly, with low CBF, hyperemia, coupled with hypometabolism, or ischemia is possible. CBF values do not allow discriminating between these three states. However, OEF will rise or fall in response to the changing relationship between CBF and CMRO2. With increased CBF, the same three scenarios are possible (Courtesy of Prof. D. Menon, Department of Anaesthesia, University of Cambridge, Cambridge, UK. Reproduced with permission). Due to the difficulties associated with CBF monitoring and treatment thresholds, the focus of interest has shifted to monitoring cerebral oxygenation. Ideally, changes in oxygen extraction fraction (OEF) should be monitored. Such a device is not readily available, but surrogate markers can be monitored. The placement of a catheter in the jugular bulb allows sampling of blood that almost exclusively drains from the intracranial circulation. Jugular bulb saturation (SJO2) is measured either from blood that is sampled intermittently from such a catheter or continuously by a fiber-optic catheter. The arteriojugular oxygen content difference (AJDO2) is calculated as the difference between the arterial and jugular oxygen content in paired blood samples. Normal values for SJO2 range from 50% to 75%, and normal values for AJDO2 from 4 mL·100 mL−1to 9 mL·100 mL−1
ICU care: surgical and medical management—neurological monitoring and treatment
Intracranial insults
Systemic insults
Intracranial hypertension
Hypoxia
Space-occupying lesion
Hypotension
Brain edema
Hypocapnia–hypercapnia
Seizures
Hypoglycemia–hyperglycemia
Vasospasm
Hyponatremia
Hydrocephalus
Hypo-osmolarity–hyperosmolarity
Cerebral infection
Hyperthermia
Anemia
Sepsis
Neuromonitoring
Clinical neuromonitoring
FOUR score
GCS
Eye response
4
Eyelids open/opened, tracking, or blink to command
4
Spontaneous eye opening
3
Eyelids open but not tracking
—
—
2
Eyelids closed but open to loud voice
3
Eye opening to speech
1
Eyelids closed but open to pain
2
Eye opening to pain
0
Eyelids remain closed with pain
1
No reaction to pain
Motor response
4
Thumbs-up, fist, or peace sign
6
Obeying commands
3
Localization to pain
5
Localization to pain
2
Flexion response to pain
4
Normal flexion to pain
—
—
3
Abnormal flexion to pain
1
Extension response to pain
2
Extension to pain
0
No response to pain or generalized myoclonus status
1
No response to pain
Verbal response
Not assessed
5
Oriented
4
Confused conversation
3
Inappropriate words
2
Incomprehensible sounds
1
No response
Brainstem reflexes
4
Pupil and corneal reflexes present
Not assessed
3
One pupil wide and fixed
2
Pupil or corneal reflexes absent
1
Pupil and corneal reflexes absent
0
Absent pupil, corneal, and cough reflex
Respiration
4
Not intubated, regular breathing pattern
Not assessed
3
Not intubated, Cheyne–Stokes breathing pattern
2
Not intubated, irregular breathing
1
Breathes above ventilator rate
0
Breathes at ventilator rate or apnea
Intracranial pressure and cerebral perfusion pressure monitoring
CBF monitors
Cerebral oxygenation monitoring
Jugular bulb oxymetry
Stay updated, free articles. Join our Telegram channel

Full access? Get Clinical Tree
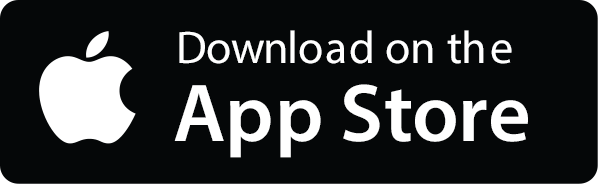
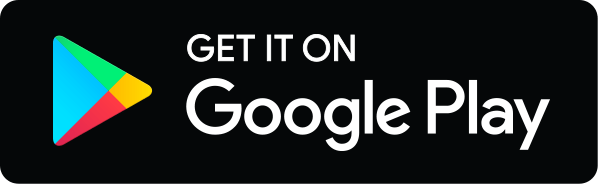