1 Image Guidance and Epilepsy Surgery Navigational systems have gained widespread acceptance in the neurosurgical community over the past decade. There is hardly a contemporary neurosurgery center that does not have one or several image-guided surgery (IGS) systems in the operating room. However, the utilization of these systems still does vary from institution to institution and from one surgeon to the next.1 For the most part, epilepsy surgeons embraced computer-guidance technology early on because stereotaxis already was an integral component of techniques needed and employed by most epilepsy surgeons.2–6 Although stereotactic frames were used in animals in the 19th century and early prototypes for human use have been reported,7, 8 the first use in humans is generally attributed to Spiegel and Wycis initially in the context of treating psychiatric disorders, then for pain and movement disorder surgery.9 The application of frames for the stereotactic placement of depth electrodes to study epilepsy was reported in the 1950s by Bancaud and Talairach.10 At the time, centers already performing surgery for epilepsy on the basis of scalp and intraoperative electroencephalogram (EEG) recordings, seizure semiology, and to a limited degree imaging studies (pneumoencephalography, angiography) gradually adopted stereotactic methodology for the investigation of subsets of patients. Renewed interest in epilepsy surgery in the 1980s, along with the advent of computed tomography (CT)-based and magnetic resonance imaging (MRI)-based stereotaxis, led to more extensive use of frame-based stereotaxis for invasive EEG studies.11–13 Discussion of the enormous impact of computer-based neuroimaging modalities of CT and especially MRI on epilepsy surgery is beyond the scope of this chapter. With regard to stereotaxis, these imaging modalities were used to supplement or replace atlas-based strategies that relied on ventriculography, stereotactic angiography, or both. Stereotactic angiography is still employed in some centers as an adjunct to avoid vascular injury during electrode placement and possibly to increase accuracy.12 The improvement of CT and MRI, the possibility of acquiring “volumetric” imaging datasets, and the increase of computer power used to correlate these datasets to stereotactic space paved the way for image-guided technology. Patrick Kelly was one of the pioneers in the correlating of three-dimensonally (3-D)-defined image datasets with surgical stereotactic space as defined by a stereotactic frame.14–16 When image datasets are acquired as a volume, the head can be reconstructed in 3-D in image space and the surfaces can be “rendered.” In this manner the surface of the skin and (when using CT) the skull can be used to reference the location of targets in the brain instead of a stereotactic frame. Thus the concept of frameless stereotaxis was born.17–21 To match target points of the image dataset with surgical targets in the operating room, the surface of the imaging-rendered volume must be correlated point for point with the surface of the patient’s head at surgery. This process is known as registration and is of considerable importance with regard to the ensuing accuracy and reliability of image guidance at surgery.22 Registration is dependant on a tracking system that can determine the position of points in space with regard to a fixed reference at the time of surgery. The earliest forms of frameless registration used ultrasound or mechanical arms for tracking, and these were applied to epilepsy surgery.3,6,23 Most currently available image-guidance systems have moved to optical tracking where the position of a pointer and reference are triangulated by pole-mounted cameras, or to magnetic tracking where the head and instrument positions are defined within the reference of a generated magnetic field.1,24–26 It cannot be overstressed that technology cannot be a substitute for anatomical knowledge or medical and surgical knowledge and experience. Any surgeon undertaking surgery for epilepsy should have the appropriate medical and surgical background and training. Knowledge of neuroanatomy and concepts of functional neuroanatomy and localization of function are more critical to epilepsy surgery than any other area of neurosurgery. Within these parameters, the author has found image guidance to be a remarkable adjunctive tool in epilepsy surgery strategies. Although epilepsy surgeons should be able to plan and perform surgery without reliance on image-guidance technology, the author hopes to highlight the multifaceted usefulness of imageguidance technology in the contemporary approach to surgery for epilepsy. Image guidance is not restricted to use in the operating theater. For general neurosurgery, the planning of cranial procedures can be instructional in assessing a variety of approaches and surgery strategies on a virtual basis using the IGS planning station. This preoperative role can be greatly expanded in the epilepsy context. Because of the overwhelming importance of a variety of imaging modalities to the contemporary investigation of epilepsy, an IGS workstation can be used as a tool to bring these imaging modalities together for analysis and discussion, even in patients that end up not being candidates for surgery. Many of the software options incorporated into current generation IGS systems can be used to great effect for image analysis and correlation in epilepsy, quite independently of surgical guidance issues. Although similar analysis can be performed on imaging workstations not related to IGS, not all medical centers have all of these options at their disposal. More important, image processing and quantification can be quite labor-intensive and dedicated personnel may not be available. A neurosurgeon familiar with the more sophisticated aspects of IGS workstations can contribute valuable analysis options to a multidisciplinary team involved in epilepsy surgery workups. With the advent of MRI, previously recognized epilepsy-related physiopathological entities such as mesiotemporal sclerosis (MTS) became visible on preoperative imaging studies.27,28 This has simplified surgical decision making for clearly visualized MTS with congruent electrophysiological and neuropsychological findings.29 On the other hand, when imaging findings have been less clear-cut in both temporal and extratemporal epilepsy, many epilepsy centers have resorted to the quantification of structure volumes on MRI with the hope of gaining additional localizing information in epilepsy investigations.30,31 This can be performed on volumetrically acquired MRI data on an imaging workstation, and more recently on a regular PC or laptop with the installation of the appropriate software. In several different centers, the author has taken advantage of the fact that MRI volumetric acquisition appropriate for epilepsy-imaging protocols was also appropriate for subsequent image guidance at surgery. In this manner, imaging protocols can be designed for both analysis and surgery, a cost-effective strategy to avoid repeat imaging. With this dual role of imaging in mind, Table 1.1 lists the author’s basic, routine imaging sequences for epilepsy/epilepsy surgery with discussion of add-on sequences for specific indications. There is a wealth of literature on volumetric studies that quantify MRI volumes of hippocampus, amygdala, entorhinal cortex, other cortical areas, total temporal, frontal lobe or whole brain, thalamic nucleus, etc.32–35 The motivation behind these strategies and the anatomical knowledge of the structural boundaries involved are beyond the scope of this chapter. The following brief discussion will be restricted to practical consideration for using an IGS workstation to perform volume analysis. This is predicated on the fact that most IGS systems will give a readout of volume estimation of any model that is built on the workstation. For research purposes, absolute volumes as given by IGS workstations need to be validated against other volumetric measurement strategies such as non-IGS workstation segmentation and stereology.36 Most of the author’s experience in volumetric assessment using an IGS workstation has been with the StealthStation (Medtronic IGN, Louisville, CO). Each IGS manufacturer limits the number of volumes that can be defined. On most recent systems, whole brain volume can be segmented relatively automatically, and an appropriate menu will provide the volume readout for this. For other structures, such as the hippocampus, the author has relied on mainly manual segmentation (Fig. 1.1). This means that the structure of interest must be manually outlined on a sequential slice-by-slice basis, usually on coronal planes at higher magnification. It is usually useful in correctly identifying the structure of interest to set the screen view to simultaneously show axial and sagittal reformats. It is important to define the outlines of the structure of interest in only one plane as you progress through the volume. Once the volume has been defined on the last slice of the MRI, it is crucial to save the volume because acquisition is quite time-consuming and if one fails a “save” instruction, everything will be lost. As soon as the volume is saved, a readout of the measured volume becomes available on most systems. Because IGS workstations are designed for general neurosurgeons, not epilepsy surgeons, right and left hippocampal volumes may have to be designated as “skull” and “ventricles,” respectively (for example). Color coding of volumes is usually available to help clarify matters somewhat. In the overall investigation of possibly surgically treatable epilepsy, the correlation of different imaging sequences and modalities is very useful. Here again, most contemporary IGS systems incorporate algorithms for image correlation or “fusion.” Of available “routine” MRI sequences, the author has used fluid-attenuated inversion recovery (FLAIR) sequences the most frequently to help define areas of potential cortical dysplasia, gliosis, or low-grade tumor.37 It is helpful to acquire any image sequence intended for routine importation into an image-guidance system in as “volumetric” an acquisition as possible (i.e., thin sequential cuts with no gap). Current “image fusion” software in IGS systems will compensate for suboptimally acquired secondary imaging datasets to some degree. This will be at the expense of image quality in the reformatted planes. Most IGS systems now have software that “automatically” performs image fusion between different datasets based on mutual shared information algorithms. This works very well in most cases. Manual fusion is still available when automatic fusion seems suboptimal. From a practical standpoint, manual image correlation is performed by selecting matching anatomical points on the two sequences to be correlated.22 If fiducial markers (either adhesive or skull-implanted) are present, they can be used for this. Otherwise, the author recommends the pituitary stalk, optic chiasm, anterior and posterior commisures, and vascular bifurcations (carotids, basilar tip, vertebrobasilar junction, some cortical veins). The same landmarks can be examined to assess the reliability of an automatically performed image fusion. Newer anatomical MR imaging sequences of interest in epilepsy include diffusion tensor imaging (DTI)38,39 and higher field MRI inversion recovery sequences. In most cases, these image sequences need to be correlated or “fused” to a primary volumetric dataset for 3-D localization and image-guidance purposes. In addition to the correlation of different MRI anatomical imaging sequences, the co-registration of other non-MRI modalities can be facilitated by IGS station software. This includes source localization from EEG, magnetoencephalography (MEG), positron emission tomography (PET), and ictal single photon emission tomography (SPECT) to name just a few (Fig. 1.2).25,40–43 The main goal is to provide 3-D-defined brain localization for functional or metabolic data. This can then be presented at multidisciplinary meetings for discussion and interpretation (Fig. 1.3). Fig. 1.1 This is an illustration of a 3-D model built on an IGS workstation (StealthStation, Medronic, Colorado). The right and left amygdalar (red) and hippocampal (models) were segmented slice by slice on coronal images. The individual models give a volume readout when built. From a practical standpoint, it is important to save the individual models frequently as they are being built to not lose the work in progress. Functional imaging modalities can involve activation studies in functional MRI (fMRI) or in some of the modalities mentioned above (PET, MEG, magnetic resonance spectroscopic imaging [MRSI], EEG, etc.). As previously mentioned, it is useful to attempt localization of the modality being tested to a cortical area, if possible. In this manner it can be possible to compare localization of function to that of epileptic activity and anatomical landmarks. Frequently, cortical vein modeling provides useful landmarks to help localize target areas in the brain (Figs. 1.2 and 1.3). The overall goal of epilepsy investigations in view of possible surgery is the same as that when considering any medical treatment: an assessment of the risks of a particular procedure compared with the likelihood and degree of benefit. In this context, an IGS workstation used to correlate and present multiple investigations can be a powerful tool. Discussing possible vascular risks, functional risks, and surgical challenges is best done when looking at a workstation multimodal display. In patients who are thought to be suitable candidates for epilepsy surgery, image guidance can be helpful for actual neuronavigation. If a variety of imaging modalities have already been correlated using the image-guidance workstation, these datasets can be used during surgery with relatively little further refinement. It remains essential, however, to highlight that for most common surgical procedures for epilepsy, surgeons should have an intimate knowledge of neuroanatomy, the literature regarding functional localization, and the neuropsychological risks related to resective or disconnective procedure variants. As with any technology, neuronavigation should be an adjunct to refine treatment, as opposed to a crutch. In more demanding situations that require a heavier reliance on image guidance, it becomes essential to be aware of how reliable and accurate this technology may be during surgery. Most contemporary imaging protocols that have evolved for use with image guidance have been streamlined to introduce relatively minimal error into the procedure. There remain, however, many other sources of localization error of neuronavigation during surgery. The final error of localizing targets during surgery is the cumulative result of all the possible sources of error when correlating targets on the IGS workstation (in “image space”) with corresponding targets at surgery (in “surgical space”). The term application accuracy has been introduced to refer to this cumulative error.22,44–46 Application accuracy will vary from case to case. There are methods that can lead to higher levels of accuracy, which can be crucial in some situations but unnecessary in others. Fig. 1.2 This patient with longstanding epilepsy had new hemorrhage in the right central area (in green on the brain renderings in [A,B]). She had very brief “reflex”-type motor seizures. (A) Fusion of ictal SPECT data with a volumetric MRI sequence. The area of activation in the right hemisphere has been rendered in red on the 3-D model and with activity predominantly within the posterior bank of the central sulcus. Note that there is also some mirror-image activation in the left hemisphere, which has not been rendered. (B) Functional MRI data fused with the same volumetric MRI sequence. The fMRI in this illustration was performed with motor activation of the left hand, and this is rendered in blue on the 3-D model. Fig. 1.3 This is a further example of multimodal image fusion from the same patient as in Fig. 1.2. (A) A 3-D-rendered brain from MRI with contrast as viewed from above. Note the rendered sagittal sinus and cortical veins. The area of hemorrhage is again rendered in green. (B) This image illustrates the rendered SPECT activation (in red) shown in Fig. 1.2A as seen from this angle. (C) Left leg motor activation fMRI data (in purple on 3-D model) fused with the same volumetric sequence. (D) This image illustrates the rendered left hand motor activation fMRI data (blue) shown in Fig. 1.2A, as seen from this angle. (E) The simultaneous 3-D renderings of (A-D). (F) The craniotomy exposure at surgery. This image was obtained by fusing the preoperative MRI brain volume with a postoperative volumetric CT and building a skull model of the craniotomy exposure. (G) The surgical exposure. The hemorrhagic lesion has been excised after stimulation motor mapping. Electrocorticography showed active spiking in the postcentral gyrus, which has undergone subpial transection including down into the posterior bank of the central sulcus. With the assumption that imaging protocols used are optimized for IGS, and that the mechanical accuracy of the various IGS systems has also been optimized, two main sources of localization error at surgery need to be considered here, registration and brain shift.47 When information for suitability for resective surgery is insufficient or when there is conflicting information between EEG, imaging and/or neuropsychology testing, invasive EEG studies may be indicated. When placing intracranial electrodes, IGS systems can be useful in targeting anatomical or pathological areas on imaging studies. Traditionally, penetrating depth electrodes (DE) have been placed stereotactically by means of stereotactic frames.10,12 Some institutions have used frameless IGS strategies for DE placement.3,53,54 To optimize the accuracy and reliability of DE placement, the author strongly recommends using implanted screw fiducials for subsequent imaging and registration. This is a perfect example of the need for optimized application accuracy.
Development of IGS
Stereotaxis
CT/MRI
Early IGS in the 1990s
Is IGS Necessary for Epilepsy Surgery?
IG for Preoperative Assessment and Planning
Preoperative Analysis
Quantitative MRI
Image Fusion
Multimodal Correlation
Functional Correlation
Risk versus Benefit Assessment
Application Accuracy
IGS for Investigative Epilepsy Surgery
Depth Electrodes
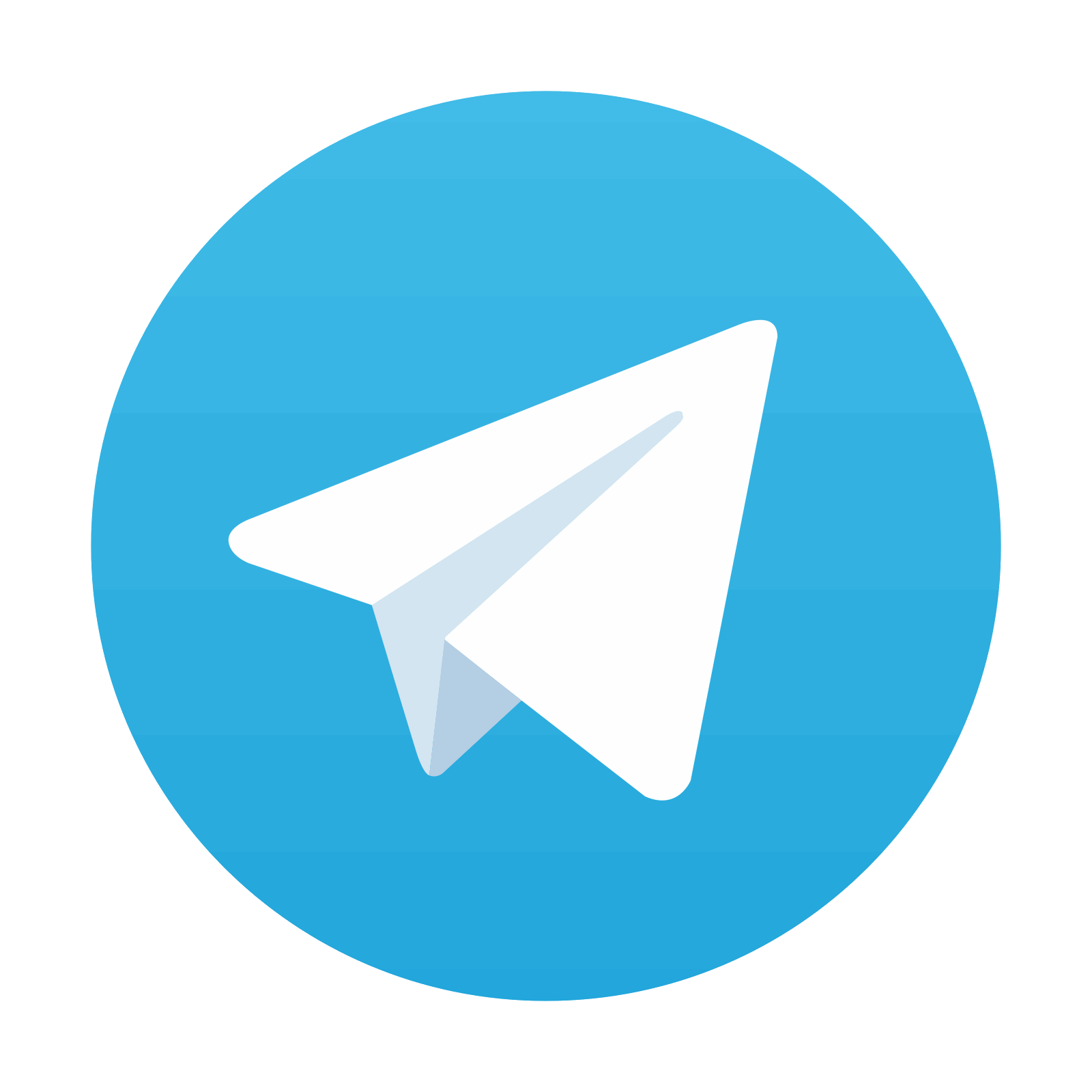
Stay updated, free articles. Join our Telegram channel

Full access? Get Clinical Tree
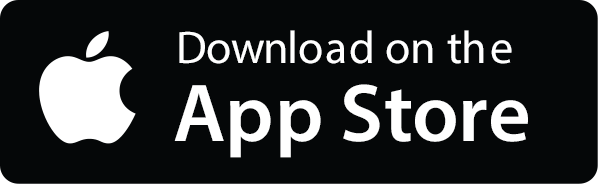
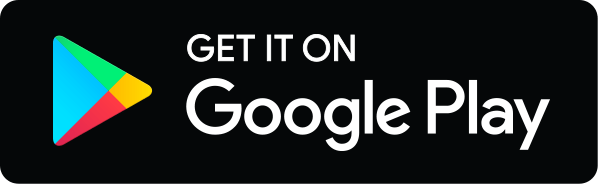