Image-Guided Intensity-Modulated Radiotherapy for Spinal Tumors: A Review
Yoshiya Yamada
Michael D. Lovelock
Mark H. Bilsky
INTRODUCTION
Recent technologic innovations have the potential to make significant improvements in patient outcomes for those treated with radiation therapy. These new technologies include miniature linear accelerators mounted on a robotic arm (Cyberknife, Accuray, Sunnyvale, CA) or mounted in a ring gantry (TomoTherapy, Inc., Madison, WI), as well as improvements and modifications of more traditional gantry-based linear accelerators using gantry-based kilovoltage imaging systems such as the Novalis (Brainlab, Westchester, IL) or cone beam-capable machines such as Synergy (Elekta, Stockholm, Sweden) or Trilogy (Varian Medical Systems, Palo Alto, CA). These technologies all allow the accurate delivery of highly conformal high-dose radiation, by providing a reliable platform to give very high radiation near sensitive structures such as the spinal cord. The improved outcomes that are demonstrated with these technologies are starting to change the traditional treatment paradigm of spinal tumors.
A multidisciplinary approach to spine tumors is important to provide the optimal treatment approach. Systemic therapy, radiation therapy, and surgical interventions, on their own, often result in less than satisfactory outcomes for spine tumors. Surgical resections are often limited by adjacent normal structures, such as the dura. Also, patients with metastatic disease may not be able to tolerate aggressive surgery of the spine. A recently reported randomized trial found significant benefit with the use of surgical resection with conventional radiation therapy compared with conventional radiation alone for high-grade epidural spinal cord compression (1). However, for patients who do not have high-grade epidural cord compression, or who do not have other indications for surgery, management remains controversial. Although many important recent advances in systemic therapy have been demonstrated, systemic therapy for bony metastases is often is less effective than that in soft tissues.
Conventional radiotherapy has been used for many years in the management of spine metastases, with less than ideal results (30% to 50% durable control) (2). One important limitation is the dose, which must be limited to respect spinal cord tolerances. It is delivered by using a shaped photon beam produced by a linear accelerator mounted on a gantry, with a limited number of radiation beams (typically two beams that oppose each other from the anterior and posterior directions). The gantry rotates about the patient in a transverse plane, allowing the gantry angles to be chosen such
that the beam passes through the target volume while avoiding critical structures. A structure such as the spinal cord is very difficult to avoid with radiation beams, because of its proximity to spinal tumors and its central location. Multiple beams from different directions may be used in an effort to miss the spinal cord; however, all beams must strike the target. It is difficult to miss the spinal cord without also underdosing part of a nearby tumor (3).
that the beam passes through the target volume while avoiding critical structures. A structure such as the spinal cord is very difficult to avoid with radiation beams, because of its proximity to spinal tumors and its central location. Multiple beams from different directions may be used in an effort to miss the spinal cord; however, all beams must strike the target. It is difficult to miss the spinal cord without also underdosing part of a nearby tumor (3).
Although each beam deposits dose within the patient from its entry to its exit on the opposite side of the patient, the doses combine only within the volume of the target where the beams overlap. In regions of the body away from the target, the dose is much lower. Thus it is possible to deliver tumoricidal doses to the target while keeping doses to other structures no greater than one seventh of the target dose in an equally weighted seven-beam non-coplanar plan, for example.
INTENSITY-MODULATED RADIATION THERAPY
An important development in radiation therapy was the introduction of the multileaf collimator (MLC). It consists of pairs of opposing tungsten fingers, or leaves, and resides in the head of the treatment machine. The position of each leaf can be set such that the gap between the opposing leaves can be adjusted, both in width and position, anywhere within the radiation field of the machine. An MLC is equipped with up to 60 opposing leaf pairs. The width of each leaf, depending on the manufacturer, varies from 1 to 3 mm. The use of an MLC allows each field to be set up automatically with greater speed and better geometric accuracy than the custom collimators or blocks.
The most important consequence of multileaf collimation, however, is that it has enabled intensity-modulated radiation therapy (IMRT). This is one of the most significant advances in the field of radiation oncology in the past 20 years. By sliding in and out of an active radiation beam, a portion of the radiation field can be modified or modulated in intensity. Thus the MLC can be used to vary the integrated intensity of the photon beam as a function of position within the aperture. The result of multiple intensity-modulated beams is a cloud of radiation that conforms tightly to the threedimensional (3D) characteristics of the target volume (Fig. 6.1)
The goal of highly conformal radiation therapy is to deliver a tumoricidal dose to the target while minimizing the dose to normal tissues. IMRT, when used in conjunction with a software tool known as inverse treatment planning, provides an approach through which this goal can best be achieved. With this approach, the 3D volumes of the target and critical structures are first defined by manually delineating them in a computed tomography (CT) scan. Further information on target extent may come from positron emission tomography (PET) or magnetic resonance imaging (MRI) scans, which can be fused with the CT scan.
Another important component of IMRT is the use of inverse treatment planning. Inverse treatment planning differs from the above by starting with the final dose distribution and working backward toward the intensities for each beam. Specific dose constraints are assigned to organs and volumes of interest. Such constraints may include specifying a minimal dose to the target and a minimal dose uniformity within the target. Doses to normal structures are constrained by limiting the maximal dose within these structures to acceptable levels. The inverse-planning algorithm is then run to find the intensity distributions for each beam, such that the sum of the distributions from each modulated beam best satisfies the specified constraints. For the treatment of paraspinal
disease, such optimized distributions may partially or completely wrap the spinal cord, while featuring steep dose gradients to allow maximal coverage of target near to the spinal cord, while keeping the cord dose below a safe limit.
disease, such optimized distributions may partially or completely wrap the spinal cord, while featuring steep dose gradients to allow maximal coverage of target near to the spinal cord, while keeping the cord dose below a safe limit.
![]() FIGURE 6.1 Highly conformal IMRT plan with sparing of the spinal cord. A myelogram was performed to identify the spinal cord. |
Typically, with the use of IMRT, a sharp dose gradient of approximately 10% per millimeter can be achieved near the spinal cord (4). Thus if the spinal cord were even 2 to 3 mm from the edge of the target volume, 7,000 cGy could be delivered, whereas the maximum dose to the cord surface would be less than 5,000 cGy.
A technique called “dose painting” can be used in IMRT plans to provide higher doses of radiation to volumes with the highest population of tumor cells, while simultaneously giving areas less likely to harbor tumor cells a lower dose, matching radiation doses with biologic characteristics of the target volume. For example, a higher dose of radiation can be given to the target volume with gross disease (gross tumor volume or GTV), while a lower dose can be prescribed to the volume that may include microscopic disease (clinical target volume or CTV). Dose painting can be a useful technique to spare excessive dose to nearby critical structures.
IMMOBILIZATION
The tumor (and the patient) must remain stationary in the correct position throughout dose delivery. The techniques used can be grouped into two approaches. In the first, the patient is immobilized in a stereotactic body frame or immobilization cradle. In a study of patients treated with IG-IMRT, patient-positioning errors were measured after positioning patients into a customized noninvasive cradle. Up to 7 mm of positioning error was detected in 30 patients repositioned at least 10 different times. However, once image-guided corrections were performed with immobilized patients, an analysis of 650 images, the standard deviations, as determined by the imaging procedure, were 1.3 mm, 1.6 mm, and 2.1 mm in the anterior/posterior (ant/post), right/left, and superior/inferior directions. Immobilization was assessed by measuring patient shifts between localization
images taken before and after treatment. From 67 ant/post image pairs and 49 lateral image pairs, the standard deviations were found to be less than 1 mm in all directions (5). It appears that once the necessary corrections in positioning are made, the spine can be reliably immobilized in the correct position for the duration of treatment to within ± 1 mm of error. Stereoscopic infrared cameras are also used to track reflective markers attached to the patient to provide continuous monitoring while the beam is on. Less than 2 mm of variation is the norm.
images taken before and after treatment. From 67 ant/post image pairs and 49 lateral image pairs, the standard deviations were found to be less than 1 mm in all directions (5). It appears that once the necessary corrections in positioning are made, the spine can be reliably immobilized in the correct position for the duration of treatment to within ± 1 mm of error. Stereoscopic infrared cameras are also used to track reflective markers attached to the patient to provide continuous monitoring while the beam is on. Less than 2 mm of variation is the norm.
A second approach is used when localization images can be taken frequently during the treatment without greatly lengthening the procedure. It is especially suited to procedures in which dual in-room kV units are available. The patient can be set up conventionally on the treatment-machine couch without additional immobilization. The positions of the bony structures are checked many times during treatment; thus any shifts in position can be detected and quickly corrected.
IMAGE-GUIDED PATIENT SETUP
Precise positioning of spinal targets can be accomplished by using an image-guided patient setup. Pretreatment localization images of the target are acquired just before treatment. These are registered with corresponding reference images to determine any setup error. These errors are then corrected, usually by making small translations to the treatment-machine couch. The traditional image-guided technique has been to use the megavoltage treatment beam to take an image of the bony anatomy. Potential problems of this approach include poor definition of the bony structures in the MV localization images, especially in larger patients. Gold fiducial markers that can be seen clearly in the localization images are often implanted into the spine to improve the registration accuracy. The reference image itself may also have relatively poor spatial resolution in the cranial-caudal direction. This can arise because it is constructed from the planning CT, which may have poor longitudinal resolution because of slice spacing.
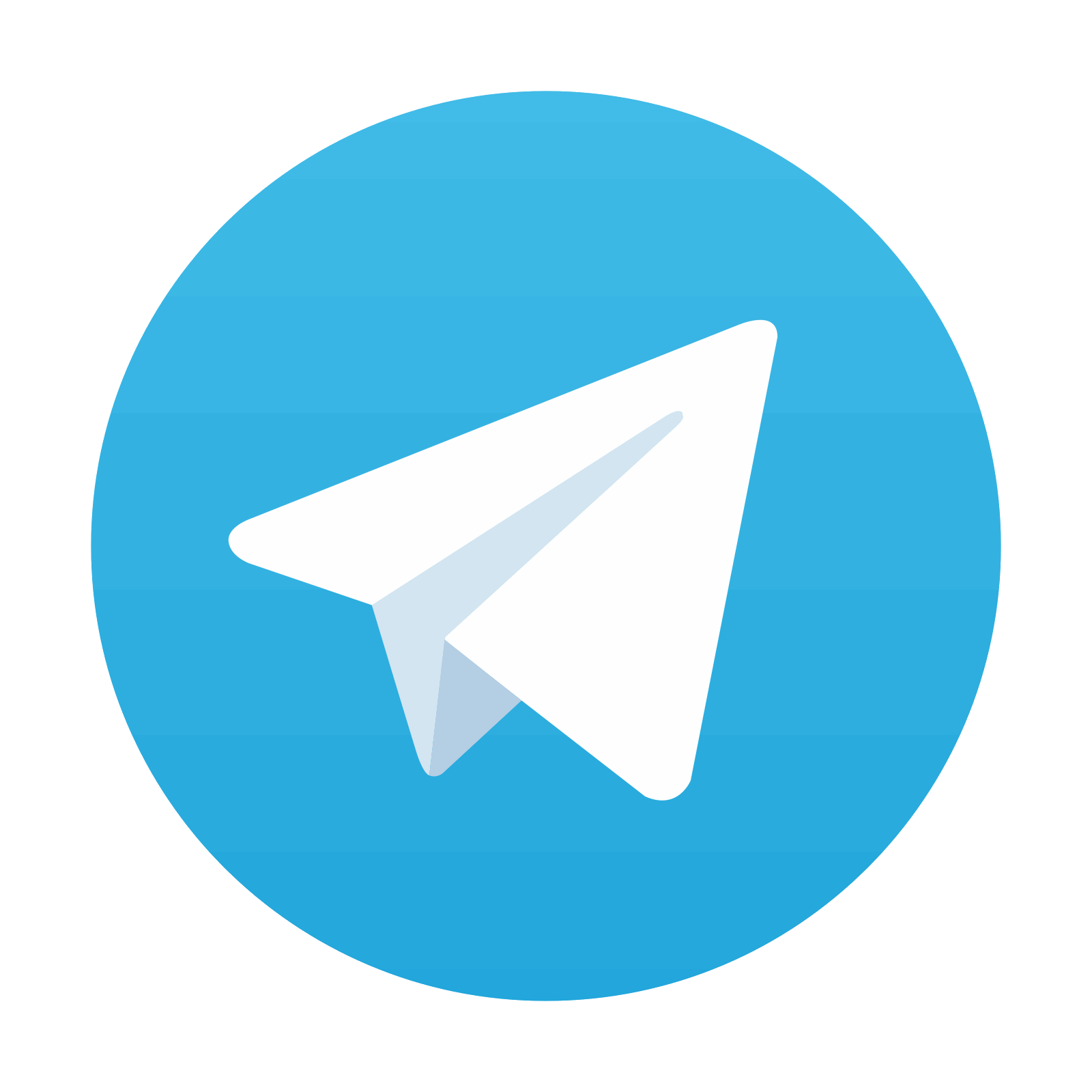
Stay updated, free articles. Join our Telegram channel

Full access? Get Clinical Tree
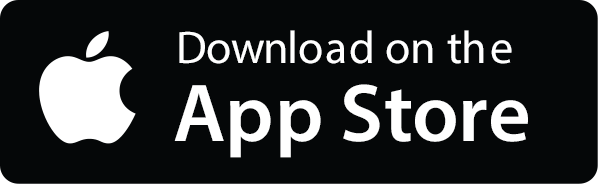
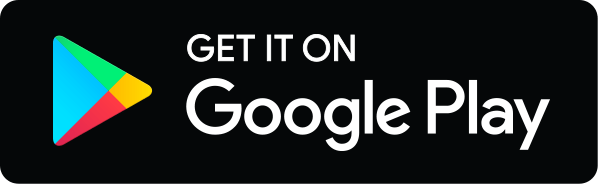