Fig. 29.1
Non isocentric treatment planning delivering staged image-guided radiosurgery using a Cyberknife G4 model to a right ONMS presenting with visual failure in a 28 year old woman. It can be noted the ability of this frameless radiosurgical modality to deliver beams through the basal frontal,orbital and maxillary regions
Because of their intimate relation to the optic nerve and their sharing of a common pial blood supply, ONSMs present a rather unique treatment challenge. Even in very experienced hands, blindness typically follows microsurgical resection of ONSMs (Cristante 1994; Delfini et al. 1996).
This and the typically slow development have encouraged a conservative, watch-and-wait approach reserving the surgical resection for patients with a long history and near-complete or complete loss of vision in the affected eye. Nevertheless, progressive deterioration of vision over time has been reported in approximately 85 % of patients with prolonged follow-up (Dutton and Anderson 1985; Turbin et al. 2002), while long-standing visual deficits are less likely to be reversed by treatment.
Conventional radiotherapy, either as an adjuvant to surgery or alone, has been shown to be moderately effective and reasonably well tolerated. A more conformal technique developed in the last decade, stereotactic fractionated radiation therapy (SFRT) has been successfully applied to the treatment of ONSMs (Jeremic and Pitz 2007; Pitz et al. 2002). Primary use of SFRT has preserved vision in patients with ONSM better than observation alone. Based on its safety and efficacy, and concerns about injury from single-session stereotactic radiosurgery (SRS), SFRT has been proposed as a standard treatment approach for ONSM. Nevertheless, residual dose spillage over the retina and other nearby structures has occasionally led to loss of vision due to radiation retinopathy or vascular occlusion of retinal vessels. Long-term ophthalmic, adnexal and peripheral complications secondary to conventional irradiation or SFRT have been reported and include iritis, temporal lobe atrophy and endocrine failure (Parsons et al. 1994a, b). Optic neuritis has been also described following SFRT (Andrews et al. 2002).
Stereotactic radiosurgery involves the precise delivery of a very high dose of radiation with the goal of ablating a small-to-medium-sized intracranial target, with sparing of nearby structures. While SFRT relies on a combination of tissue sparing and daily fractionation to protect the optic nerve, radiosurgery provides extremely tight dose distributions and conformality, restricting the irradiation to the tumor and offering the highest degree of perilesional tissue protection, with an unchallenged 80–20 % dose fall-off within 3 mm of the target. Frame-based radiosurgery requires drastic measures for the immobilization of the patient and accurate targeting of the lesion, with the entire treatment being typically delivered in a single session, or stage, of 12–14 Gy, a dose exceeding the tolerance of the optic nerve (Girkin et al. 1997). Nevertheless,it was recently suggested that effective single session radiosurgery may carry an acceptable risk of radioinduced optic neuropathy (Liu et al. 2010).
An emerging radiosurgical technique, frameless image-guided robotic radiosurgery, eliminates the need of a stereotactic frame, introducing multisession (or hypofractionated) radiosurgery as a safe and efficacious new treatment option for ONSM and other lesions affecting radiosensitive structures such as the the cranial nerves or spinal cord (Romanelli and Adler 2008). Hypofractionated SRS delivers high doses of radiations in multiple sessions (usually two to five) while keeping the highest degree of conformality and accuracy, with consequent sparing of the adjacent critical structures. Staged image-guided robotic radiosurgery has been applied to the treatment of ONSM with promising preliminary results (Romanelli et al. 2007, 2011).
Role of Surgery for ONSM
ONMS confined in the orbit are approached via an orbitotomy. The surgical approach, however, depends on the location of the meningioma in relation to the optic nerve (Boulos et al. 2001). A craniotomy is required in cases of intracranial extension while unroofing of the optic canal is often required to provide decompression (Shimano et al. 2000). En bloc resection of the optic nerve together with the tumor is likely the best strategy in patients in whom functional vision is lost, especially if there is extensive intracranial extension. Orbital exenteratio is performed when the orbit is invaded by tumor beyond the optic nerve (Alper 1981).
Encasement of the optic nerve by an ONSM makes it impossible to obtain a radical resection without causing severe injury to the nerve itself or to its vascular supply, which is peripheral and completely shared by the tumor. As a consequence, vision preservation following microsurgical resection of ONSMs is rare (Alper 1981; Clark et al. 1989). In particular, as a general attitude toward the most common type of ONSMs, a fusiform sheath growing around the optic nerve, most surgeons do not even attempt to dissect the nerve but directly proceed to the resection of the nerve and the tumor together. Surgery is therefore considered in patients with disfiguring exophthalmos and blindness. Young patients with good vision may harbor meningiomas that behave more aggressively and thus carry a poor prognosis. In these cases the risk of chiasmal involvement and bilateral loss of vision should be contemplated and early surgery may be attempted.
Stereotactic Fractionated Radiation Therapy
In 2002 Egan and Lessell published an observational study on 42 patients with ONSM (Egan and Lessell 2002) and reported that, with a 6.2-year follow-up, approximately 50 % of the untreated patients maintained stable visual acuity and 3 out of 16 patients actually showed a slight improvement over time. These results prompted the researchers to warn the neurosurgery community against treating patients with ONSM with stable conditions and thereby subjecting them to unnecessary risk.
On the other hand, a relatively large retrospective study published in 2002 (Turbin et al. 2002) included 64 patients that were either simply observed or treated with surgery only, with radiotherapy only, or with a combination of surgery and radiotherapy. Although the very different patient characteristics and treatment regimens make definitive conclusions difficult, the radiation-only group demonstrated the fewest complications and the smallest degree of deterioration of visual acuity.
SFRT is a technique by which it is possible to use linear accelerators with multiple fields and intensity modulation to obtain radiation dose distributions that are more conformal with relatively sharp dose gradients on the structure surrounding the tumor. This technique produces conformal dose distributions and relatively steep dose gradients with consequent substantial sparing of surrounding normal tissues and is clearly a more sophisticated and safer treatment option than conventional radiotherapy. SFRT has been therefore increasingly used to treat ONSMs. Favorable short-term results have been published (Andrews et al. 2002; Jeremic and Pitz 2007; Moyer et al. 2000; Narayan et al. 2003; Pitz et al. 2002; Turbin et al. 2002), and primary SFRT has been advocated to preserve vision in patients with ONSM rather than undertaking observation alone (Andrews et al. 2002; Turbin et al. 2002). Accordingly, SFRT can be considered a standard treatment approach for ONSM.
However, SFRT cannot reach the extremely tight dose distribution typical of radiosurgery and may still allow radiation doses leaking over the retina and other nearby structures. As a consequence, radiation retinopathy, loss of vision, and endocrine failure may still occur after SFRT for ONSMs (Becker et al. 2002; Subramanian et al. 2004). Radiation optic neuropathy has also been described after low-dose treatment (40–45 Gy delivered at 2 Gy/fraction) of pituitary lesions. Furthermore, several other long-term ophthalmic and adnexal complications following external-beam radiotherapy and SFRT have been described (Durkin et al. 2007).
Stereotactic Radiosurgery
The optic nerve is one of the most sensitive structures to radiations. Goldsmith et al. (Goldsmith et al. 1992), predicted, based on clinical data, that doses to the optic nerve or chiasm less than or equal to 890 “optic ret” would be safe.
The optic ret is based on the total dose and the number of fractions used:

where N is the number of fractions used and D is the total physical dose in cGy. Therefore for a particular number of fractions, N, the optic tolerance would be predicted to be total dose D, where
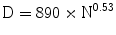

(29.1)
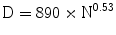
(29.2)
According to this model the maximal dose to the optic nerve should be lower than 890 cGy in single fraction. Even though doses of up to 11 Gy–1 cm3 of the volume of the nerve are considered safe, at least 13 Gy are necessary to achieve a sufficient probability tumor control of a meningioma. Therefore, it is straightforward that, for ONSMs, it is impossible to achieve a therapeutic ratio between normal tissue complication probability (NTCP) and tumor control probability (TCP).
Despite this widely recognized issue, results on the use of single-session radiosurgery has been recently reported (Liu et al. 2010; Smee et al. 2009). Liu et al. (2010) reported the long-term results of 30 patients who underwent gamma-knife radiosurgery for ONSM. The average dose was 13.3 Gy achieving, at a median follow-up of 56 months, a tumor control in 93 % of patients. Visual acuity improved in 11 patients, remained stable in 13 patients (including 4 patients who were completely blind before GKS), but deteriorated in 6 patients (20 %). These results demonstrates that SRS can reach an high TCP, but carries also carries significant risk of vision impairment and should be reserved mostly to patients who have lost vision already.
Image-Guided Robotic Radiosurgery
The CyberKnife is a frameless 6 MV LINAC system for image-guided robotic radiosurgery (Conti et al. 2012; Romanelli and Adler 2008; Romanelli et al. 2009). Real-time image guidance based on digitally reconstructed skull radiographs is used to localize and treat the targeted site. The robotic arm provides great flexibility and the highest number of noncoplanar penetration trajectories (1,600 with the new G4 model). Radiation is delivered with submillimetric accuracy. The system presumes a fixed relationship between the target and the skull, as with other forms of stereotaxy. A light-weight 6-MV LINAC is accurately positioned by a robotic arm with 6 degrees of freedom.
Two x-ray imaging devices positioned on either side of the patient’s anatomy acquire real-time digital radiographs of the skull at repeated intervals during treatment. Amorphous silicon sensors create a high-quality image of the skull or spine with a modest exposure to radiation (10 mA, 75 kV; corresponding to a dose per image of ~25 mrad).
The images acquired during the treatment are automatically registered to digitally reconstructed radiographs derived from the treatment planning CT scans. This registration process allows the position of the skull (and thus the treatment site) to be translated to the coordinate frame of the LINAC. A control loop between the imaging system and the robotic arm adjusts the pointing of the LINAC therapeutic beam to the observed position of the treatment anatomy (target). If the patient moves, the change is detected during the next imaging cycle and the beam is adjusted and realigned.
Frameless radiosurgical technology is characterized by the ability to perform hypofractionated treatments. Hypofractionation allows the delivery of ablative, radiosurgical doses to the treated lesion in association with an enhanced protection of the adjacent tissues receiving lower doses and lower dose rates but also being allowed precious time to recover between fractions. Frameless hypofractionated radiosurgery of lesions affecting the cranial nerves and spinal cord substantially enhances treatment safety and prevention of dreaded neurological complications (Romanelli and Adler 2008). Furthermore, frameless systems are not limited to delivering spherical dose distributions (i.e., treatment planning and delivery can be nonisocentric). Early radiosurgical systems treated nonspherical targets by delivering radiation to multiple isocenters packed inside the treated volume. Nonisocentric planning provides a straightforward approach for the treatment of irregularly shaped lesions, and can result in a more homogeneous radiation distribution that is usually very conformal to the shape of the target lesion.
The CyberKnife offers a distinct advantages in the treatment of optic pathway lesions due to the absence of a rigid frame placed just under the eyes, banning beam penetration through the lower frontal, orbital, temporal and maxillary fields. Frameless radiosurgery allows the opening of this strategic regions to beam delivery, providing enhanced dose distribution to the tumor and sparing of the optic nerve. By delivering treatment in multiple sessions it is possible to exploit the differential speed of recovery of normal and pathological tissues, thereby limiting or avoiding damage to the visual pathways while controlling tumor growth. Also, inverse planning software is used to optimize target coverage and achieve tightly conformal isodose distributions with substantial sparing of surrounding brain tissue. Nonisocentric beam delivery (as opposed to isocentric multishot technology) also enables a significant measure of dose homogeneity.
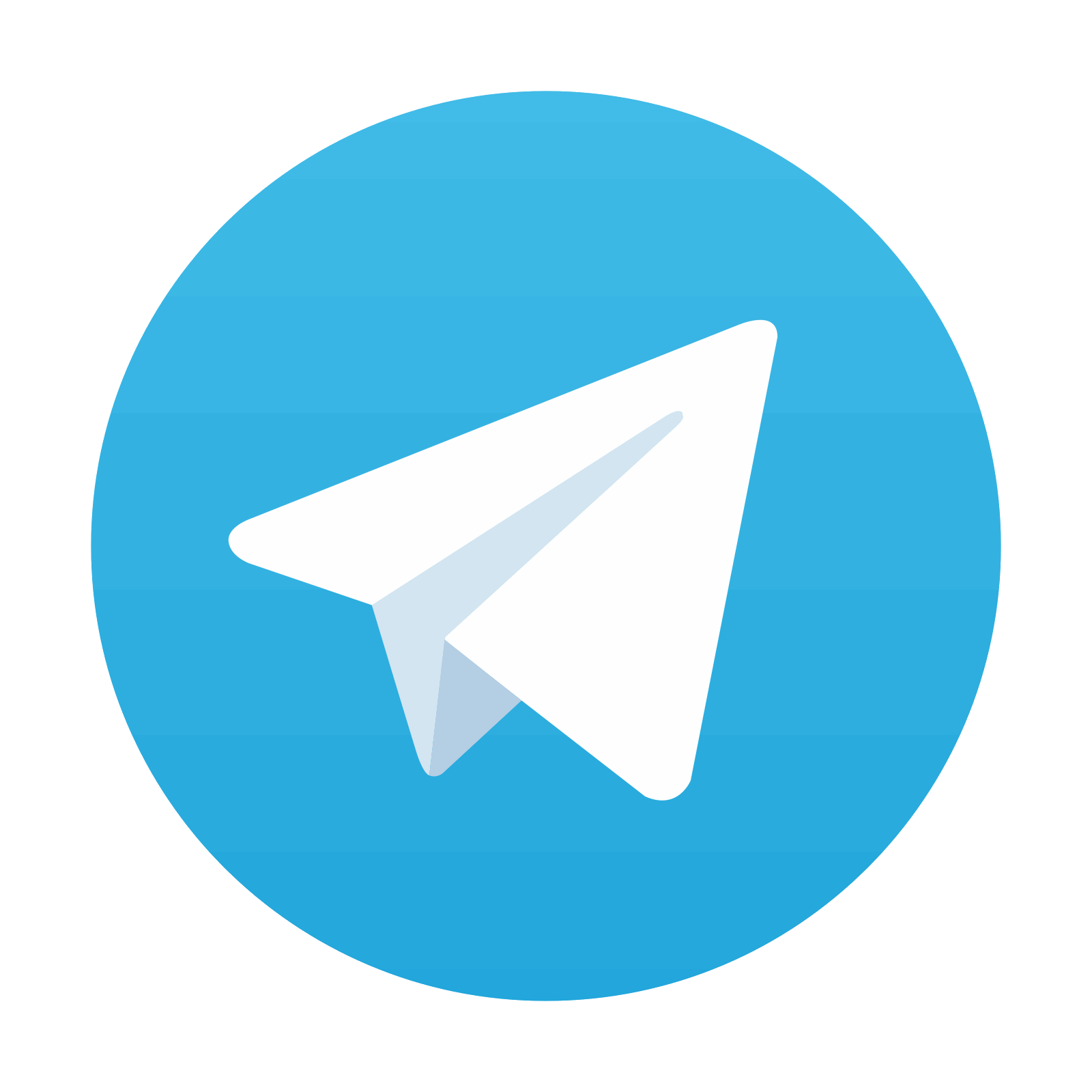
Stay updated, free articles. Join our Telegram channel

Full access? Get Clinical Tree
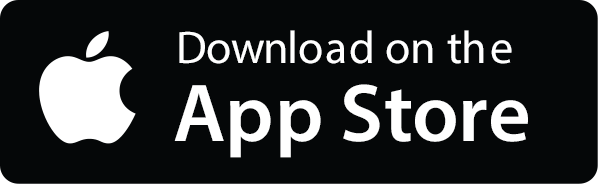
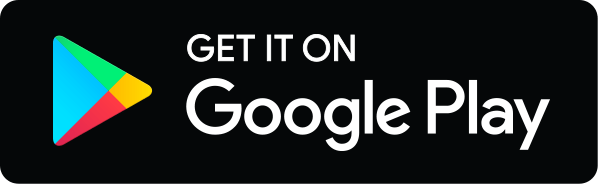