Fig. 12.1
Neuroimaging paradigms in assessment of residual cognitive processes in DOC
12.2.1 Active Paradigms
Active paradigms refer to mental imagery tasks which measure wilful modulation of brain signal in specific brain areas, aiming to detect command-following. Command-following in patients with DOC is of major clinical importance because, according to standardized behavioural assessment, this behaviour differentiates patients in MCS from patients in UWS (Bruno et al. 2011b; Giacino et al. 2004). At the bedside, command-following is tested by asking patients to follow simple object-related and the non-object-related commands. Those patients that can follow the commands “consistently”2 or “reproducibly”3 are said to be in MCS. In the fMRI environment, two mental imagery tasks have been shown to encompass reproducible cortical activations across healthy controls, namely, thinking about playing tennis (encompassing primarily supplementary motor area) and imagining visiting the rooms of one’s house (encompassing primarily parahippocampal cortex; Fig. 12.1, left panel) (Boly et al. 2007). When this paradigm was employed in a behaviourally unresponsive patient, her brain activity was indistinguishable from healthy controls. Since this patient was able to comprehend and execute the mental imagery commands in a sustainable manner, the behavioural diagnosis was challenged, and the patient was no longer considered as in UWS (Owen et al. 2006). In a larger cohort (n = 54) of patients with DOC, the same paradigm confirmed that not all behaviourally assessed VS/UWS patients were unresponsive (Monti et al. 2010). Indeed, command-following was observed in five patients, two of whom showed no signs of awareness when evaluated behaviourally. Interestingly, one patient was able to further use this technique to provide yes/no responses to autobiographical questions which could not be elicited at the bedside. Based on the command-following rationale, other mental tasks for evidencing response to command in patients with DOC have been employed. For example, with a silent picture-naming task, complete and partial preservation of the object-naming brain network was observed for all patients in MCS and two in VS/UWS (Rodriguez Moreno et al. 2010). By instructing patients in VS/UWS to move their hand, voluntary behaviour was also evidenced for two patients who activated premotor cortex (consistent with movement preparation) in the absence of overt muscle activity (Bekinschtein et al. 2011). Similarly, using selective auditory attention, all 3 assessed patients (2 MCS and 1 VS/UWS) were able to convey their ability to follow commands, and 2 of them (1 MCS and VS/UWS) were even able to use attention to correctly communicate answers to several binary questions (Naci and Owen 2013). Residual cognitive capacities in patients DOC has also been assessed using a hierarchical fMRI approach, starting from command-following tasks similar to those described above, to question tasks using binary choice responses and eventually multiple choice responses (Bardin et al. 2011).
A criticism of using mental imagery tasks to unfold cognition and/or to communicate relies on patients’ limited short-term memory resources and restricted attention span. As a result, relatively long scanning intervals might be necessary to increase the signal-to-noise ratio, which in turn contributes in patients’ fatigue and ultimately in lack of their vigilance (Naci et al. 2013). Additionally, patients may suffer from other cognitive (i.e. aphasia, apraxia) and sensory impairments (i.e. blindness, deafness), from small or easily exhausted motor activity, pain, sedative medication, sleep disturbances and/or medical complications (such as infections), which can interfere with the direct assessment of command-following. In these cases, however, absence of responsiveness does not necessarily correspond to absence of awareness (Sanders et al. 2012). Alternatively, residual cognitive function in patients with DOC has been further assessed by means of passive and resting-state paradigms which overcome the above-mentioned limitations.
12.2.2 Passive Paradigms
Passive paradigms measure brain responses to external sensory stimulation (e.g. auditory, somatosensory and visual), while the subject is not performing any mental task. Using PET, the administration of simple auditory clicks in patients in VS/UWS was shown to activate primary auditory cortices (Laureys et al. 2000c), whereas patients in MCS demonstrated more widespread activation in the secondary auditory cortex, as well as temporal and frontal areas (Boly and Faymonville 2004). More recently a difference between patients in VS/UWS and MCS was observed in the impairment of backward connectivity from frontal to temporal cortices, as evidenced by an EEG mismatch negativity paradigm using auditory clicks (Boly et al. 2011) (see also Chap. 9). When more complex auditory stimuli were administered, differential brain responses between patients and controls, as well as between patient groups, were also observed. For example, sentences which were manipulated at different levels of auditory intelligibility4 and semantic ambiguity5 were presented to a patient in VS/UWS. The patient presented consistent and similar-to-controls responses in the auditory cortex as a response to intelligible speech stimuli as well as partially intact responses to semantically ambiguous stimuli (Owen and Coleman 2005). Also, in an fMRI task of passive listening to narratives played forward and backward, one patient in VS/UWS (out of three) and one patient in MCS (out of four) showed cerebral responses very similar to healthy controls (Fernández-Espejo et al. 2008). Another salient auditory stimulus which has been preferred because of its attention-grabbing properties is the patient’s own name. With the own-name paradigm, it was shown that one patient in VS/UWS exhibited activation in medial prefrontal cortex, left temporoparietal and superior frontal cortex, which is again similar to activation observed in controls (Staffen et al. 2006). With a similar own-name paradigm, two out of seven patients in VS/UWS and all four patients in MCS showed activation not only in the primary auditory cortex but also in higher-order associative temporal areas, which are thought to be implicated in the conscious processing of the incoming stimuli. Interestingly, these two patients in VS/UWS subsequently recovered to MCS as observed 3 months after their fMRI scan, highlighting the prognostic value of this paradigm (Di et al. 2007). This prognostic utility was further supported by data of seven out of eight patients in VS/UWS who progressed to MCS and showed speech-specific or semantic responses to sentence stimuli 6 months earlier in their fMRI assessment (Coleman et al. 2009).
In the somatosensory modality, painful electrical stimulation of the median nerve of the wrist encompassed the entire “pain matrix” (including the anterior cingulate cortex and insular areas; Fig. 12.1, middle panel) in patients in MCS. On the other hand, patients in VS/UWS showed restricted activation to lower-level subcortical and primary cortical areas (Boly et al. 2008; Laureys et al. 2002), indicating that MCS patients are more likely to experience the administered stimuli as painful.
In the visual modality, when pictures of different emotional valences were presented to patients in MCS by means of fMRI, visual activation similar to healthy controls was found for patients (Zhu et al. 2009). A more recent case study on visual cognition used a battery of tests in a MCS patient. Specifically, the battery first assessed passive visual processing whereas, at the final level, it assessed the ability to voluntarily switch visual attention though the focus on competing stimuli. This approach revealed appropriate brain activations, undistinguishable from those seen in healthy and aware volunteers suggesting that the patient retained the ability to access his own visual representations (Monti et al. 2013).
Taken together, the rationale behind passive experimental paradigms is that an indistinguishable response between patients and controls is indicative of covertly preserved cognitive processing in these patients (Owen 2013). Generally, these paradigms have shown that auditory, visual and somatosensory activation is restricted to lower-level sensory regions in patients in VS/UWS, while brain activation is widespread in MCS reaching higher-level associative areas. The limitations of using this approach stem from patients’ pathologies and technical requirements. Indeed, patients can present variant clinical picture, ranging from visual problems, motor spasticity, somatosensory hypersensitivity and cortical auditory deafness, which can prevent the administration of external stimuli. Also, the technical setup of these examinations are not always straightforward, and therefore cannot be widely used across medical and research institutions.
12.2.3 Resting-State Paradigms
Alternatively, increasing attention is being paid to resting-state paradigms (Soddu et al. 2011). In these paradigms, spontaneous brain function is assessed while subjects receive no external stimulation and are instructed to let their mind wander. As such, this approach surpasses the limitations which are raised by the other two types of experimental tasks.
Using PET at rest, it was shown that patients in VS/UWS exhibit decreased brain metabolism up to 40 % of normal value (Laureys et al. 2000b; Tommasino et al. 1995). Nevertheless, recovery from the VS/UWS does not coincide with the resumption of global metabolic levels. It rather seems that some areas are more critical to consciousness than others. Indeed, patients suffering from DOC show decreased metabolism in a widespread network encompassing frontoparietal areas, such as lateral prefrontal and posterior parietal regions as well as midline anterior cingulate/mesiofrontal and posterior cingulate/precuneal associative cortices (Nakayama et al. 2006; Silva et al. 2010). Importantly, recovery from the VS/UWS parallels the restoration of connectivity in these areas (cortico-cortical) but also between these regions and the thalamus (thalamo-cortical) (Laureys et al. 2000a, 1999). More recently, it was shown that patients in MCS retain metabolism in the lateral frontoparietal areas, whereas midline regions are highly dysfunctional (Thibaut et al. 2012). Broadly speaking, the midline cortices are assumed to mediate self-related cognition, whereas frontoparietal cortices are thought to mediate awareness of the environment (for a short review, see Demertzi et al. 2013a). As such, these data suggest that patients in MCS are characterized by altered self-awareness besides their abilities to, at least to a certain extent, interact (but not communicate) with their surroundings.
In fMRI, the resting-state network approach has been used lately to quantify various higher-order and sensory-related systems (Damoiseaux et al. 2006; Laird et al. 2011; Smith et al. 2009). These networks show differential connectivity alterations under different states of unconsciousness (Heine et al. 2012), highlighting their importance when assessing consciousness levels. In a recent investigation of fMRI resting-state connectivity in patients with DOC, it was found that, among the long-range systems, the default mode network (DMN, which encompasses precuneus, medial prefrontal cortex and bilateral temporoparietal junctions) and bilateral executive control or frontoparietal networks were severely disturbed in patients with DOC (Fig. 12.1, right panel) (Demertzi et al. in press). This implies that these systems might be important to sustain consciousness-related processes. Interestingly, it has been found that the resting brain is characterized by a switch between the dominance of the DMN (linked to “internal” or self-awareness) and the bilateral frontoparietal network (linked to “external” or environmental awareness; Fox et al. 2005; Fransson 2005). More recently, it was found that such alternating pattern not only happens spontaneously in the brain but also has a behavioural counterpart. In other words, behavioural reports of “internal awareness” were linked to the activity of the DMN, whereas subjective ratings for “external awareness” seem to correlate with the activity of lateral fronto-parieto-temporal regions (Vanhaudenhuyse et al. 2010), which are part of the so-called executive control network, exhibiting increase of activity during attention-demanding cognitive tasks. These findings imply that the anti-correlated pattern between the internal (DMN) and external (executive control network) awareness systems is of functional relevance to the phenomenological complexity of subjectivity (Demertzi et al. 2013b). This assumption is further supported by evidence from patients who show severely disrupted connectivity in one or both systems. For instance, in a brain dead patient, functional connectivity of the DMN was absent (Boly et al. 2009), whereas in patients with DOC, albeit preserved, functional connectivity of the DMN showed consciousness level-dependent decreases (Soddu et al. 2012; Vanhaudenhuyse et al. 2010).
Next to the investigation of reduced connectivity, the presence of pattern of hyper-connectivity might also be informative of patients’ brain function. Indeed, it was recently showed that the subcortical limbic system (including the orbitofrontal cortex, insula, hypothalamus, and the ventral tegmental area) exhibits paradoxically increased fMRI connectivity with the DMN in patients with DOC (Di Perri et al. 2013). These results were considered as suggestive of a persistent engagement of residual neural activity in self-reinforcing neural loops, which, in turn, could disrupt normal patterns of connectivity in patients.
12.3 Conclusions
Functional neuroimaging has been employed to test imaging correlations in patients not able to communicate as a result of severe brain damage. Nevertheless, parallel to function, information about the brain’s anatomy also sheds light on the differential neuropathology of patients with DOC. For example, recent studies suggest that the structural connectivity assessment by means of DTI is severely disrupted in patients in coma. Specifically, the connections from the brainstem to the thalamus, also known as the ascending arousal system, have been shown to be seriously impaired in patients (Edlow et al. 2012). Although one could expect that patients with such severe structural damage show poor recovery rates, this is not necessarily the case, especially when the assessment of axonal injury is shortly after the accident. For example, structural imaging of a patient suffering from altered states of consciousness 8 weeks after a traumatic accident showed severe damage in the corpus callosum, brainstem and bilateral white matter; nevertheless, 1 year after injury, this patient had regained consciousness and reintegrated in the community (Edlow et al. 2013). In more chronic patients, it has been shown that structural connectivity assessment could correctly classify patients in UWS versus MCS with a 95 % accuracy in a group of 25 patients (Fernández-Espejo et al. 2011). These studies imply that the assessment of structural connectivity is of salient clinical importance especially when it is known that structure is linked to function (Sui et al. 2014). Ideally, maximal information about patients’ clinical picture can be obtained by combining different technologies (Bruno et al. 2011a; Gantner et al. 2013); the use of functional imaging together with electroencephalography is very promising, as recently done with transcranial magnetic stimulation and EEG (TMS/EEG; Casali et al. 2013; Rosanova et al. 2012) (see also Chap. 10). Multimodal assessment of patients using the aforementioned neuroimaging methods is expected to bring us closer to patient-specific underlying neuropathology, which in turn could aid diagnosis and prognosis (Bruno et al. 2011a).
Acknowledgment
Dr Rossetti is supported by the Swiss National Science Foundation [Grant CR32I3_143780].
References
Bardin JC, Fins JJ, Katz DI, Hersh J, Heier LA, Tabelow K, Dyke JP, Ballon DJ, Schiff ND, Voss HU (2011) Dissociations between behavioural and functional magnetic resonance imaging-based evaluations of cognitive function after brain injury. Brain 134:769–782CrossRefPubMedCentralPubMed
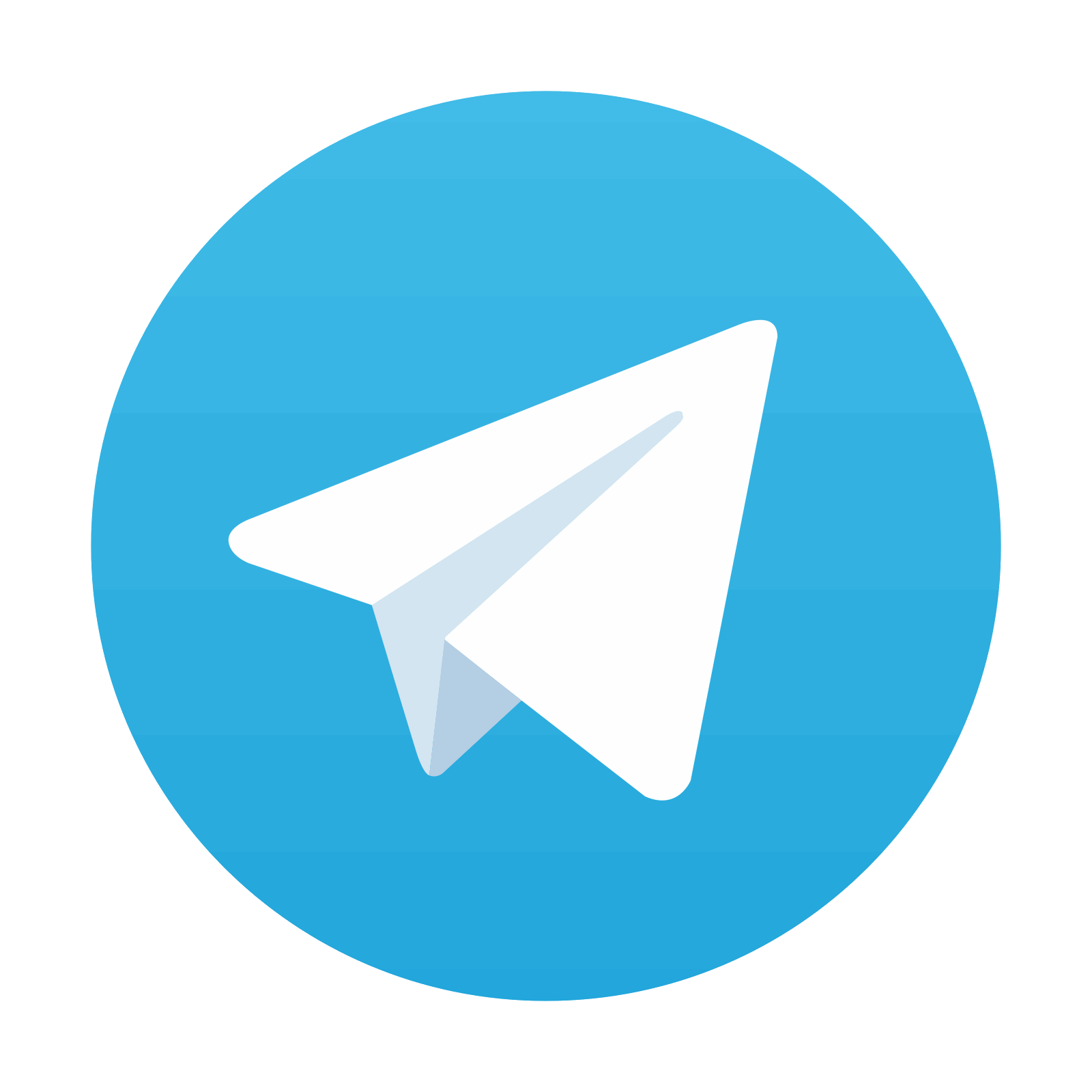
Stay updated, free articles. Join our Telegram channel

Full access? Get Clinical Tree
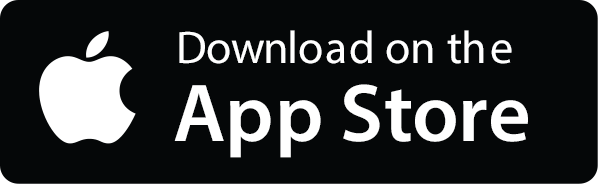
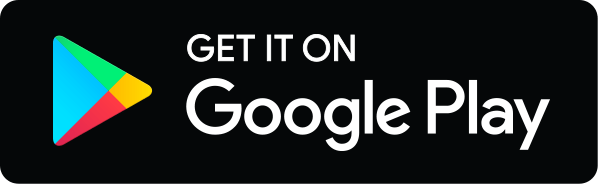