Fig. 6.1
Computed tomography (CT) scan of a 16-year-old girl with an earlier history of pansinusitis and a left frontal cerebritis and abscess who had undergone surgical treatment and presents 4 months later with nonconvulsive status epilepticus
Magnetic Resonance Imaging
MRI is an essential tool for the evaluation of patients with epilepsy, but may have a more limited application in SE. MRI technology, including equipment, field strength, and software are being optimized continuously for better resolution, and MRI provides far greater resolution of brain structures compared to that of other imaging. Rather than radiation, MRI uses radiofrequency pulses and magnetic fields to change atomic spins temporarily and generate images. Although the lack of ionizing radiation is an advantage, magnetic fields can be dangerous in patients with internal pacemaker wires, stimulators, or other ferromagnetic implants. MRI has become widely available, but high field strength scanners and several particular scanning sequences and acquisition protocols may be available at multidisciplinary epilepsy centers only.
Among potential limitations of MRI, a routine study of the brain may take from 30 to 60 min, and a high-resolution epilepsy-focused MRI, 60–90 min. Immobility of the patient is essential for maximal image resolution; even subtle movement, including swallowing or eye blinking, can compromise the image quality. Usually, multiple sequences must be obtained, requiring greater cooperation by the patient to remain still. For these reasons, patients in NCSE may have an initial screening MRI to assess for acute changes, followed by a separate study to improve resolution of the imaging. The sequences used in epilepsy-focused protocols can take as long as 9–11 min each—compared to 2–4 min each for screening studies. MRI-compatible surgical clips, coils, shunts, and other hardware can also distort the images. Patients needing ventilatory support require MRI-compatible ventilators and monitors. Patients with surface EEG electrodes (e.g., those on continuous EEG monitoring) who require urgent imaging must be confirmed as having MRI-compatible electrodes first. Others patients have MRI performed prior to or after monitoring.
While most standard screening MRI examinations of the brain are sufficient to evaluate gross pathology, subtle pathology (which is now more often recognized in epilepsy patients) is seen only with high-resolution MRI studies that include thin-section isotropic 3-D spoiled gradient T1-weighted images, ideal for evaluating cortical morphology and the gray-white matter interface.
The exquisite resolution of images and multiplanar capabilities of MRI provide a significant advantage in evaluating seizure patients. Tumors, infection, or inflammatory lesions can cause seizures and epilepsy and are often seen readily by MRI, and the extent of surrounding edema and mass-effect can be seen easily as well. Developmental or structural disorders are best detected by MRI, including large structural deformities such as schizencephaly and lissencephaly, as well as more subtle heterotopias and cortical malformations (although visualizing the latter abnormalities may not be possible during an episode of SE). Many advantages of MRI are compromised in the setting of SE by clinical emergencies delaying time-consuming scans—and they cannot usually be done at all during GCSE.
Many epileptogenic lesions can be detected by changes in signal intensities; fluid attenuated inversion recovery (FLAIR) and T2-weighted images have increased sensitivity for detecting such lesions. Some acute insults lead to restriction of water diffusion in the brain and are detected best with diffusion-weighted imaging (DWI) sequences, which provide information regarding the microstructure of the brain and diffusivity of water in the local environment. Some of these findings were evident in a patient with hippocampal sclerosis who presented in NCSE (Fig. 6.2).


Fig. 6.2
Magnetic resonance imaging (MRI) scan of an 18-year-old woman with a history of seizures presenting in nonconvulsive status epilepticus. Coronal fluid attenuated inversion recovery (FLAIR)-weighted images show high signal intensity in the right hippocampus (a). Coronal spoiled gradient echo images show atrophy of the right anterior hippocampus (b)
With MRI becoming a more accessible diagnostic tool across many sites, there are now several retrospective studies demonstrating regional and global abnormalities associated with convulsive SE [2], most commonly including restricted DWI signals, reduced T1-weighted signals, and sometimes, contrast enhancement [3–6]. Usually, at least one post-contrast imaging sequence should be obtained to detect contrast-enhancing lesions. There are fewer studies evaluating MRI changes in NCSE, but imaging findings are generally similar to those in convulsive SE. Bauer and colleagues reported T1-weighted changes and edema in the right frontal lobe in the patient who, at that point, had been in NCSE for 5 days [1]. In three patients with complex partial SE, Lansberg and colleagues demonstrated reversible cortical DWI and FLAIR abnormalities, similar to those seen in strokes, but not respecting vascular territories [7]. The abnormalities resolved, but some atrophy resulted in the same areas. Similarly, Chu and colleagues reported the case of a 52-year-old patient in NCSE whose MRI demonstrated marked cortical DWI hyperintensity throughout the brain, with an apparent diffusion coefficient (ADC) decreased in the corresponding areas [8].
Kawai and colleagues described a patient who presented in hypoglycemic coma with focal motor SE whose MRI showed multiple areas of signal hyperintensities broadly over the right hemisphere [9]. PET showed intense glucose hypermetabolism. After seizures ceased, the hyperintensities resolved, and atrophy was noted in some of those areas. Much of the hippocampal atrophy found on long-term follow-up MRI scans has been the result of prolonged convulsive SE rather than NCSE and often in children, with both febrile SE and SE from other causes [10].
Transient peri-ictal MRI abnormalities have been reported in up to 30% of patients presenting in SE. In one series, the abnormalities (mostly increased T2, FLAIR, and DWI signals) were markedly varied in location, both cortical and subcortical, and in many regions [11]. At long follow-up (mean 30 months) 63% of patients had persistent MRI abnormalities, including focal atrophy and cortical laminar necrosis, but some of these abnormalities may have been related to the underlying causes of the SE. In another report, 19 of 69 patients (28%) presenting in the emergency department with focal or generalized SE, almost always convulsive, had peri-ictal DWI restriction, usually cortical, and most with additional thalamic DWI restriction; all patients with DWI restriction had periodic lateralized epileptiform discharges on EEG and had intense, repetitive seizures [12].
In some patients, cortical (with or without subcortical) T2 and FLAIR hyperintensity may resolve after seizures are controlled. DWI changes described in patients with focal motor SE often resolve. In others, irreversible brain injury can occur. The acute signal changes seen with hypterintense T2 or FLAIR and restricted diffusion may resolve, but encephalomalacia or cortical laminar necrosis can result in other patients [2]. Predicting the evolution of those lesions induced by seizures remains difficult.
Positron Emission Tomography
PET typically utilizes 2-deoxy-2-(18F) fluoro-d-glucose (FDG) in the interictal state to demonstrate the hypometabolic activity of epileptogenic regions. PET lacks the anatomic resolution of MR but, as it is a functional metabolic study, it can be more sensitive in detecting potential epileptogenic areas. Furthermore, EEG can be recorded simultaneously with PET, improving its diagnostic potential. Unfortunately, the availability of PET is limited and it is very costly. A PET scan takes approximately an hour to complete, including the administration and uptake of the agent and scanning time. PET scanning is typically ventilator-compatible, but trained personnel must be present to monitor neurologic and medical stability during the procedure.
FDG-PET changes described in case reports during NCSE have been inconsistent. Most reports on PET during SE have been with focal SE and have shown regional hypermetabolism [13]. One report demonstrated focal, regional, or multiregional hypermetabolism, which may correspond to the localization of EEG ictal activity [14]. In a patient with cognitive deficits, van Paesschen and colleagues found parietal hypermetabolism on PET scan, supporting the diagnosis of focal NCSE, while nearby frontal hypometabolism corresponded to focal slowing on the EEG, in turn correlating well with additional clinical deficits [15]. In other reports, focal hypometabolism was seen in the presence of NCSE due to a destructive lesion [16]. Handforth and colleagues found an area of hypermetabolism on PET in a patient with periodic lateralized epileptiform discharges on EEG following a focal-onset convulsion and concluded that this was evidence of ongoing focal NCSE [17]. Given the paucity of systematic studies, the role of PET in the diagnosis or treatment of NCSE has not been defined.
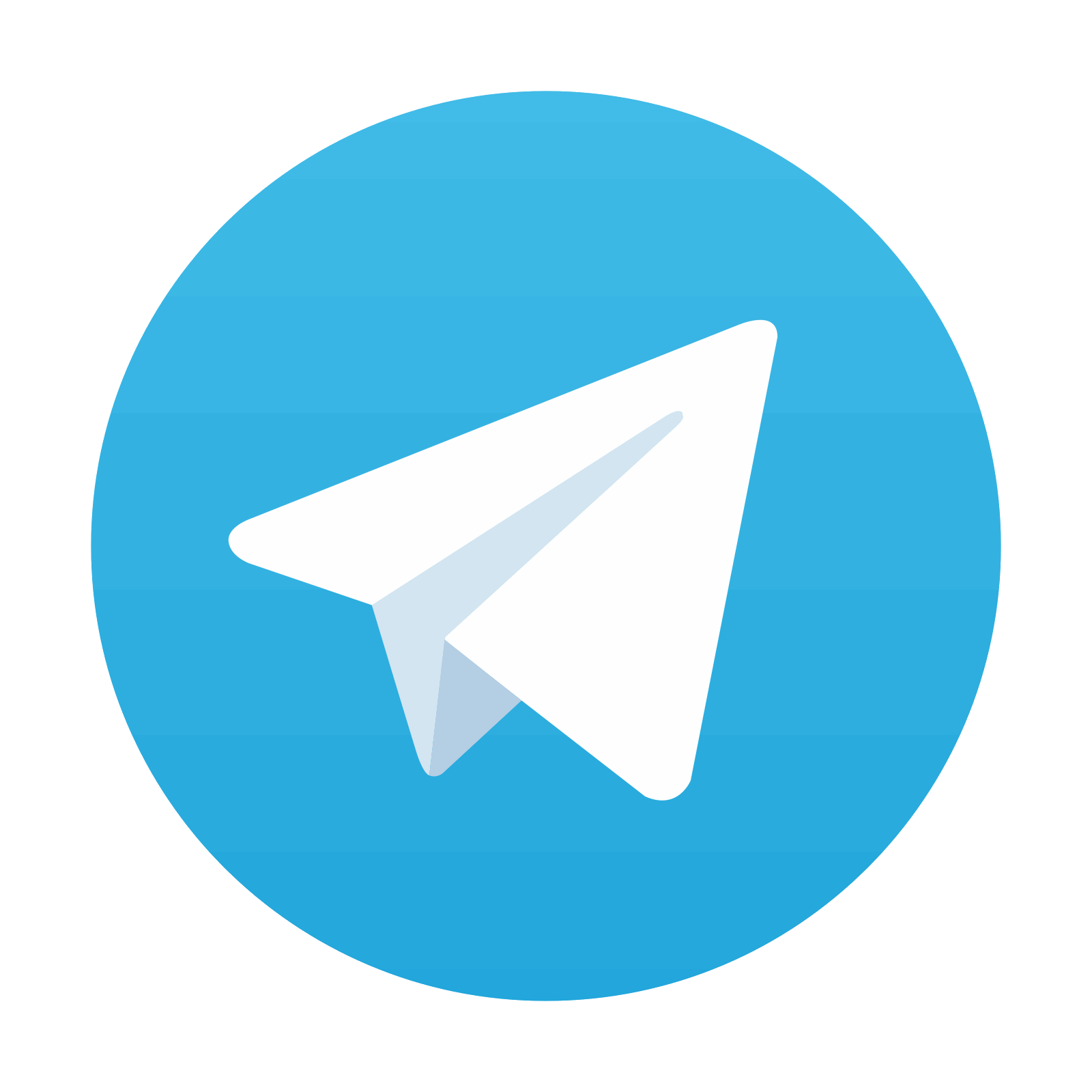
Stay updated, free articles. Join our Telegram channel

Full access? Get Clinical Tree
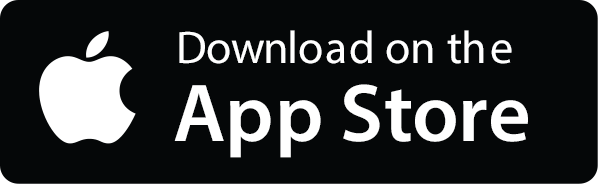
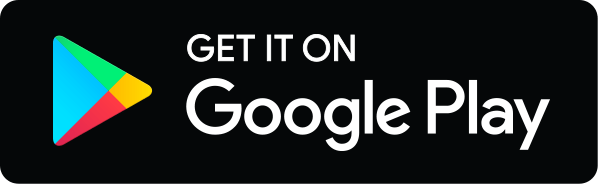