Multifocal enhancing lesions
Solitary enhancing lesion
Solid
Metastases
Multifocal glioma
Lymphoma
Demyelination
Granulomatous disease
High-grade glioma
Metastases
Lymphoma
Medullublastoma
Ependymoma
Cystic
Metastases
Infection (bacterial, fungal, parasitic)
High-grade glioma
Metastases
Hemangioblastoma
Pilocytic astrocytoma
Ependymoma
Calcification
Granulomatous disease
Metastases (squamous cell carcinoma, mucinous adenocarcinomas, osteosarcoma, chondrosarcoma)
Oligodendrogliomas
Gangliogliomas
Central neurocytomas
Hemorrhage
Metastases (melanoma, renal cell carcinoma, and thyroid carcinoma)
Fungal septic emboli
High-grade glioma
Metastasis
Pleomorphic xanthoastrocytoma
Tumor Permeability and Peritumoral Edema
Metastatic tumors to brain resemble the tissue characteristics of the primary tumors and therefore do not possess the blood–brain barrier seen in normal brain tissues. Thus, lesional enhancement is almost always observed for metastases on delayed post-contrast CT or T1-weighted MR images due to extra-vascular leakage of contrast agents. Aggressive primary intra-axial tumors such as higher grade astrocytomas, primitive neuroectodermal tumors, and primary CNS lymphomas also commonly enhance, although the mechanism of enhancement often include disruption of blood brain barrier and secretion of vasoactive substances. Other non-neoplastic intracranial processes can also exhibit contrast enhancement, including infarct, inflammation or infection.
One consequence of increased vascular permeability is accumulation of interstitial water content, resulting in decreased attenuation on CT as well as hyperintense signal on T2-weighted MRI from prolonged T2 relaxation times. A vasogenic pattern is characterized by edema that does not violate the boundary at the gray-white matter junction and can be easily identified by noting the exaggerated difference between gray and white matter attenuation or signal intensities on both CT and MRI. In contrast, cytotoxic edema resulted from direct damage to tissues as seen with ischemic infarction, does not spare the gray matter and there is a reduction in visual contrast at the gray-white matter junction. The extent of edema surrounding intra-axial metastases is often significantly greater than the actual tumor size. Rarely, vasogenic edema can be absent particularly with very small tumors (<1 cm).
When untreated brain metastases in the cortex near cerebral sulci or cerebellar folia are observed without associated edema, involvement of the extra-axial space, i.e. leptomeninges, should be suspected. Extra-axial metastatic tumors, similar to some meningiomas, can produce edema in the adjacent brain due to mass effect causing venous congestion or direct effect on capillary permeability.
Intratumoral Hemorrhage
Among intracranial metastases, hemorrhage more commonly occurs in melanoma, renal cell carcinoma, and thyroid carcinoma. Hemorrhagic metastases demonstrate variable signal intensities depending on the age of hemorrhage, and intratumoral hemorrhages tend to evolve more slowly and often contain blood product of mixed ages as compared to hemorrhages from non-tumor causes. Due to their intrinsic melanin content and frequently intratumoral hemorrhages, hyperintensity on unenhanced T1-weighted images can be observed in melanoma [5–7] (Figs. 2.1a–c and 2.2a–d).



Fig. 2.1
a–c Melanoma hemorrhage . Two melanoma metastases in the left temporal and occipital lobes demonstrate hyperintensity on T1-weighted image (a). Following contrast administration, subtle enhancement is seen along the margins of hemorrhage. Magnetic susceptibility within the masses confirm presence of hemorrhage (c)

Fig. 2.2
a–d Melanoma . 33-year-old female presented with history of melanoma presenting with rapid onset hearing loss. A nodular lesion in the left cerebellopontine angle is hyperintense on T1-weighted image (a) and hypointense on T2-weighted images (b). No magnetic susceptibility is seen associated with this lesion (c). Following contrast administration, an enhancing component is difficult to detect in the presence of underlying T1 hyperintensity (d)
Assessing whether parenchymal hemorrhage in brain is spontaneous or secondary to aneoplastic process is an important task in neuroimaging. Important clues to an underlying tumor include presence of nodular enhancement adjacent or within hematoma, incomplete hemosiderin ring, peritumoral edema more extensive than expected for the age of the hemorrhage, and delay in the expected evolution of signal characteristics in the hematoma. When these imaging signs are not present, short-term follow-up imaging may be required to confirm the nature of hemorrhage.
Venous infarcts can mimic a brain mass with enhancement, vasogenic edema, and hemorrhage. The site of sinus or cortical vein thrombosis can lead to venous infarct in corresponding venous drainage territory. Thus, recognizing common location of venous infarct corresponding to site of thrombosis can be helpful in making the diagnosis.
Tumor Necrosis and Tumor Associated Cysts
Necrosis is a common imaging feature in brain metastases but also commonly seen with glioblastomas as well as lymphoma in immunocompromised population. Necrosis in metastases can also commonly occur following chemo- or radiation treatment, occasionally accompanied by enlargement of overall tumor size requiring close imaging follow-up to determine durability of treatment response.
Cystic brain metastases are commonly found with tumors of gastrointestinal or genitourinary origins but also occur in lung cancers [8]. In contrast to necrosis, tumor associated cysts tend to have thinner borders. Other primary neoplasms, including hemangioblastoma, pilocytic astrocytoma, and ganglioglioma can contain cysts although these tumors are usually not associated with peritumoral edema. Unlike post-chemotherapy or radiation necrosis often observed during treatment response, enlargement of tumor associated cysts often indicates elevated tumor secretion and can be a sign of tumor growth.
The thin-wall cysts seen in some metastases can appear identical to non-neoplastic cystic brain lesions. It is important to consider infectious etiologies such as brain abscesses, which can appear as multifocal rim-enhancing lesions and exhibit surrounding vasogenic edema. Often patients with brain abscess(es) have a distinct clinical history as well as presenting signs and symptoms from those with brain metastases, although it can be challenging to distinguish them clinically on occasions. Diffusion-weighted imaging based on the detection of changes in the random motion of protons in water is particularly helpful in diagnosing brain abscesses since their mucopurulent content tends to have very low diffusivity and exhibits a dark signal on the apparent diffusion coefficient (ADC) maps. In contrast, brain metastases have peripheral low diffusivity due to higher cell density while the necrotic content centrally tends to be of high diffusivity (bright on ADC). However, presence of hemorrhage can affect diffusion characteristics and one should be careful when there is evidence of susceptibility changes within the area of diffusion signal abnormality. Compared to abscesses, metastases are much more likely to be associated with hemorrhage. Furthermore, a small group of metastases, usually from GI or GU origins, may present as mucin-containing cystic lesions that manifest as similarly low diffusivity. On the other hand, atypical infections such as toxoplasmosis may not show low diffusivity within the cyst content.
Calcifications
Intratumoral calcifications rarely observed among untreated brain metastases, although it has been reported in a variety of tumor types, including common tumor metastasizing to brain such as lung and breast cancers as well as more rare tumor types including squamous cell carcinoma, mucinous adenocarcinomas from genitourinary or gastrointestinal origins, osteosarchoma, and chondrosarcoma [9, 10]. On the other hand, calcifications frequently occur in many primary intracranial tumors, most notably oligodendrogliomas, gangliogliomas, neurocytomas, and craniopharyngiomas. Following treatment, calcifications can develop within metastases. CT is both sensitive and specific in detecting macroscopic calcification, and approximately 3% of brain metastases seen on CT exhibited calcification in one series [11]. On MRI, calcifications can result in variable signal intensities depending on the microenvironment of calcium, but most commonly they appear as focal T1 shortening and magnetic susceptibility. These MR imaging findings, however, overlap with iron within blood products although they can be distinguished by differences in phase shifts [12].
Extra-Axial Intracranial Metastases
Within the extra-axial compartment metastases can involve bony structures of calvarium and skull base, dura (pachymeninges), leptomeningeal spaces including cerebral sulci, cerebellar folia, and cranial nerves, or within ventricles. Similar to osseous metastatic tumor elsewhere, metastases to calvarium and skull base can have lytic, sclerotic, or mixed patterns on CT depending on primary tumor subtypes. For example, prostate cancer metastases are generally sclerotic, whereas renal and thyroid metastases tend to present as lytic lesions. Following treatment, lytic metastases frequently produce a sclerotic pattern.
Dural (pachymeningeal) metastases often grow from adjacent calvarium or skull base but can be directly seeded from hematogeneous sources. Breast cancer, lymphoma, leukemia, prostate cancer, and neuroblastoma are tumors most commonly presenting as dural metastases (Fig. 2.3a–c). Meningiomas are the most common benign tumor arising from dura, and it can be difficult to distinguish from dural metastases unless meningiomas contain calcifications or induce hyperostosis of adjacent bone. Intracranial hypotension is a common non-neoplastic cause of dural enhancement but the pattern of enhancement is almost always diffuse and smooth rather than focal or nodular, and is often supported by clinical history of previous cranial or spinal intervention. In severe cases, intracranial hypotension can be associated with subdural hygroma or hematoma, and it is also not uncommon for dural metastases to produce subdural fluid collection or hemorrhage. Contrast-enhanced MRI is best for detecting nodular enhancement along the dural lining of hematomato diagnose dural-based tumor (Fig. 2.3a–c).


Fig. 2.3
a–c 70-year-old man with metastatic prostate cancer presents with headache. T1-weighted unenhanced image shows a T1 hyperintense collection along right frontal convexity (a) consistent with subacute subdural hematoma. Following intravenous contrast administration, extensive dural thickening with multifocal enhancing masses are identified (b and c), evening along the lining of subdural hematoma (b)
Leptomeningeal metastases occur in many types of cancer, particularly breast carcinoma, lung carcinoma, melanoma, lymphoma, and leukemia. Metastatic spread to the meninges can occur hematogenously via meningeal vessels or through direct extension from metastatic lesions at the pial surface. Leptomeningeal metastases are usually not detectable on CT without contrast enhancement, although hydrocephalus can be an indirect sign of leptomeningeal involvement. Contrast-enhanced MRI provides significant better sensitivity in detecting leptomeningeal metastases as planar or nodular enhancement along cerebral sulci, cerebellar folia, or cranial nerves [13] (Fig. 2.4a–c). Other non-tumor processes including ischemic infarction and leptomeningitis can also exhibit enhancement along brain surfaces but often can be distinguished based on clinical history. A nodular pattern of leptomeningeal enhancement is more commonly seen with neoplastic processes but can also be observed in infectious etiologies. CSF analysis should be obtained when leptomeningeal abnormality is identified on imaging but there is uncertainty regarding the diagnosis. On fluid-attenuated inversion recovery (FLAIR) sequence , leptomeningeal tumor can appear as hyperintense signal along sulci as a result of shortened T1 relaxation time but this finding without enhancement is not specific since other CSF pathologies such as hemorrhage or infection can appear identical. Unlike parenchymal metastases, leptomeningeal seeding of tumor commonly does not lead to significant vasogenic edema, although edema and mass effect can be observed when there is florid involvement. Table 2.2 summarizes the main differential diagnoses of extra-axial masses.


Fig. 2.4
a–c Leptomeningeal . 55-year-old man with history of lung cancer presents with acute headache and gait disturbance. Axial T1-weighted post-contrast images demonstrate leptomeningeal carcinomatosis with enhancement along cerebral sulci (a), cerebellar folia (b), and cranial nerve in the internal acoustic canals (c)
Table 2.2
Differential diagnosis for extra-axial mass (es)
Intraosseous-calvarium | Leptomeningeal | Pachymeningeal |
---|---|---|
Hemangioma Fibro-osseous lesions Metastasis Langerhans cell histiocytosis Sarcoma Meningioma | Metastases Infectious meningitis Lymphoma Sarcoidosis PNET | Meningioma Metastases Lymphoma Sarcoidosis Idiopathic pachymeningitis |
General Approach to Imaging of Patients Presenting with Suspected Brain Metastases
When one or more enhancing mass is found on brain imaging of a patient with known progressive or recurrent metastatic cancer, diagnostic certainty for brain metastasis is high. For patients with no or remote history of cancer presenting with newly discovered brain mass(es), the diagnostic possibilities can be broad, including primary brain neoplasms, infection, demyelinating lesions, vascular lesions that can be difficult to distinguish from brain metastasis on both imaging and clinical presentation.
While multiplicity can be helpful in making the diagnosis, solitary metastases are common and can be difficult to distinguish from other intra-axial tumors such as primary glioma or lymphoma, as well as non-neoplastic mass-like processes such as demyelination, infection, or vascular pathology. When multifocal enhancing brain metastases occur, the extent of T2 signal abnormality surrounding lesions tend to be separated on T2-weighted imaging, whereas multifocal enhancement in high-grade gliomas typically show contiguous areas of infiltrative appearing nonenhancing T2 abnormalities between foci of enhancement. In addition, the areas of T2 abnormality in high-grade glioma often demonstrate infiltration of gray matter.
Demyelination disease can be “tumefactive,” mimicking a brain mass clinically and on imaging. These lesions, however, tend to occur in younger patients without history of malignancy. On imaging, an “incomplete ring sign” is an important feature suggesting the presence of a demyelinating lesion. CSF analysis for gamma globulin can help clarify the diagnosis, and follow-up imaging several months later often confirms eventual improvement or resolution.
Vascular lesions including arterial or venous infarction as well as vascular malformations can mimic a brain mass. Ischemic infarcts, particular during subacute phase, can present as enhancing lesions 2–3 days following onset of the ischemic event that persist for several months; diffusion signal changes typically associated with acute infarct usually normalize after 7–10 days [14]. Enhancement associated with ischemic infarct, however, does not exhibit surrounding vasogenic edema. For small enhancing lesions along gray matter that cannot be diagnosed as infarction based on clinical history or lesion location, a short-term follow-up imaging can readily clarify the etiology. It is important to keep in mind that ischemic infarct can coexist with brain metastasis due to a hypercoagulable state associated with malignancy, as well as from embolic events from systemic procedures. In addition, perioperative infarct is not infrequently observed and often located immediately adjacent to the surgical margin. It is important to perform and examine the diffusion- weighted sequence during the immediate postoperative MRI to identify area(s) of peritumoral infarct so the expected subsequent enhancement will not be misinterpreted as tumor recurrence.
When brain imaging reveals findings suggestive of metastatic disease in a patient without history of systemic cancer, imaging for potential primary cancer site or additional extracranial metastases is often necessary to identify an anatomical site that carries a lower risk for diagnostic biopsy. The imaging modalities depend on the patient demographics, but often include CT scan of the chest, abdomen and pelvis. FDG-PET imaging can be highly sensitive in detecting occult primary malignancy and can be used as part of staging in many systematic cancers.
Optimization of Radiological Protocol for Evaluation of Intracranial Metastasis
For staging systemic malignancy in an asymptomatic patient, CT can be the test of choice due to its wider availability and the speed of imaging. While small brain metastases can be difficult to detect by CT unless there is significant surrounding edema, mass effect or intratumoral hemorrhage, CT has the advantage of visualizing lesions that are centered in bone or producing calcifications. CT imaging of the bony margins can help elucidate the underlying pathology. For example, slow-growing lesions are often accompanied by bone remodeling and manifesting as smooth cortical rather than destructive margins. Calcifications are often found in a subset of brain tumors, including meningioma, oligodendroglioma, germ cell neoplasm, and craniopharyngioma, and these are better visualized by CT evaluation. CT may be the only available option for screening if patients have contraindications for MRI such as cardiac pacers, ferromagnetic foreign bodies, implants or surgical devices. While whole-body 18fluoro-D-glucose positron emission tomography (FDG-PET) is commonly used for initial stating of systemic cancers, its sensitivity in detecting brain metastases is generally considered low due to relative high FDG uptake in normal brain tissues. For example, the sensitivity of brain PET/CT for detecting brain metastases in lung cancer was 72% in one series [15].
For most systemic malignancy, MRI is the imaging modality of choice in assessing brain metastases due to its excellent soft tissue contrast and lack of ionizing radiation. Several MRI sequences are generally recommended during evaluation of brain metastases. An isotropic volumetric post-gadolinium T1-weighted sequence allows detection of very small lesions, on the order of 1–2 mm. This high sensitivity can be important since the total number as well as the distribution of metastatic brain lesions contributes to selection of treatment approach. The sensitivity of detecting brain metastasis can be improved by increased time duration following contrast and using contrast agents with longer T1 relaxation time [16–18]. Higher magnet strengths, i.e., 3 versus 1.5 T, can further increase sensitivity [19, 20]. In addition, volumetric acquisition allows multi-planar reformation for better lesion visualization and can be fused with stereotactic equipment for surgical guidance. This type of acquisition method requires longer acquisition time and is therefore more susceptible to patient motion artifact when performed using lower field strength MR scanners. Finally, it is often helpful to perform a T1-weighted sequence before contrast administration to distinguish true enhancement from other tissue types with short T1 relaxation times such as hemorrhage, fat or proteinaceous cyst.
Mass lesions with a cystic component as well as perilesional edema can be readily delineated by a T2-weighted sequence. Infiltration of tissues with prolonged T2 relaxation time in the cortical gray matter with expansion of gray matter and blurring of the gray-white matter junction is a specific sign of primary glioma and not observed with metastatic tumors. On T2-weighted imaging, the interface of tumor and adjacent brain often appears sharper compared to high-grade gliomas.
Diffusion weighted imaging (DWI) is commonly included during evaluation of brain lesions due to its high sensitivity in detecting a variety of brain pathologies including infarct, abscess, cerebritis, and tumor that manifest as hyperintensity on DWI and hypointensity on apparent diffusion coefficient (ADC) maps that are calculated from DWI. Findings on DWI images are more helpful when they are interpreted alongside conventional imaging sequences and clinical history for improved specificity. For example, subacute infarct can manifest as cortical swelling and enhancement mimicking brain tumor, but a serpiginous pattern of low diffusivity confined to an arterial distribution is characteristic of ischemic infarct rather than neoplasm. When a peripherally enhancing brain lesion is encountered, evidence of low diffusivity within the lesion is suggestive of abscess as discussed previously but the diagnostic specificity can be increased if the enhancing rim is also thin and systemic signs of infection are present.
On MR imaging, DWI has also been correlated with hypercellularity typically manifesting as reduced diffusivity on the ADC map [21]. This imaging marker of cell density can be used to help several tumor types that are characteristically hypercellular, including breast and lung metatases [22]. CNS lymphomas are characteristically cellular [21, 23] and are more commonly located in the periventricular regions of brain. It is important to recognize that intratumoral hemorrhage can appear as hyperdensity on CT, and ADC measurement can also be decreased due to presence of blood product. DWI is also very useful in identifying calvarial and skullbase metastases due to its high lesion to background contrast [24] (Fig. 2.5a, b).


Fig. 2.5
a–b DWI-Calvarium-Orbit. 46-year-old female with breast cancer developed metastases to calvarium and right orbit. The lesions are seen on both T1-weighted imaging (a) than DWI (b), although more conspicuous on the latter due to greater lesion to background contrast
T2*-sensitive techniques such as gradient-echo and susceptibility-weighted imaging are useful for detecting intratumoral hemorrhage, which is common in high-grade gliomas and metastases, but rare in low-grade gliomas and lymphoma. The deoxyhemoglobin causes magnetic susceptibility that results in shortened T2* relaxation times and hypointense signal changes on T2*-weighted (T2*W) images. This phenomenon can improve sensitivity of detecting small metastatic lesions with hemorrhages that do not enhance or elicit peritumoral edema. For example, 7% of melanoma lesions were detected only on this sequence in one series [5]. Susceptibility-weighted imaging (SWI) can amplify the effect of magnetic susceptibility by overlaying magnetic phase changes with high-resolution magnitude images to detect foci of microhemorrhages [25]. These techniques are also helpful in identifying thromboses within dural venous sinuses or cortical veins due to exaggerated magnetic susceptibility from deoxyhemaglobin [26]. The appearance of venous infarction on conventional MRI sequences can mimic brain tumor due to mass effect, edema, and enhancement. If misdiagnosed, this can lead to unnecessary biopsy or a delay in anticoagulation treatment.
Advanced Techniques in Brain Metastasis Imaging
Several imaging techniques have become increasingly available for evaluation of brain lesions including MR spectroscopy, MR perfusion, and MR diffusion tensor imaging. When combined with conventional imaging sequences, these advanced methods can often provide useful diagnostic information when evaluating brain lesions including metastases. As these advanced imaging methods are being improved and validated, it is important to understand their advantages and limitations before incorporating them into clinical imaging protocol.
Magnetic Resonance Spectroscopy (MRS)
MR spectroscopy can provide a noninvasive window to analyze metabolic composition of brain lesions. Similar to most pathological processes observed in brain, metastases show reduced NAA peak on MR spectroscopy [27, 28], and the level of NAA concentration is often absent or much lower than other intrinsic brain pathologies where brain tissues are being infiltrated or damaged as in case of primary brain tumor or inflammation. Choline concentration also typically increases in the enhancing portion of metastases as a result of increased cellular turnover [27, 29]. This metabolic marker can be useful in distinguishing peritumoral edema surrounding metastases from infiltrative glioma since reactive or vasogenic edema, unlike tumor infiltration, does not result in greater cellular turnover [30]. Lipids and lactate are also frequently seen in metastases although these are not specific findings and can be found also in high-grade gliomas [30]. Based on a retrospective analysis of multicenter trial data, automatic brain tumor classification by MRS allows classifications of glioblastoma, meningioma, metastasis, and low-grade glial with diagnostic accuracies of near 90% for most diagnostic pairs except for the glioblastoma versus metastasis discrimination [31].
Perfusion-Weighted Imaging (PWI)
Similar to high-grade primary brain neoplasms, metastatic lesions induce neovascularization resulting in greater intravascular blood volume, although the vessels associated with metastases are highly permeable similar to those of the primary systemic tumors [32]. Estimation of intravascular volume in tumors can be performed by dynamic imaging methods such as dynamic contrast-enhanced MRI (DCE-MRI) , dynamic susceptibility contrast MRI (DSC-MRI) , as well as dynamic contrast-enhanced CT. Due to its short acquisition time of less than 2 min, DSC-MRI can readily be incorporated into standard imaging protocol for evaluation of brain mass. Elevation of regional cerebral blood volume (rCBV) is characteristic of most high-grade gliomas, although metastatic tumors, particularly renal cell carcinoma, choriocarcinoma, and thyroid carcinoma, can also demonstrate high intravascular blood volume making this diagnostic imaging marker less useful [33]. The specificity of DSC-MRI can be improved by using spin-echo (SE) acquisition compared to gradient-echo (GRE) since the former technique appears to correlate with microscopic angiogenesis that is typical with gliomas, while the later technique detects macroscopic intratumoral vessels more commonly seen in cases of hypervascular metastases [34]. Furthermore, higher rCBV within the peritumoral region is also suggestive of infiltrative tumor rather than vasogenic edema [35, 36].
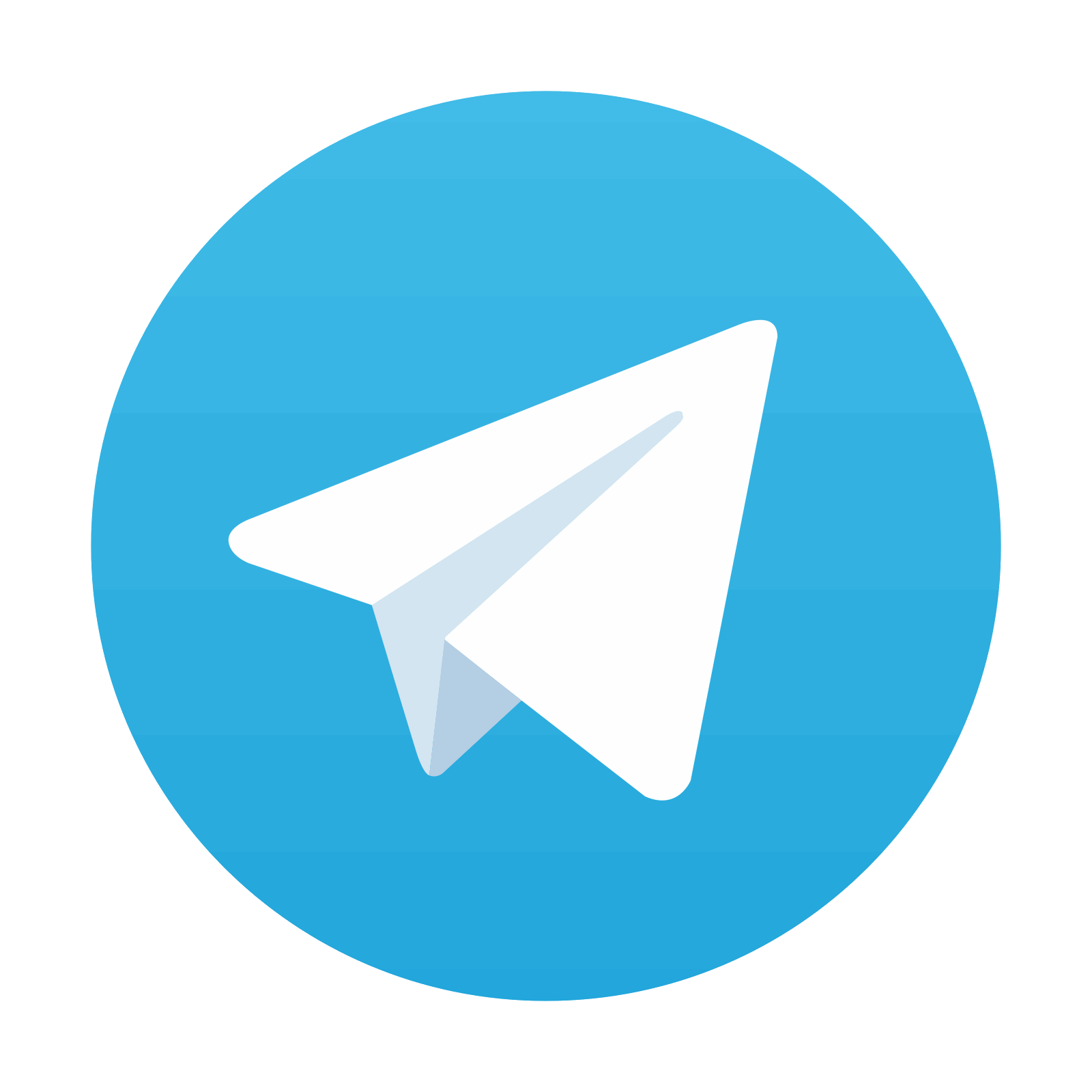
Stay updated, free articles. Join our Telegram channel

Full access? Get Clinical Tree
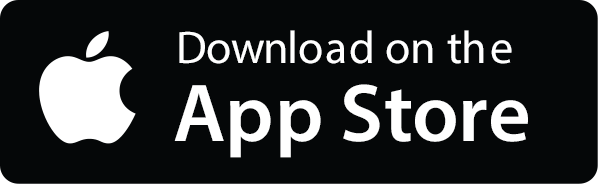
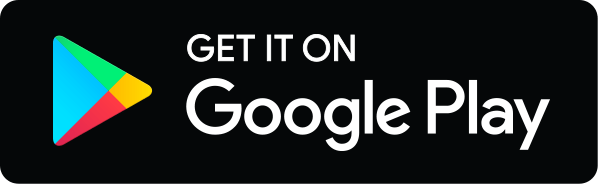