
feature of aging. The etiology for this observation may be related to a decrease in volume of subcortical structures rather than to a change in width of the cortex (59). Miller et al. (61) reported that the ratio of gray matter versus white matter was 1.13 at age 20, 1.28 at age 50, and 1.55 at age 100, which suggests that white matter atrophy exceeds that of gray matter. Most authors suggest that physiologic atrophy commences at the age of 50. However, CT studies on postmortem and living patients do show a wide variation in the CSF space size, ranging between 30% and 50% of the size of healthy young adults. Associated widening of the interhemispheric fissure, extending posteriorly to or beyond the callosomarginal sulci, has also been observed. Progressive widening of the sulci occurs in the frontal lobes and cerebellar vermis, beginning in the teenaged years (13). Widening of the superficial sulci seen with normal aging is often termed cortical atrophy by radiologists; however, in fact, this should more correctly be referred to as gyral or superficial atrophy. Sulcal enlargement in aging is diffuse; however, changes are best identified in specific locations after 50 years of age (12). Widening of the superficial cortical sulci is seen first in the frontal and parietal parasagittal regions (81). The anterior interhemispheric fissure and the CSF spaces around the cerebellar vermis also widen with age (37). The sulci around the central, precentral, postcentral, and superior frontal gyri widen later (49,82).
Table 3-1. Computed Tomography Ratios | ||||||||||||||||||||
---|---|---|---|---|---|---|---|---|---|---|---|---|---|---|---|---|---|---|---|---|
|
and an increase in the free radical formation (2). Iron is also deposited in the walls of blood vessels. Iron is more easily visualized on T2-weighted images as focal regions of low signal secondary to field heterogeneity and magnetic susceptibility (25).
Table 3-2. Magnetic Resonance Changes in Aging | ||||||||||||||||||||||||||||||||||||||
---|---|---|---|---|---|---|---|---|---|---|---|---|---|---|---|---|---|---|---|---|---|---|---|---|---|---|---|---|---|---|---|---|---|---|---|---|---|---|
|
have been inconsistent. A number of investigators have described diminished regional glucose metabolism (CMRGlc) in the temporal, parietal, somatosensory, and, especially, frontal regions. Others have described more prominent decreases in the frontal and somatosensory cortices in comparison with younger controls (15,51,87). When brain atrophy was not considered, mean CMRGlc values were lower in older patients, particularly in the frontal, parietal, and temporal regions. Also, women had significantly higher mean CMRGlc than men. Cerebrovascular risk factors in the population were also seen not to have any effect on CMRGlc (87).
of the temporal lobes can be performed and compared with normative data published by Bhatia et al. (7). In patients with AD, volumetric measurements reveal significantly lower measurements compared with a group of controls (47). A more recent study by Cuenod et al. (19) has shown that atrophy of the amygdala can occur earlier than hippocampal atrophy.
Table 3-3. Value of Imaging Techniques in Alzheimer’s Disease | ||||||||||||
---|---|---|---|---|---|---|---|---|---|---|---|---|
|
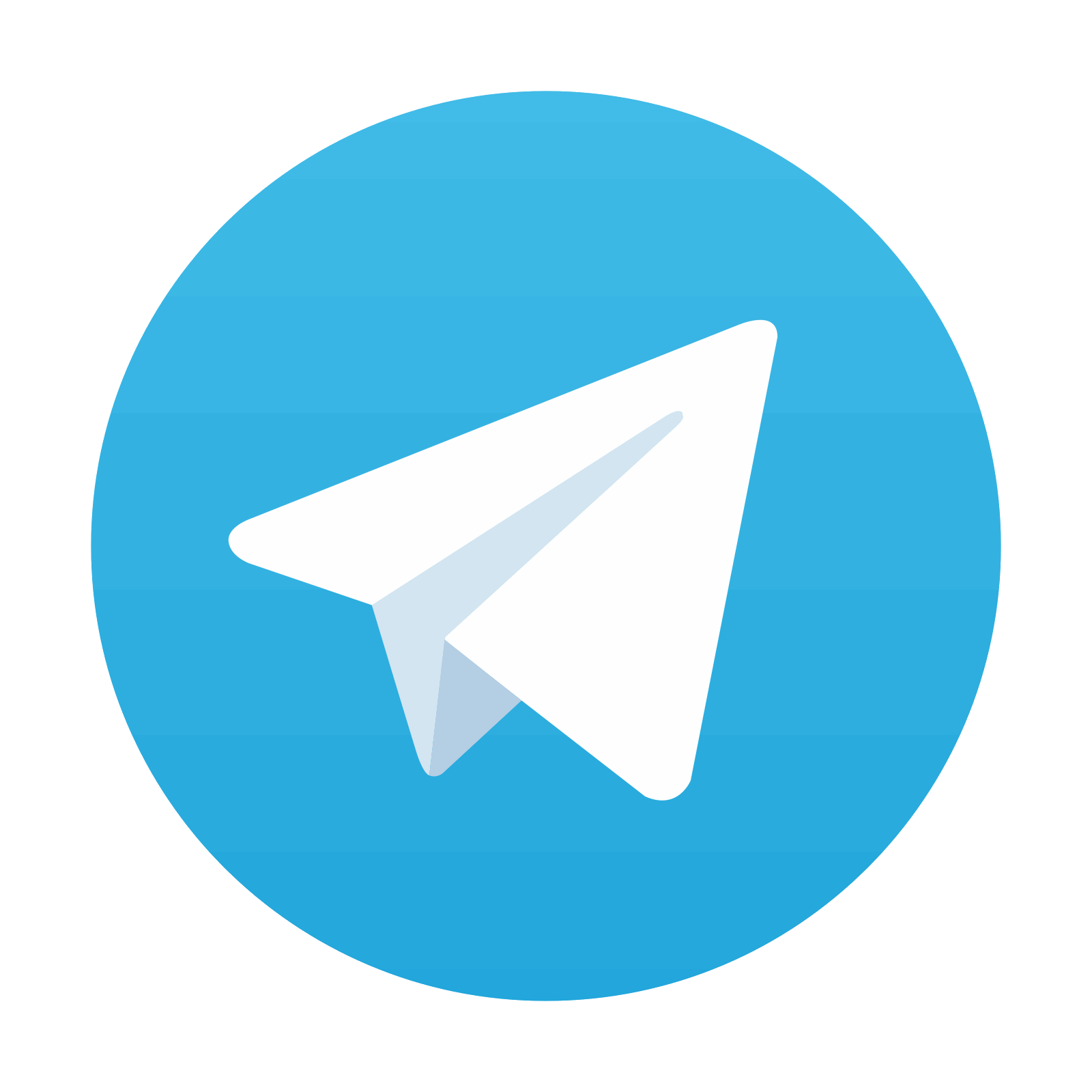
Stay updated, free articles. Join our Telegram channel

Full access? Get Clinical Tree
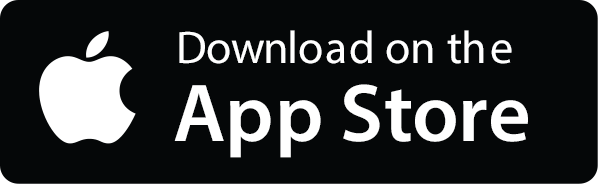
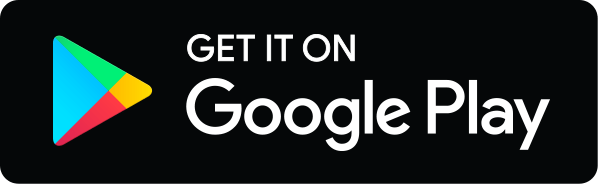
