Fig. 31.1
MR anatomy of the brainstem. (a) Midline sagittal T2. Pontomesencephalic and pontomedullary sulci (black arrows). Limits of the basis pontis (white arrowheads), with the tegmentum pontis behind. Caudalmost limit of the fourth ventricle marking the lower limit of the “open” medulla (white arrow). The “closed” medulla extends from there to the plane of the foramen magnum. (b) Midline sagittal T1. Same landmarks. (c) Coronal T2. It shows the three cerebellar peduncles: superior (small arrowheads), middle (large arrowheads), and inferior (arrows). (d) Axial T2 through the “closed” medulla with the pyramids ventrally. (e) Axial T2 through the “open” medulla with the inferior olivary nuclei anterolaterally, the inferior cerebellar peduncles posterolaterally, and the lower fourth ventricle dorsally. (f) Axial T2 through the pontomedullary sulcus, showing the lateral medullary fossa (white arrow). (g) Axial T2 through the pons showing the basis pontis anteriorly, the tegmentum pontis posteriorly (white arrowheads) with the median sulcus (black arrow), and the facial colliculi on either side. (h) Axial T2 through the cerebral peduncles with the superior cerebellar peduncles. (i). Axial T2 through the superior midbrain with the cerebral peduncles, the tegmentum mesencephali, the aqueduct (flow void), and the tectal plate dorsally
Coronal cuts (Fig. 31.1c) are useful to show the whole extent of the brainstem from the thalami to the cord, and especially to demonstrate the three cerebellar peduncles. However, the most useful plane beside the sagittal one is the axial plane, with cuts spanning the whole neuraxis from the upper cord (C2 or C3) to the thalami (Fig. 31.1d–i). The upper cord presents a transverse oblong appearance. The caudal medulla presents with the anterior bilateral protrusion of the pyramidal decussation (giving it kind of a triangular shape) and a tiny ependymal canal. The cut through the mid-portion of the rostral medulla shows the anterolateral prominence of the inferior olivary nuclei with the posterior widening of the lower third ventricle. One step above, the cut includes the pontomedullary sulcus with the lateral recesses, the inferior cerebellar peduncles, and the emergences of the facial and acoustic nerves. A cut through the caudal pons beautifully demonstrates the pons itself (transverse “bridge”) with the middle cerebellar peduncles, the tegmentum pontis posteriorly, as well as the ventricular floor with its median sulcus and medial eminences (colliculi faciales); a more rostral cut crosses the rostral pons anteriorly but the tegmentum mesencephali posteriorly (due to the obliquity of the midbrain-pons interface) as well as the superior cerebellar peduncles. Finally the last two axial cuts demonstrate the aqueduct and periaqueductal gray matter, the inferior and superior colliculi, the red nuclei and substantia nigra, and the diverging cerebral peduncles forming the junction with the base of the cerebral hemispheres.
31.2 Radiological Features of Brainstem Tumors
Old imaging modalities such as pneumoencephalography could not assess the parenchyma itself. Angiography could demonstrate a global swelling of the brainstem, sometimes some abnormal vascularity, and the frequent encasement of the basilar artery by the anterior exophytic portions of the tumor. Because no surgery was performed, and no biopsy, all brainstem tumors were categorized as a single group with a uniformly poor prognosis. CT for the first time allowed a parenchymal evaluation which, beside morphology, provided information on the structure of the mass (solid/cystic/edematous/hemorrhagic) and on its vascularity and blood-brain-barrier status. It was only after clinical experience with CT had accumulated that it became apparent that brainstem tumors could be subdivided in very different specific entities [1, 5]. This obviously could only be amplified by the generalized use of MR imaging after 1985, which resulted in a complete metamorphosis of the way brainstem tumors are seen, from both the anatomical and the structural points of view.
31.2.1 The Imaging Tools
MR imaging is an extremely versatile modality. The information it provides can be described as depending on relaxation imaging (classical T1- and T2-weighted imaging with its specific variants), proton diffusion imaging (diffusion-weighted and diffusion tensor imaging), proton metabolic imaging (MR spectroscopy, chemical shift imaging), and vascular and blood–brain-barrier imaging. In clinical settings, MR technology investigates protons only, whose behavior when excited by appropriate radio waves depends on their particular immediate physicochemical environment intrinsic (e.g., proteins) or extrinsic (e.g., gadolinium compound).
Relaxation imaging comprises the classical T1, T2, FLAIR, and T2*/susceptibility imaging.
T1 imaging, whatever the specific sequence design used, demonstrates the anatomy nicely, with a good gray-white contrast. The CSF is black. The gray matter depending on its organization is of intermediate signal and the white matter is bright. This is primarily due to myelin, or rather to the components of myelin which shorten the T1 relaxation time of the protons (magnetization transfer effect). This occurs quite early in the development because of the accumulation of myelin precursors, the more so in the brainstem as myelination proceeds from the cord to the forebrain. The “mature” T1 pattern is present early in the brainstem, about the end of the first semester. Whatever process changes the myelin content (edema, necrosis, gliosis) decreases the signal as well (it becomes dark). On the contrary gadolinium exerts a strong magnetization effect and induces a very bright signal.
T2 imaging also is good for anatomy, especially the anatomy of the brainstem nuclei. The CSF is white. The gray matter is of variable degrees of intermediate density, depending on the cellularity, the amount of myelinated fibers, and the amount of iron deposition. The white matter is dark (short T2 relaxation time), not as much as a direct effect of myelin but rather because the accumulation of compacted myelin, which has a weak T2 signal, correlates with a decrease of water content. The “mature” pattern on T2 imaging is present in the brainstem about the end of the first year (even though myelination is not completed until the end of adolescence). Whatever increases the relative amount of water in the tissue (edema, or lack, loss or replacement of myelin) appears as a bright signal. T2 imaging is insensitive to gadolinium. T2 spin-echo (or fast spin-echo) sequences are devised to neutralize local magnetic susceptibility artifacts induced, for example, by iron (notably blood or blood residue), air-bone interfaces, etc.
In susceptibility-weighted sequences (SWI, T2*GE, etc.) on the contrary, artifacts related to local magnetic field heterogeneities are maintained and even enhanced, in order to better demonstrate tiny foci of hemorrhage often associated with malignancy, blood residues (e.g., hemosiderin in macrophages) [9, 20, 21], calcium, metallic fragments, etc. Specific sequence design may also demonstrate the intraparenchymal blood-containing venous channels nicely [22].
T2–FLAIR sequences are true T2 sequences in which the signal of the free water (normally bright) is specifically cancelled (appears black) by an appropriate radiofrequency pulse. This allows differentiating the bound water associated with cellular membranes, proteins, or even gadolinium (remains bright) from the CSF or CSF-like (e.g., interstitial fluid) collections (blackened). The interstitial water is suppressed in the neonatal brain (white matter is dark) because there is not enough myelin to bind it. Yet after a few weeks, the accumulation of myelin precursors increases the amount of bound water and reduces that of free water, and the white matter becomes bright. Thereafter, the signal proceeds as with conventional T2 sequences, except that the mature pattern is not reached until later than on classical T2 sequences. T2-FLAIR sequences are optimal to appreciate structural changes and to recognize faintly bloody or hyperproteinic CSF, cystic or interstitial fluids. On 3 T magnets, the iron-related hyposignal in the globi pallidi and the red nuclei often is especially prominent.
T2 steady-state sequences (CISS/FIESTA) produce 3D-T2 submillimetric images with an outstanding morphological definition but poor demonstration of the intrinsic parenchymal structures. They are therefore of limited use in this type of pathology except for the demonstration of an aqueductal obstruction and, in the follow-up of brainstem tumor patients with hydrocephalus, demonstration of the patency of a third ventriculostomy.
Diffusion imaging uses the Brownian motion of the protons at the body temperature. It comprises the classical diffusion-weighted imaging (DWI/ADC) and the more quantitative diffusion tensor imaging (DTI). Totally independent from the relaxation parameters T1 and T2, it is based on the fact that with optimized sequences (defined by the factor b, typically b1000), the range of diffusion of the protons in a living tissue at 37 °C is of the same order as the size of the extracellular space.
DWI/ADC mapping is intended to reveal restriction of the proton motion mostly in the extracellular spaces. The freer the protons are to move (e.g., in the ventricles), the more de-phased they become and the weaker the returned signal is (CSF is black). The more restricted their motion is by the surrounding cells, the more coherent and stronger their returned signal remains (the lesion is bright). DWI therefore has the ability to detect a restricted diffusion, due to a decrease of the extracellular spaces, but it cannot appreciate an increased diffusion. The calculated correlate of the restricted diffusion imaging is the ADC mapping (apparent diffusion coefficient) where areas of free motion appear bright, while areas of restricted motion appear dark. In tumor pathology, restricted diffusion may be demonstrated when a dense cellularity restricts the extracellular spaces.
DTI goes one step further as it has the capability to quantify the diffusivity: therefore, it can evaluate both decreased and increased diffusions, that is, both restricted and expanded extracellular space. In a fascicular structure such as the white matter, diffusivity parameters can be appreciated three-dimensionally according to the direction of the fibers: axial diffusivity is parallel to the fibers and radial diffusivities are perpendicular to the fibers; they are measured in mm2/s. The directionality of the structure can be expressed by a fractional anisotropy index (FA) which may vary from zero (the motion of the proton is the same in every directions, like in the ventricles) to one (motion is in one direction only). In normal fascicular white matter, intermediate FA values are obtained as the diffusivity is more along the fibers than perpendicular to them. With appropriate post-processing it is possible to use FA mapping to represent the anatomical organization of the white matter fascicles (fiber tracking). Decreased FA may reflect a decrease of axial diffusivity (altered fascicular organization, hypercellularity) or an increase of radial diffusivity (loss of fibers and/or myelin, extracellular edema) or both. In brainstem glioma, fiber tracking is used to help differentiate infiltrative tumors from compact tumors and from necrosis and tumors from inflammation [7, 23–25].
Metabolic imaging includes MR spectroscopy (typically monovoxel) and metabolic cartography with chemical shift imaging (CSI).
Proton MR spectroscopy ( 1 HMRS) has been included in many routine MR protocols for about two decades. The measurement is usually done in a single voxel of approximately 2 by 2 by 2 cm selected in the region of interest. Given its overwhelming abundance, the signal of the free water has to be cancelled by specific radiofrequencies. MRS is based on the fact that the resonant frequency of the excited proton depends on its molecular environment: this phenomenon is called the chemical shift. Different proton-containing molecules may be differentiated, thanks to their specific molecular arrangements, resulting in separate peaks on the spectrum (the shift is measured in parts per million or ppm), and their relative amount is reflected by the relative size/area of the peaks. For tumors, MR spectra obtained with a long echo time provide most of the information needed in a reasonably short time: lactate (energy failure, necrosis)) at 1.33 ppm, NAA (neuroaxonal activity mostly) at 2.01 ppm, creatine (energetic metabolism) at 3.02 (more or less invariant in the neural tissue), and choline (cellular turnover) at 3.22 ppm. By using a short echo time, more metabolites can be identified such as the macromolecules (necrosis) at 0.9–1.3 ppm, myoinositol (a marker of the glia) at 3.56 ppm, or taurine (a marker of embryonal tumors) at 3.35 ppm. Absolute metabolite concentrations may be extrapolated also [6, 26–28].
Instead of investigating a single voxel, CSI investigates a plane or even a volume, assessing the core of the tumor as well as its margins and the surrounding normal tissue [28]. Given the small size of the brainstem, however, and the proximity of the bony structures with the related distortion of the magnetic field, it is usually not the procedure of choice in brainstem tumors.
Perfusion imaging provides information on focal relative cerebral blood volume (CBV), transit time (TT), and relative cerebral blood flow (CBF). Therefore, it has the potential of being useful to differentiate benign from malignant tumors as well as radiation necrosis from tumors. It may also be helpful to appreciate the effects of therapy when antiangiogenetic drugs are used. Perfusion data are obtained by analyzing the curve of a single-pass bolus of gadolinium contrast (dynamic susceptibility-weighted contrast-enhanced perfusion imaging DSC). It may also be done by labeling the incoming arterial blood with a specific inversion pulse (arterial spin labeling ASL) [29]. When performed by using a bolus of gadolinium compound, it may also be used to evaluate the vascular permeability and to document the degree of impairment of the blood-brain barrier: after the first pass the curve remains above base level because of the accumulation of contrast into the tissue due to the leaking abnormal or impaired BBB [30]. However, perfusion studies are difficult to perform in a small structure like the brainstem that is surrounded by so many artifact-producing structures; they are not routinely used [31].
31.2.2 Imaging Protocols
High-field clinical magnets (3.0 T) and multiple array coils in general yield images with good definition. The location in the posterior fossa however is typically associated with more artifacts than the supratentorial space due to the proximity of bone (susceptibility artifacts), the pulsatility of blood in the basilar artery and transverse sinuses, and the CSF-flow artifacts in the cisterns and fourth ventricle.
Imaging protocols are many, depending on local traditions and technical availabilities. Obviously MR imaging is the modality of choice and, as always, it must be multi-planar and multi-sequential. Because the brainstem is so small (diameters of the medulla are 11–13 mm, those of the midbrain, 20–27 mm; those of the pons are the largest, at 20–40 mm), thin slices (2–3 mm) are needed, without any significant gap. 3D-T1GE millimetric images (either MPRAGE or TFE or SPGR depending on the vendor) are useful, given their excellent gray-white contrast and the possibility of secondary reformation of the images in any plane. Thin sagittal T2 sections are useful too: both T1 and T2 sagittal sections show the craniocaudal extent of the lesion well. T2-FLAIR is often considered the best sequence to appreciate the full extent of glioma [3], and axial cuts are especially useful to show the transverse extent of the lesion. The three T1, T2, and FLAIR sequences contribute to the analysis of the structural changes (solid, cystic, necrotic, edematous components); because of their high degree of hydration, gliomas typically are dark on T1 and bright on T2 and T2-FLAIR. However, isolated hypercellular malignant foci may appear as T2-dark nodules surrounded by a bright edematous tissue.
In all varieties of gliomas, conventional DWI/ADC typically shows no restriction, but quantitative DTI is more sensitive and it provides a quantification of the diffusivity and may therefore demonstrate foci of high cellularity [4]. Susceptibility imaging may demonstrate the presence of intratumoral hemorrhages: this feature, although not absolutely specific, is strongly suggestive of a high-grade tumor [9]. Also, DTI tractography (although not done routinely) may assess the involvement of the main fiber tracts, assist in the differential diagnosis, and help to direct a surgical biopsy or even an open surgical approach, especially when integrated in the neuronavigation program [7, 23–25]. From a strictly anatomical point of view, the corticospinal tract; the superior, medial, and inferior cerebellar peduncles; and the medial lemnisci can be identified [7].
To evaluate the vascular bed and the vascular permeability (BBB), contrast administration is always mandatory, with axial (usually done according to the requisites of the surgical neuronavigation), sagittal, and coronal imaging planes. For medullary tumors, the cervical spine must be imaged as well, as the tumor often extends caudally, and a subjacent hydrosyringomyelia should then be ruled out. Pilomyxoid astrocytomas may originate in the brainstem [32] and often disseminate in the leptomeninges [33], which justifies whole spinal imaging, typically not at the first study however as the precise diagnosis may be attained later in the course of the disease.
CT has a limited role to play in the diagnosis of brainstem tumors. Its main contributions in a context of tumors are the demonstration of calcification, which are more common in gangliogliomas than in typical gliomas, and a high attenuation suggestive of a densely packed cellular tumor, which in typically not observed into the brainstem.
31.2.3 Imaging Findings
Location is, in tumors of the brainstem like elsewhere in the CNS, an important point to consider when assessing a tumor. Several classifications of the brainstem tumors have been suggested [34], and in all the most important point is to differentiate infiltrative tumors of the pons from tumors elsewhere [35–37], as pontine tumors are essentially all of poor prognosis (FA grade II, III, IV) [11]. This topographic specificity is expressed by giving it the specific name of diffuse intrinsic pontine glioma.
Diffuse intrinsic pontine gliomas (DIPG) (Figs. 31.2, 31.3, 31.4, and 31.5) are centered within the ventral pons (or basis pontis, where the numerous transverse pontocerebellar fibers are located), and typically they expand the pons in every direction. On a sagittal plane the upper and lower boundaries or the pons (pontomesencephalic and pontomedullary) tend to be displaced as the tumor appears initially to squeeze more than to invade the midbrain and medulla. Yet, invasion does occur, and the fact that the tumor crosses the anatomic boundaries of the pons is felt to reflect its malignant nature [12] (Fig. 31.2a, b). In an axial plane the tumor is essentially bilateral, more or less symmetrical (Fig. 31.2c–h). The DIPG often are exophytic anteriorly, filling the cisterns and engulfing the basilar artery (Fig. 31.2c–g), but not so much posteriorly into the fourth ventricle: on the contrary the ventricular floor is stretched transversely and craniocaudally so that the ventricular lumen is expanded rather than effaced, and hydrocephalus is typically absent, at least in the early stages of the disease.








Fig. 31.2
Diffuse intrinsic pontine glioma. (a) Midline sagittal T2. Diffuse expansion of the pons with smooth posterior displacement of the fourth ventricle and patent aqueduct. Filling of the prepontine cisterns by exophytic components (white arrow). Upward compression of the midbrain and downward compression of the medulla. The mass is heterogeneous with areas of necrosis (white arrowhead) and petechial hemorrhages (black arrow). (b) Midline sagittal T1 shows the anatomy, not so much the structure. (c) Axial FLAIR, assumedly the best to appreciate the limits of the tumor. (d) DWI. Uniformly high diffusion with no evidence of restriction. (e–h) Axial T2. Sequential cuts demonstrating the heterogeneity of the lesion with irregular preservation of some tracts and nuclei, areas of necrosis, petechial hemorrhages, etc.

Fig. 31.3
Diffuse intrinsic pontine glioma. Cellular density. (a) Axial FLAIR. In the heterogeneous bright signal of the infiltrative tumor, there is an ill-defined area of lower signal (white arrow). (b) Axial DWI, same level. This area shows some restriction of the water diffusion (black arrow), suggesting high cellularity. (c) Axial gadolinium-enhanced T1. Follow-up study after 3 months demonstrates high vascularity (black arrow) and necrosis


Fig. 31.4
Diffuse intrinsic pontine glioma. Enhancement patterns (axial T1 post-gadolinium administration). (a) No enhancement. (b) Dilated vessels, likely veins (arrows), reflecting the increased blood flow. (c) Dilated vessels (arrows) with ill-defined area of faint enhancement (arrowheads). (d) Nodular enhancement (arrow). (e) Irregular patchy enhancement (arrow). (f) Ringlike enhancement with central necrosis (arrow)

Fig. 31.5
MR spectroscopy of pontine tumors. (a) Normal pattern. NAA (arrowhead) is higher than Cr (short arrow) and Cho (long arrow). The three are aligned, the peaks decreasing from right to left (yellow line). (b) DIPG. Assuming that Cr remains invariant, NAA (neuronal activity) is low (arrowhead), and Cho (cellular turnover) is extremely high (long arrow). (c) DIPG. Same pattern but in addition there is a lactate doublet (arrow) that reflects cellular necrosis
Uncommonly, dorsal exophytic gliomas may originate in the tegmentum pontis, and they typically are of low grade (PA grade I). They protrude into the fourth ventricle and are associated with hydrocephalus. Also, lateral tumors (Figs. 31.6 and 31.7) may develop into the brachium pontis (middle cerebellar peduncles) and seem to be more commonly of low grade (PA grade I) as well.



Fig. 31.6
Lateral pontine gioma (pilocytic astrocytoma). (a) Sagittal T1. Huge pontine mass, heterogeneous, with significant posterior expansion and hydrocephalus (dilated third ventricle). (b) Axial FLAIR. The mass is centered on the right middle cerebellar peduncle. (c) Axial T1 with contrast administration. No significant enhancement. (d) “Malignant” pattern of the MR spectrum with increased Cho (high cellular turnover), low NAA (neuronal activity) and Lac doublet. A surgical partial exeresis of the posterior exophytic component disclosed a pilocytic astrocytoma (WHO grade I). (This case illustrates the fact that when dealing with gliomas, location is more contributive to the diagnosis than MRS.) Perfusion would have shown a low CBF and high contrast leakage in this case of PA, in contrast with a high-grade glioma which would have shown a high CBF

Fig. 31.7
DTI tractography. (a) Highly malignant pontine tumor. (Upper) Axial T2 demonstrates areas of high cellularity (long arrows), hemorrhage (short arrow), and dilated vessels (arrowheads). (Middle) FA map demonstrates complete loss of the organization of the fiber tracts in the pons. (Lower) Craniocaudal fiber-tracking of the corticospinal tract reveals that they are interrupted at the level of the tumor. This may reflect a destruction of the fibers, or a massive extracellular edema with loss of anisotropy. (b) Low-grade lateral pontine glioma. (Upper) On the axial FLAIR image the tumor is centered on the left cerebellar peduncle. (Middle) The FA map shows a partial distortion but a general preservation of the organization of the fiber tracts (compare with a). (Lower) The corticospinal tract on the left is displaced medially but otherwise preserved
Cervicomedullary gliomas are another typical presentation of brainstem tumors [38] (Figs. 31.8, 31.9, and 31.10). They are centered at the junction of and occupy the upper cord and caudal medulla, which are both circumferentially expanded (Fig. 31.8c), typically with an exophytic component into the caudal part of the fourth ventricle resulting in its obstruction (Fig. 31.8a, b, d); hydrocephalus may or may not be present [39]. The histology of these tumors is characteristically benign (PA grade I). It has been suggested that their particular pattern of extension sparing the rostral medulla (Fig. 31.8a, b, d) was related to the decussation plane (pyramidal decussation, internal arcuate fibers, olivocerebellar tracts) (it might perhaps reflect the brainstem compartmentation in rhombomeres as well.) and that this behavior would reflect their nonmalignant nature [12]. This may not be absolutely true however as some truly benign cervicomedullary tumors may secondarily invade the upper medulla (Fig. 31.9b) and may extend to the pons (Fig. 31.10). Extension often is more lateral toward the inferior cerebellar peduncle as well. Strictly focal intrinsic medullary tumors are uncommon, typically (but not always) benign, and may be exophytic (Fig. 31.11) or infiltrative, in a surgically difficult location [40, 41].








Fig. 31.8
Cervicomedullary pilocytic astrocytoma. (a) Midline sagittal T1. The tumor seems to originate from the upper cord, to extend into the lower medulla and to emerge from the dorsal surface of the lower medulla into the cisterna magna: this fits the model according to which the tumor wouldn’t cross the decussation plane (or the inter-rhombomeric plane). The mass appears homogeneous, solid, and on its dorsal exophytic component lined with a normal-looking layer of brain tissue. (b) Midline sagittal T2. Similar findings, with the core of the tumor being bright; the cord below the tumor seems to be edematous. (c) Axial FLAIR. Diffuse enlargement with concentric bulging into the cisterna magna. (d) Midline sagittal T1 post-gadolinium. Diffuse enhancement of the mass with a few necrotic patches, consistent with the diagnosis of low-grade PA

Fig. 31.9
Cervicomedullary pilocytic astrocytoma. Long-term progression. (a) Midline sagittal T2, age 6 years. Tumor of the upper cord with exophytic growth into the cisterna magna, sparing the upper medulla. (b) Midline sagittal T1, age 12 years. The tumor has expanded into the medulla and is now reaching the pontomedullary boundary (therefore has crossed the decussation plane)

Fig. 31.10
Cervico-medullo-pontine diffuse glioma, identified as low-grade (13-month-old boy). (a) Sagittal T1. The tumor is centered on the medulla and extends toward both the upper cord and the pons. (b) Midline sagittal T2. The tumor appears ventrally located with exophytic nodules in the prepontine and premedullary cisterns while the dorsal aspect of the medulla appears better preserved. (c) Axial T1 post-contrast. Dilated vessels, concentric enlargement of the medulla with apparent preservation of its dorsalmost aspect


Fig. 31.11
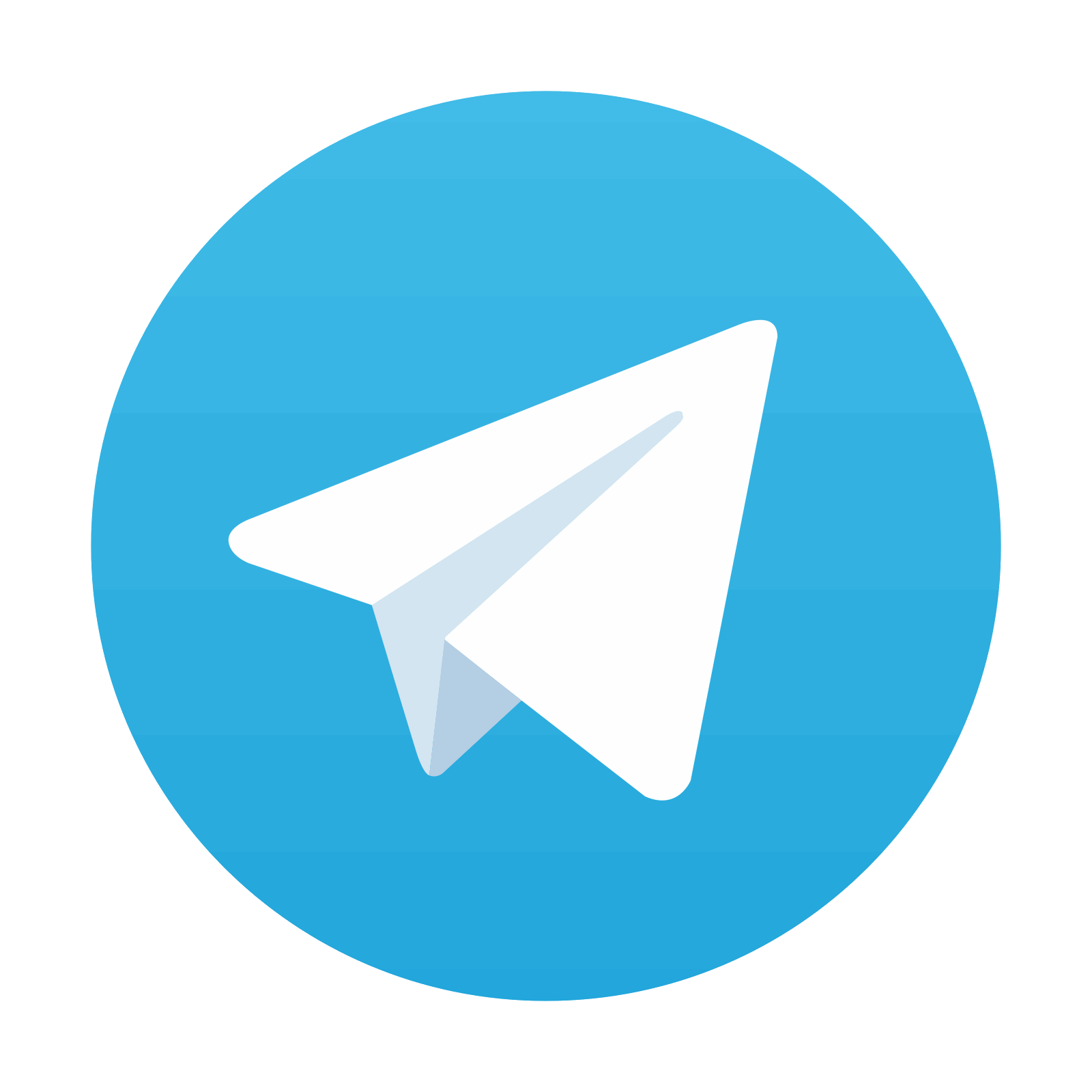
Medullary glioma, focal. (a) Midline sagittal T1. The tumor is located in the medulla and is exophytic dorsally into the cisterna magna. (b) Axial T2. The tumor is mostly dorsal, somewhat lateralized to the right. (c) Axial FLAIR. The tumor appears essentially solid. (d) Lateral sagittal T1 post-gadolinium. The tumor is irregularly enhanced, which still is consistent with the diagnosis of low-grade glioma. It extends superiorly into the inferior cerebellar peduncle
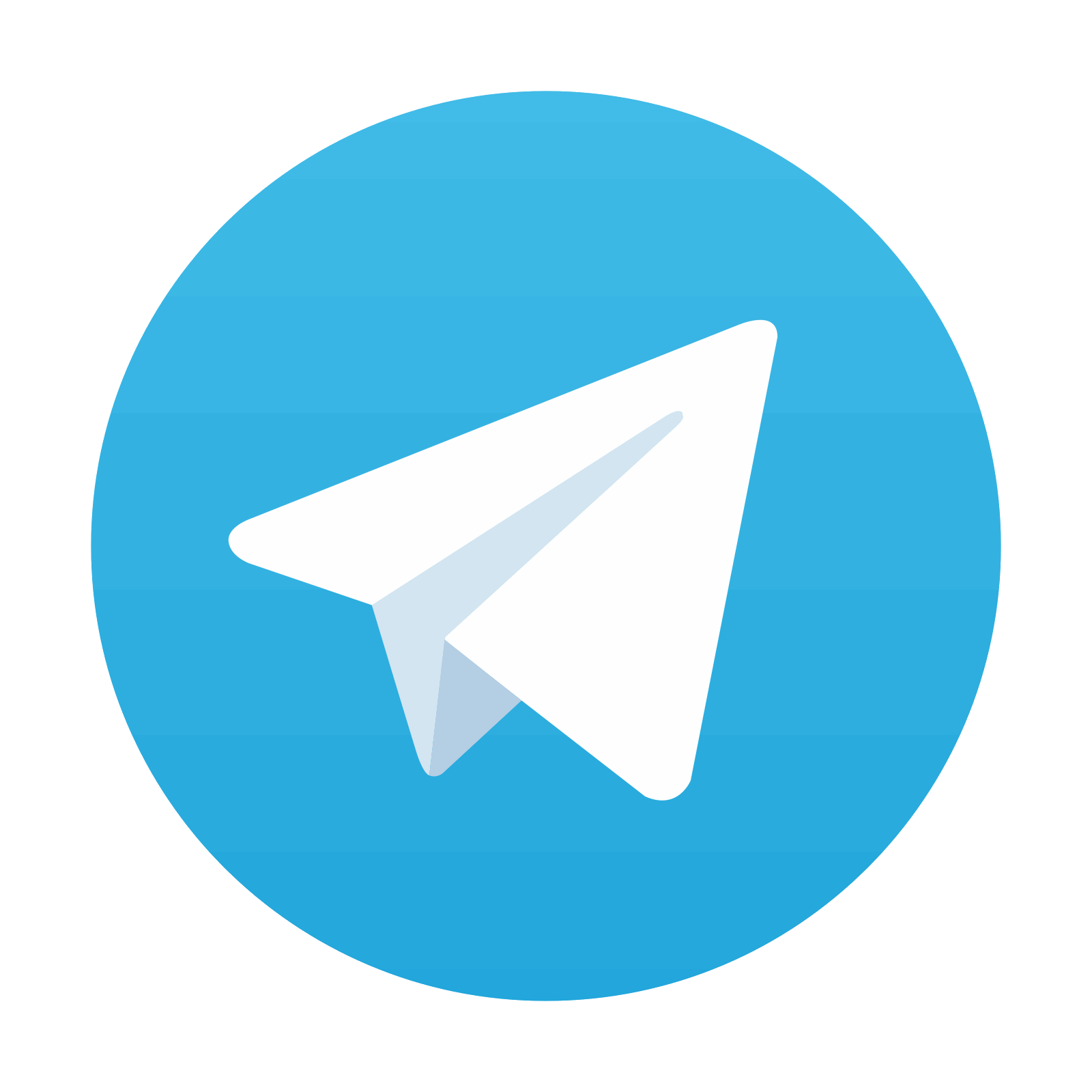
Stay updated, free articles. Join our Telegram channel

Full access? Get Clinical Tree
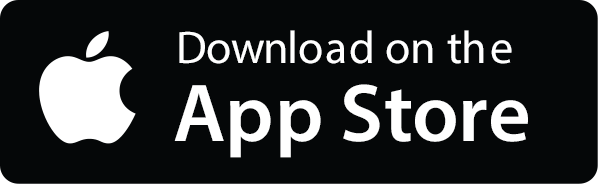
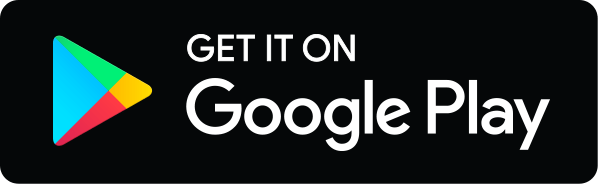
