Fig. 16.1
Note the more posterior cerebral hemisphere of this normal 20 week fetus is clearly seen while the more anterior cerebral hemisphere of the fetus is obscured (white arrow) and the lateral ventricle (LV) is not visualized
Magnetic resonance (MR) imaging entered the arena of prenatal imaging in the early 1990s with the development of ultrafast sequences taking less than a second per image, which permitted the very active fetus to be caught by the MR camera (Fig. 16.2) [3]. Fetal MR imaging has several advantages over prenatal sonography but first and foremost is the improved contrast resolution. This superior contrast resolution coupled with MR’s better spatial resolution, larger field of view, and multiplanar imaging capabilities, permits the morphology of the developing brain and spine to be more clearly visualized than with US. MRI allows superior delineation of the cortex, ventricles, subarachnoid spaces, and posterior fossa structures. This MR sensitivity allows assessment of the integrity of the brain parenchyma, an analysis critical in predicting neurocognitive outcome. Parenchymal US abnormalities that are better visualized with MR include malformations of cortical development, destructive lesions, sulcation anomalies, periventricular nodular heterotopia , cerebellar dysplasia, periventricular leukomalacia, germinal matrix hemorrhage, and intraventricular hemorrhage [4–8].


Fig. 16.2
Coronal T2 MRI of the fetal face at 23 weeks GA
Abnormalities of the fetal brain typically present on the prenatal screening anatomic sonogram when fetal ventriculomegaly or an abnormal posterior fossa are most commonly identified. A subsequent MR can confirm the US abnormality but often discovers additional pathology not appreciated on US. Studies have shown that fetal MR can detect additional pathology in 36–57 % of CNS anomalies not found on conventional 2D transabdominal US [9]. Fetal MR can also prove US findings false and confirm a normal fetal brain.
The information provided by fetal MR can affect decision making for parents and physicians, altering counseling, delivery, and neonatal care. The management of the pregnancy may be changed in up to 47 % of cases [10]. Recent developments in three-dimensional (3D) sonography (Fig. 16.3) can nearly compete with the 3 plane resolution achieved by MR in some cases and there are some tissue characteristics that are still best appreciated on US such as calcification and blood flow [11]. 3D sonography is better at detecting intracranial calcification (Fig. 16.4), vascular abnormalities (Fig. 16.5), intratumoral vascularity and bone dysplasia compared to MR [12]. 3D ultrasound can also demonstrate the abnormalities seen outside the CNS, frequently associated with CNS abnormalities. The 3D transvaginal high-frequency approach can still be limited due to lack of penetration and skull ossification. However, 3D US especially transvaginal, can approach, if not match the detection rate of CNS abnormalities by MR according to some authors [13, 14]. The transvaginal technique however has not been successfully popularized in the United States.




Fig. 16.3
3D US of a 23 week GA fetus

Fig. 16.4
2D US example of a periventricular calcification from congenital toxoplasmosis not seen on MR

Fig. 16.5
Color Doppler of the MCA (middle cerebral artery) (white arrow) with increased flow in a case of anemia
The most significant limitation of fetal MR imaging remains fetal motion. Sedation of the mother and fetus is not usually done although the injection of curare into the umbilical cord to obtain fetal paralysis was used before the development of ultrafast pulse sequences [15]. Despite the continuing technical advances, which permit increasingly rapid image acquisition, fetal motion remains the greatest technical challenge to obtaining a diagnostic study.
Indications for Fetal MR
MR imaging is not a routine screening tool for the evaluation of the fetal CNS and should be reserved to better evaluate abnormal findings on the second trimester screening anatomic US or lack of cranial visualization due to the usual culprits (decreased amniotic fluid, large maternal body habitus, position of the fetal head, shadowing from the calvarium in later gestational age GA ).
Common abnormalities of the brain suspected or not adequately assessed by US include vascular malformations, hydranencephaly, and infarctions. Finally the suspected congenital anomalies of the spine poorly assessed by US include neural tube defects, sacrococcygeal teratomas, caudal regression/sacral agenesis, and vertebral anomalies [16]. Destructive brain lesions related to maternal coagulation disorders, maternal hypoxia, maternal trauma, and fetal demise in multiple gestations are all indications for fetal brain MR [17].
Known central nervous system (CNS ) malformations or chromosomal aberrations in siblings and a family history of genetic disorders involving the CNS also warrant MR evaluation, even with a preceding normal US examination [18].
Fetal MR imaging is now commonly used before prenatal neurosurgical intervention in confirming the diagnosis and planning potential surgical options. It is also important for screening the fetal brain both before and after other non CNS surgical interventions. The high risk to mother and fetus of potential in-utero surgery requires accurate assessment of all anomalies. This includes Chiari II/meningomyelocele, sacro-coccygeal teratomas, and complications of monochorionic twins needing surgery [19–21].
Safety of Fetal MR Imaging
Fetal MR has been performed since the mid 1980s and in those 30 years no fetal injury due to MR has been documented [22, 23]. Studies performed to assess the safety of MR imaging in pregnant animals and animal embryos found no injurious results; however, there has been no official consensus that there is “no risk” of fetal MR imaging [24]. There are no published reports of adverse long-term effects of fetal MR in children who were imaged as fetuses, but these studies are limited by small sample size [25–27]. Because of the potential risk of MR imaging to the developing fetus in organogenesis and the current technical limitations of fetal MR imaging, we recommend practitioners wait until after the first trimester before performing fetal MRI.
Other fetal MRI risks include heat absorption and acoustic trauma [28, 29]. Only the 1.5T platform is used at our institution for fetal MR as at 3T the risk of heating and acoustic trauma is theoretically greater. Regarding noise, so far no studies have shown sustained increases in fetal heart rate during MRI and there is no evidence of hearing impairment in children who had undergone MRI as a fetus [27, 30].
Imaging Techniques: US
Prenatal sonography is the currently the initial modality of choice to evaluate the development of the fetal CNS. Prenatal screening for neurologic abnormalities is usually based on US performed either routinely in the second trimester or after abnormal maternal alpha-fetoprotein (AFP ) screening in the second trimester. Indications of uterine infection, known teratogen exposure, abnormal fetal karyotyping, and IVF are additional reasons for a focused CNS study in the first trimester [31].
In the first trimester high-resolution transvaginal 3D ultrasound has enabled the routine visualization of small embryos and fetuses. The anatomy of the cerebral ventricles can be seen as early as 9 weeks. US can now accurately diagnose neural tube defects such as a cranial encephalocele, acrania/anencephaly, and holoprosencephaly in the first trimester by week 12 [32]. The basic US examination in the second trimester is performed via conventional transabdominal US using 3 standard axial sections through the fetal brain.
3D/4D ultrasound allows scanning of the brain transabdominally or transvaginally generating images in all three classic scanning planes. Similar to MRI, 3D US gives the ability to view the brain from volumetric datasets. Simultaneous analysis in the three orthogonal planes facilitates both the basic and the detailed structural evaluation of the brain allowing better assessment of fetal brain from 20 weeks onward [12].
On a traditional screening to investigate the fetal brain from the second trimester onward, 2D transabdominal US, three standard axial sections through the fetal brain are generated: transventricular, transthalamic, and transcerebellar, [33]. The transthalamic (Fig. 16.6) and the more cranial and parallel transventricular plane (Fig. 16.7) display the minimal requirements for the basic mid-trimester anatomical survey of the cerebrum. The transcerebellar plane, obtained by rotating the probe posteriorly over 30°, images the posterior fossa (PF) (Fig. 16.8). Pertaining to the fetal CNS, there is a biometric component and an anatomic component. The biometric component includes the biparietal diameter (BPD) (Fig. 16.9) and head circumference (HC) (Fig. 16.6). The anatomic components include: the head, face, neck, lateral cerebral ventricles, midline falx, cavum septi pellucidi (CSP), choroid plexus, cerebellum (C), cisterna magna, and the spine (cervical, thoracic, lumbar, and sacral). The addition of color Doppler flow imaging enables the identification of arterial and venous flow.





Fig. 16.6
2D US of the transthalamic plane with a head circumference measurement at 20 weeks GA. White arrows indicate the laminae of the normal cavum septum pellucidum

Fig. 16.7
2D US at the transventricular plane demonstrating the normal prominent choroid plexus within the ventricles (arrows)

Fig. 16.8
2D US of the transcerebellar plane with both cerebellar hemispheres marked by white arrows. V = vermis. T = thalamus. CM = cisterna magna

Fig. 16.9
Biparietal diameter measured at the maximum transverse width in a 20 week GA fetus
The fetal spine typically is imaged in the three scanning planes, namely sagittal, coronal, and axial; similar to the brain scan it can be done using 2D or 3D sonography. Imaging the cervical, thoracic, lumbar, and sacral spine should display the minimal elements of an examination of the fetal spine. In addition an effort should be made to demonstrate the intact fetal skin covering the spine. When an abnormality is suspected, more extensive imaging of the spine is required. 3D views are obtained with the fetal spine in the sagittal plane. Surface and bone rendering improves visualization (Fig. 16.10).


Fig. 16.10
3D US of a 20 week GA fetal thoracolumbar spine
Although the fetal face is not considered as part of the fetal CNS, its examination is an essential component of the total evaluation of the fetal CNS (Fig. 16.2).
Imaging Techniques: MR
Our routine fetal protocol attempts to reduce the potential limitations in fetal MR imaging including fetal motion, maternal discomfort, and the technical difficulties of scanning a small moving structure far from the receiver coil. To minimize the difficulties created by the small size and excessive motion of younger fetuses it is ideal to wait until at least gestational week 22 to perform the MRI. Due to parental anxiety, many studies are performed earlier but we try not to preform studies before 20 weeks especially for minor abnormalities where finding additional CNS abnormalities is the real indication for the study. Gestational age limits on termination vary state by state and must be considered in the timing of the study.
Most of the anatomic information from fetal MR is obtained from ultra fast T2 weighted sequences. Axial, sagittal, and coronal image sets of the fetal brain are obtained in a sequential manner unless the fetus moves out of the plane of acquisition, unfortunately a frequent occurrence. Images are acquired during free maternal breathing with a small field of view, adjusted for increased fetal and/or maternal size. These sequences are obtained at a slice thickness of 3 mm for the brain and at 2 mm for the spine [34].
T1 weighted imaging is less useful as the scanning times are much longer and more subject to fetal motion. FSPGR images with 5-mm slice thickness are performed during a single maternal breath hold and allow detection of hemorrhage, thrombosis, fat, or calcification (Fig. 16.11). Gradient-echo images with high sensitivity to paramagnetic susceptibility are used to identify hemorrhage and mineralization as well as osseous and vascular structures [35, 36]. Advanced MR techniques such as spectroscopy, diffusion tensor imaging (DTI), dynamic MRI, and resting state functional MRI have been successfully applied to fetal MR imaging but are not routine or diagnostic.


Fig. 16.11
Thrombosing dural sinus malformation with T1 hyperintense clot (arrow) in the enlarged torcular Herophili. (a) Axial T2 weighted image. (b) Axial T1 weighted image at same level
Normal Fetal CNS Anatomy and Development —US
The age of the pregnancy is key when imaging the fetal brain, as the CNS develops in a sequential fashion with a specific time line when each new structure should appear.
During the first trimester there is a very rapid development of the brain; however, as the second and the third trimesters approach, the CNS formation slows. By 16 weeks the ventricles, cerebral and cerebellar hemispheres are formed and much of the third trimester development is the gyration and sulcation of the hemispheres. The brain experiences a dramatic growth in utero with a near 40-fold increase in the weight of the brain by the time of birth [37].
Ventricles
Naturally the transventricular standard plane best demonstrates the size and configuration of the lateral ventricles, with the anterior landmarks of the cavum septi pellucidi seen between the frontal horns and the echogenic choroid plexus seen within the posterior ventricle. The atrial diameter is measured from inner wall to inner wall (Fig. 16.12). A measurement up to 10 mm is considered normal at any second and third trimester gestational age. The germinal matrix itself is not well-delineated with US.


Fig. 16.12
2D US with a normal 6 mm measurement of the atrial diameter of the lateral ventricle
Midline Structures
The cavum septum pellucidi (CSP ) are paired lamellae separated by CSF between the frontal horns. Under normal conditions it should be easily detected at 16–18 weeks. It is seen anterior and superior to the third ventricle during the routine measurement of the biparietal diameter (Fig. 16.6). Its identification virtually excludes complete agenesis of the corpus callosum (ACC) [33]. Lesions or non-visualization of the CSP might be associated with a variety of abnormalities, including agenesis of the corpus callosum (ACC), septo-optic dysplasia, holoprosencephaly, schizencephaly, porencephaly/hydranencephaly, basilar encephaloceles, and severe hydrocephalus [38] In the transventricular plane the CSP is seen between the frontal horns of the lateral ventricles.
Parenchyma
The fetal brain parenchyma is hypoechoic in contrast to the echogenic choroid plexus within the lateral ventricles. The supratentorium is imaged when obtaining transventricular and transthalamic views. Landmarks anterior to posterior for the transthalamic view are frontal horns of the lateral ventricles, CSP, thalami, and hippocampi.
Sulcation
The normal US appearance of the cerebral cortex in the second and third trimester is required knowledge to date the fetus as well as recognize abnormalities. A fissure or sulcus is first seen as a small dimple that deepens, forming a surface notch and an echogenic line that extends into the brain and begins to branch [39]. The interhemispheric fissure is the first to be identified at 14–15 weeks and the sylvian fissure can be recognized by 18 weeks gestation [40].
Posterior Fossa
Evaluation of the posterior fossa is achieved with the transcerebellar axial image (Fig. 16.6) obtained anterior to posterior: thalami, cerebellum, and cisterna magna (CM). The CM is a fluid collection posterior to the cerebellum with normal anterior to posterior diameter between 2 and 10 mm [41]. The vermis does not cover the fourth ventricle until 18 weeks gestation, so Dandy–Walker complex should not be diagnosed before this time [42].
Skull
The echogenicity, size, and shape of the skull must be routinely examined. The skull should have normal sutures and be scrutinized for bone defects. Bone or transparency mode and three-dimensional imaging can improve visualization of the fetal skeleton [43].
Spine
The initial 2D images should begin with the axial plane, which is best for identifying the three ossification centers of the spinal column and the integrity of the overlying skin [44]. Ossification of the vertebral bodies proceeds caudally through gestational age with the L5 arch ossified by 16 weeks, S1 by 19 weeks and S2 by 22 weeks, which also can confirm the levels [45].
Normal Fetal CNS Anatomy and Development—MR
Ventricles and Subarachnoid Spaces
In early gestation, the ventricles and subarachnoid spaces appear enlarged because of the immaturity and small size of the brain parenchyma. As the brain develops and increases in weight, the subarachnoid and ventricles appear less prominent (Fig. 16.13). The extra-axial spaces remain constant until 30 weeks, from which they decrease in size. CSF appears bright or white on T2 weighted imaging. The choroid plexus appears as a faint intraventricular T2 dark hypointensity (Fig. 16.14).



Fig. 16.13
Axial T2 weighted MRI at the level of the temporal horns. (a) is a 20 week GA fetus and (b) is a 30 week GA fetus. Note the decreasing subarachnoid space and increasing gyration of the cerebral hemispheres

Fig. 16.14
Axial T2 weighted MRI at the level of the lateral ventricles. (a) is a 20 week GA fetus and (b) is a 30 week GA fetus. White arrows indicate the choroid plexus. Note the decreasing subarachnoid space and increasing gyration of the cerebral hemispheres
Midline Structures
The CSP is a CSF space lined by two thin leaflets, forming the medial wall of the lateral ventricles to the level of foramen of Monroe. The CSP will often enlarge in the third trimester, sometimes mimicking a cyst and disappear as a result of fusion of the leaflets at or near term (Fig. 16.15).


Fig. 16.15
30 week GA fetus. (a) Coronal T2 weighted MRI. (b) Sagittal T2 weighted MRI. Arrows indicate normal corpus callosum
The corpus callosum is the largest commissure of the fetal brain. It develops anterior to posterior between 8 and 20 weeks gestation as axons from the developing cerebral hemispheres navigate across the interhemispheric fissure. The corpus callosum is seen on the coronal plane as a flat structure crossing between the hemispheres (Fig. 16.15a) and on a midline sagittal image as a dark T2 crescentic structure above the fornix (Fig. 16.15b).
Brain Parenchyma
The fetal brain on MRI demonstrates signal patterns reflecting the architecture of the parenchyma. The white matter is mainly water, lacking myelination, and is therefore hyperintense on T2 and hypointense on T1 weighted imaging.
The neurons in the germinal matrix and the cortex/cortical plate demonstrate low signal on T2 and high signal on T1 because of high neuronal cellularity (Fig. 16.16).


Fig. 16.16
20 week GA fetus. (a) Axial T2 weighted MRI. Arrows indicate the T2 dark germinal matrix. (b) Axial T2 weighted MRI. Arrows indicate T2 dark normal cortex/cortical plate
The fetal neurons develop from the germinal matrix also called the ventricular zone, which is a cell dense layer lying immediately adjacent to the walls of the ventricles. Cellular proliferation takes place in the germinal matrix from 8 to 16 weeks. The germinal matrix generates the neuroectodermal elements that constitute the brain parenchyma, giving rise to both neuronal and non-neuronal cells. Neuronal migration begins by gestational week 7; most migration to the cortex is complete by 24 weeks but it is not currently visualized with MR in vivo. Cells from the germinal matrix migrate radially from the ventricular wall to the surface of the brain in an inside-out fashion to form the cortical plate [46]. Once in the cortical plate, the neurons undergo cortical organization and cellular differentiation and the germinal matrix regresses but will persist in the caudothalamic groove for several months after birth [47].
The intervening parenchyma demonstrates three visible layers between the cortex and germinal matrix, which were initially described on T1-weighted images from approximately 20–28 weeks [48] (Fig. 16.17). Myelination follows an organized pattern, progressing from central to peripheral, caudal to cranial, and occipital/central to temporal/frontal [34]. Decreased T2 signal in the dorsal brainstem, consistent with early myelination, should be detected in a fetus at 20-weeks gestational age (Fig. 16.18).



Fig. 16.17
26 week GA fetus. (a) Coronal T2 weighted MRI. Arrow heads indicate the T2 bright subplate. (b) Axial T2 weighted MRI demonstrating a wide cavum septum pellucidum (arrows) with unilateral ventriculomegaly. Arrow heads indicate the T2 dark subventricular and intermediate zones

Fig. 16.18
Sagittal T2 weighted MRI of 20 week GA fetus demonstrating decreased T2 signal in the dorsal brainstem (white arrows) consistent with early myelination
Sulcation
Posterior Fossa
An ependymal lined cystic diverticulum, called Blake’s pouch, develops in continuity with the fourth ventricle and then regresses with fenestration occurring in the area of the foramen of Magendie, giving rise to a normal fourth ventricle and a cisterna magna (CM) [52]. Along the walls of the fourth ventricle and rhombic lips, the germinal matrix gives rise to the neurons of the cerebellum. The cerebellar hemispheres form first, whereas the vermis forms subsequently in the midline. The vermis will cover the floor of the fourth ventricle by 18–20 weeks gestation [53, 54]. The brainstem should have a vertical configuration with rounded pons, which can also be measured to ensure adequate growth [50].
Biometrics
Spine
The spinal cord develops by primary and secondary neurulation at the cranial and caudal ends of the embryo respectively during organogenesis. The proximal segment, extending from the medulla to the mid-lumbar enlargement, develops by “primary neurulation” where the notochord induced neural plate rolls up and forms a hollow tube. Caudally the distal lumbar cord, conus medullaris, and filum terminale forms by secondary neurulation from a solid cell mass which undergoes “canalization and retrogressive differentiation” where the caudal cell mass develops a central cavity and remodels into a tube which aligns with the primary tube [59]. MRI at 20 weeks visualizes the spinal cord after primary and secondary neurulation are complete and an obvious spinal cord is present.
Pathology of the Fetal CNS
The US and MR appearance of CNS pathology will be described together as the studies are usually performed nearly simultaneously at approximately 20–22 weeks gestational age (GA).
Ventriculomegaly
The most common CNS abnormality found prenatally is ventriculomegaly [60] and as a result it is the most common indication for fetal MRI [61]. Ventriculomegaly is defined as an atrial width or diameter greater than 10 mm on both US and MR. Ventriculomegaly can be divided into mild (10–15 mm), moderate (>15 mm with >3 mm of adjacent cortical thickness) (Fig. 16.19), and severe ventriculomegaly (with <2 mm of adjacent cortical thickness) (Fig. 16.20) with increasing ventricular size associated with a poorer prognosis [62]. Corpus callosum anomalies such as a hypoplastic splenium will result in colpocephaly which is disproportionate dilatation of the atria and occipital horns with small frontal horns (Fig. 16.29f). Ventriculomegaly could be the result of an ex vacuo phenomenon secondary to parenchymal hemorrhage, infections, or infarction. It may also be related to hydrocephalus caused by hemorrhage (Fig. 16.21) and CSF obstruction. The prognosis of ventriculomegaly depends on the cause of the ventricular dilatation, the gestational age at which occurs, and its progression; however, neonates with developmental delay can have mild isolated ventriculomegaly in the range of 19–36 % [63].




Fig. 16.19
18 week GA US with moderate hydrocephalus. (a) Transventricular plane demonstrating enlargement of the lateral ventricles with atrial diameters measuring 15 mm. (b) Transcerebellar plane showing enlargement of third ventricle (arrow) without enlargement of the fourth ventricle suggesting aqueduct stenosis

Fig. 16.20
28 week GA MRI with marked hydrocephalus. T2 weighted images in the axial (a, c), sagittal (b), and coronal (d) planes. White arrows indicate small fourth ventricle consistent with aqueduct stenosis

Fig. 16.21
21 week GA fetus. (a) US poorly visualizes the ventriculomegaly secondary to intraventricular hemorrhage. This is clearly visualized on (b) axial T2 MRI where black arrows demonstrate the layering of the hemorrhage in the ventricles. (c) Coronal T2 MRI and (d) axial T2 MRI demonstrate parasagittal encephaloclastic clefts unsuspected by US
Up to 80 % of fetuses with ventriculomegaly have additional abnormalities that are detected by prenatal sonography and/or by postnatal evaluation [61, 64, 65].
Fetal MR can detect additional CNS abnormalities not seen on US in up to 50 % of cases of fetal ventriculomegaly [66, 67]. Findings missed on US include developmental abnormalities, such as agenesis of the corpus callosum, cortical malformations, periventricular nodular heterotopia, cerebellar dysplasia, partial agenesis of the septum pellucidum, porencephaly, multicystic encephalomalacia, intraventricular hemorrhage (Fig. 16.21), and subependymal hemorrhage [66–68]. The destructive lesions are often periventricular areas of T2 hyperintensity, focal defects in the germinal matrix, or larger areas of abnormal signal intensity with or without volume loss involving the overlying cortex (Fig. 16.21). Intraventricular hemorrhage often appears as dark layering in the dependent portion of the ventricle (Fig. 16.22).


Fig. 16.22
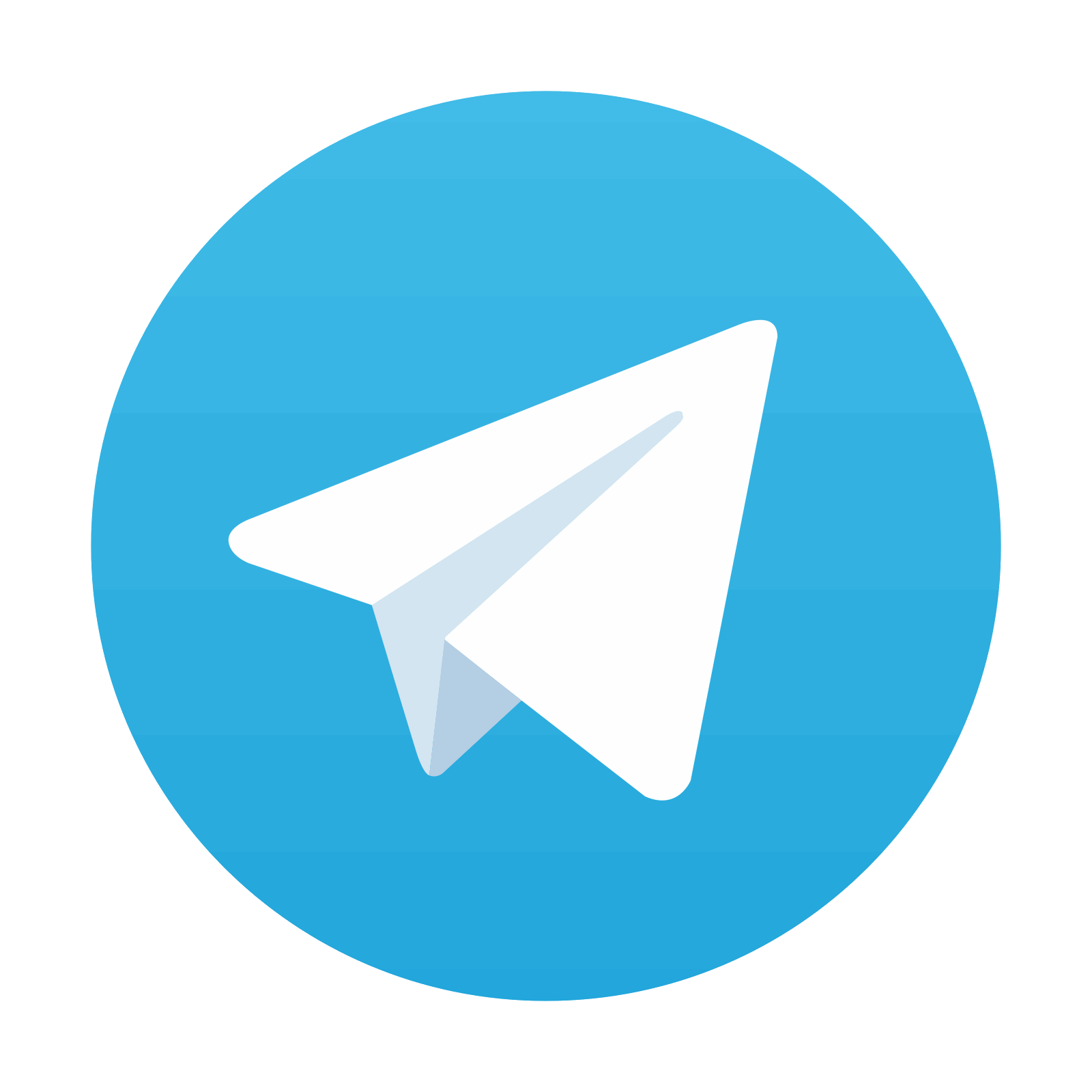
21 week GA fetus. (a) US visualizes the ventriculomegaly secondary to intraventricular hemorrhage. Arrow indicates the CSP. (b) axial T2 MRI and (d) coronal T2 MRI show T2 dark blood fluid levels (white arrows). (c) sagittal T2 MRI demonstrates the vermian cyst unsuspected by US (white arrow)
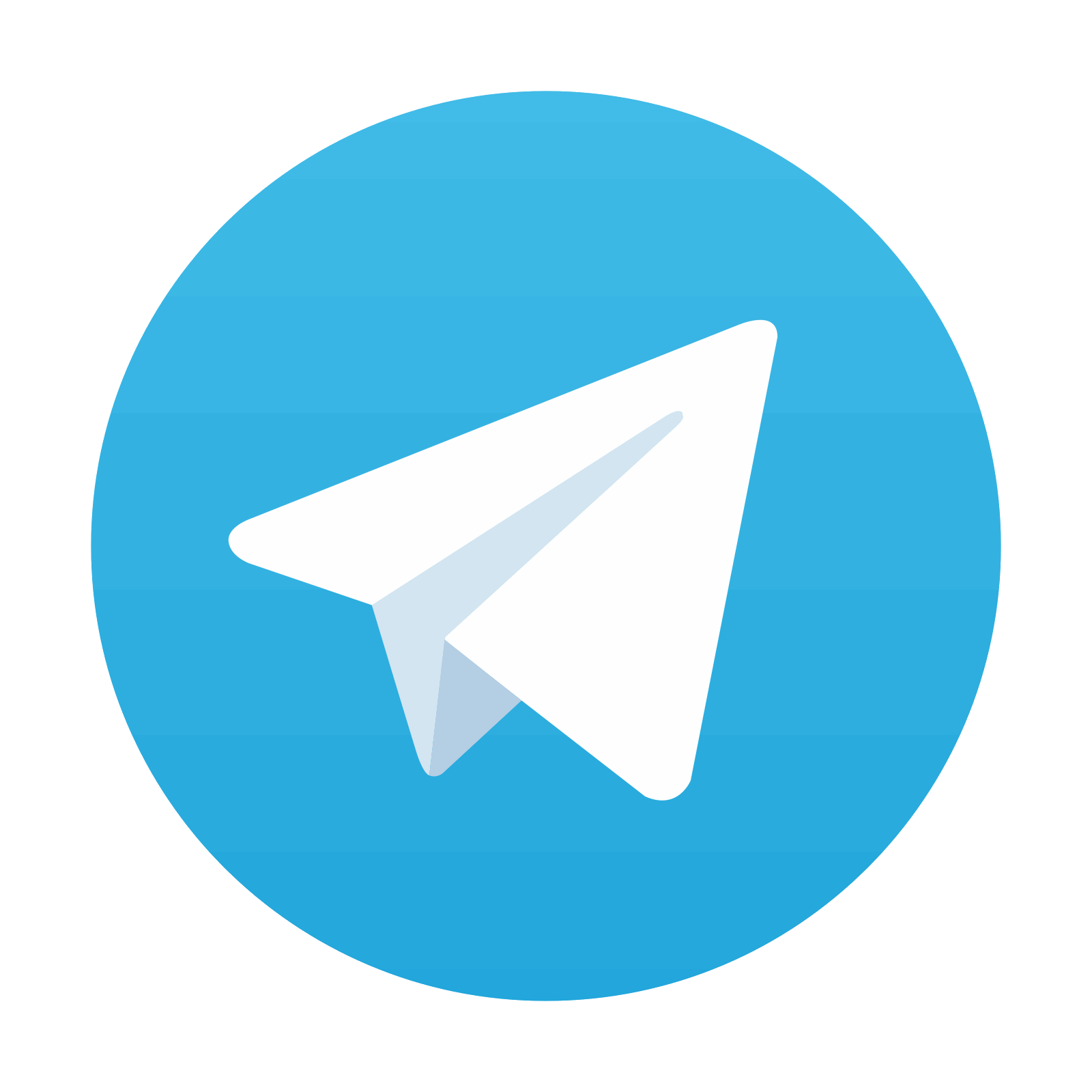
Stay updated, free articles. Join our Telegram channel

Full access? Get Clinical Tree
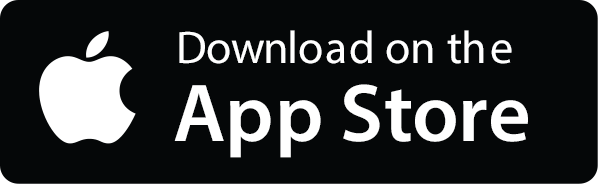
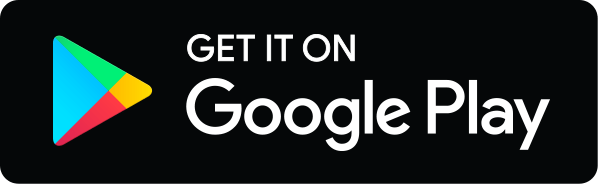
