3 Imaging of the Healthy and Diseased Spinal Disc
Darryl B. Sneag and Hollis G. Potter
Abstract
Magnetic resonance imaging (MRI) plays a critical role in evaluating degenerative disc disease (DDD) and detecting other potential pain generators. As disc degeneration is prevalent in asymptomatic individuals, it is important for the managing physician to carefully correlate MRI findings with patient symptomatology.
Effective MRI evaluation of the spine, and specifically the intervertebral disc (IVD), requires an understanding of image acquisition and the normal appearance of both the disc and its adjacent endplates. This chapter provides a basic protocol, and its underlying rationale, for a spine MRI. It briefly summarizes quantitative MRI techniques to assess disc degeneration that are still largely research tools but may in the future play important roles in early disease detection and management. It also explains widely accepted classification schemes for grading disc and endplate degeneration.
A discussion of disc herniation nomenclature and appropriate descriptors is accompanied by relevant imaging examples and schematics, where appropriate, to help highlight important concepts. Finally, the chapter provides a description and imaging examples of more unusual disc pathology, which are sometimes encountered during routine MRI exams of the lumbar spine.
Keywords: disc degeneration, herniation, Modic classification, MRI, Pfirrmann classification, quantitative MRI, T1-rho, T2 mapping
3.1 Historical Perspective
Back pain is extremely prevalent in the general population, affecting up to 85% of individuals at some point in their lives.1 Degenerative disc disease (DDD) is a common cause of both back pain and radicular symptoms and is a leading culprit of chronic disability in the working population.2 DDD refers to biochemical and structural alterations of the disc under physiological and pathological stresses that result in collagen degradation and proteoglycan (PG) and water matrix depletion.
Historically, imaging of discogenic pain involved the combination of radiography and myelography and more recently, discography and computed tomography (CT) myelography. Although such studies are still ordered to evaluate back pain, these modalities fail to detect many types of disc pathology that magnetic resonance imaging (MRI) can demonstrate.3 MRI is uniquely suited to evaluate disc degeneration and its associated pathology given its high contrast resolution, direct multiplanar capabilities, and lack of ionizing radiation. A large retrospective study evaluating 111 MR examination cases from the Spine Patient Outcomes Research Trial (SPORT) found MRI to be reliable in assessing nondisc contour degenerative findings of the lumbar spine.4 The relationships between abnormalities detected by MRI and symptomatology, however, are controversial and incompletely understood,4,5 and multiple studies have documented extensive imaging pathology in asymptomatic individuals.6,7,8
Quantitative, parametric MRI mapping sequences such as T1-rho9 and T2* mapping,10 ultrashort time-to-echo (UTE),11 and diffusion weighted imaging (DWI),12 including diffusion tensor imaging (DTI), may be able to bridge this knowledge gap by providing information about the microstructure of the intervertebral disc (IVD) and cartilaginous endplate and identify early biomarkers of DDD not detectable with conventional techniques (▶ Fig. 3.1). As these advanced imaging techniques are mainly used for research purposes and not for routine clinical practice, they lie beyond this chapter’s scope. This chapter’s goal is to describe and illustrate the role of conventional MRI in evaluating disc pathology, focusing on the lumbar motion segments.
Fig. 3.1 Coronal T1-rho (a), with corresponding T2-weighted coronal fast spin echo (b), magnetic resonance imaging of the intervertebral discs of a tail of a healthy rat demonstrates higher T1-rho relaxation times of the central nucleus pulposus (arrows) compared with the peripheral annulus fibrosus, reflecting higher proteoglycan content of the nucleus and greater restriction of water and extracellular matrix molecules in the fibrocartilaginous annulus.
3.2 MR Imaging Acquisition
At the authors’ institution, all spine MRI exams are obtained on high field strength 1.5 or 3.0 T systems using dedicated surface coil arrays. For the lumbar spine, this typically involves sagittal short tau inversion recovery (STIR) and T1-weighted fast spin echo (FSE) sequences, as well as axial, coronal, and sagittal T2-weighted techniques. The STIR sequence is used to detect bone marrow edema patterns, indicating stress reaction or fracture, as well as soft tissue abnormalities such as spinal cord and nerve root edema, annular fissures, facet joint cysts, and fascial/intramuscular edema. The coronal sequence is helpful to confirm transitional lumbosacral anatomy, assess coronal balance, and detect extraforaminal disc herniations. At our institution, axial sequences are obtained in multiple overlapped slices without gaps and parallel to the disc space, to best evaluate the degree of central stenosis and disc contour, and in the cervical spine to also assess the degree of neural foraminal stenosis. Protocols in which axial sequences are prescribed only through the disc space, with gaps between disc spaces, may fail to include a disc sequestration or extrusion extending cephalocaudal to the disc space (▶ Fig. 3.2). In the cervical spine, the coronal sequence is replaced with a 2D gradient recalled echo sequence to help differentiate between posterior endplate osseous remodeling and ridges, which are low signal intensity, and disc herniations, which are typically of higher signal intensity. Gadolinium T1-weighted sequences have a role both in the pre- and postoperative setting, but for disc pathology they are typically used to differentiate between postoperative epidural fibrosis, which will enhance, and residual or recurrent disc herniation, which will not enhance.13 In the presence of metallic hardware, imaging parameters of conventional sequences are optimized to reduce susceptibility effects. Dedicated metal artifact reduction sequences developed mainly for arthroplasty imaging, such as MAVRIC (multiacquisition variable resonance image combination) and SEMAC (slice-encoding metal artifact correction), with proton-density weighting or inversion recovery, can also be employed to further reduce susceptibility effects (▶ Fig. 3.3).14
Fig. 3.2 Localizer lines delineate cephalad (e.g., parallel line 1) and caudal (parallel line 10) slices of each axial batch through the lumbar spine on this sagittal T2-weighted sequence (a). Note that as plotted, axial imaging would not include the sequestered disc fragment (arrows) posterior to the L4 vertebral body (magnified in sagittal image) (b).
Fig. 3.3 Sagittal T2-weighted magnetic resonance image of the instrumented lumbar spine (a) demonstrates complete obscuration of the neural foramina by susceptibility effect. Corresponding multiacquisition variable resonance image combination (MAVRIC) proton-density sequence (b) during the same exam demonstrates markedly reduced susceptibility and clear visualization of the left L3 nerve root (arrow) within the L3-4 neural foramen.
3.3 Disc Degeneration Pathoanatomy
The discovertebral complex is formed by the intervertebral disc (IVD) and its adjacent cartilaginous endplate. The disc comprises an inner nucleus pulposus (NP), eccentrically located toward the posterior disc margin, and peripheral annulus fibrosus (AF), which is attached to the cartilaginous endplates. The NP contains mostly water and proteoglycans in a loose network of type II collagen, whereas the AF is a dense, fibrocartilaginous structure comprised mostly of type I collagen. The term degeneration, as defined by Modic et al., refers to various disc pathology including desiccation, fibrosis, disc space narrowing, annular fissuring, endplate changes, and osteophytes at the vertebral apophyses.2
3.4 Disc Degeneration Classification
Pfirrmann et al. developed a five-level grading scheme to describe lumbar disc degeneration using midsagittal T2-weighted FSE imaging and demonstrated adequate intra-and interobserver reliability to discriminate between the different grades (▶ Fig. 3.4).15 The normal disc (Grade I) maintains its height and appears as homogeneous high signal intensity with clear distinction between the high signal intensity central NP and lower signal intensity AF. Grade II maintains a clear distinction between NP and AF but signal arising from the NP is more inhomogeneous. Grade III involves inhomogeneous, intermediate signal intensity of the NP, unclear distinction between the NP and AF, and normal to slightly decreased height. The distinction between NP and AF is lost in Grade IV and disc height can be normal to moderately decreased. In Grade V, the disc space is collapsed and the disc is diffusely hypointense.
Fig. 3.4 Pfirrmann grades on sagittal T2-weighted magnetic resonance imaging. (a) Image demonstrates normal discs in an 11-year-old girl. (b,c) Images in an 18-year-old woman and a 51-year-old man, respectively, demonstrate varying degrees of disc degeneration, classified by the Pfirrmann grading scheme (Roman numerals).
One caveat of interpreting the Pfirrmann grading scheme is that to date, no studies have convincingly correlated disc abnormalities on MRI with patients’ symptoms.2 It is also important to understand that T2 signal intensity changes do not directly reflect decreased water content of the disc. A study of cadaveric spines of various ages demonstrated that absolute T2 measurements correlated more with glycosaminoglycan (GAG) concentration rather than absolute water content.16 Thus, signal intensity likely reflects the state of water rather than total water content and as such, the term disc desiccation should be avoided when interpreting disc T2 signal changes. As disc degeneration progresses, the disc may undergo calcification that typically appears as internal regions of low signal intensity. However, the type and concentration of calcification impact signal intensity; concentrations of particulate calcium of up to 30% by weight may in fact shorten T1 relaxation times and result in T1 signal hyperintensity of the disc.17
3.5 Quantification of Disc Degeneration Based on MRI
Quantitative methods to analyze and characterize disc degeneration are not currently used in routine clinical practice and have been mainly explored for research purposes. If properly developed, however, these techniques might be used to identify individuals or individual IVDs at risk for symptomatic degeneration that could benefit from future therapeutic interventions such as cell-based and growth factor therapies. The IVD has three major constituents: water, collagen, and aggregan–proteoglycan (PG) core and GAG chains. With DDD, there is loss of PG and water, fibrocartilage formation, and disorganization of annular architecture. Several MRI techniques for biochemical evaluation of the IVD currently exist. T1-rho is a spin-lock sequence that employs a lower Larmor frequency to permit the detection of low-frequency physiochemical interactions between water and extracellular matrix (ECM) molecules.18 A positive linear relationship between T1-rho relaxation times and GAG content has been observed in a cadaveric spine ser-ies,18 and T1-rho values also appear to correlate with Pfirrmann grades in both human and animal species.9 T2* mapping involves interrogation of T2 relaxation times of the disc that correlate strongly with water content and weakly with PG content19 and also correlate with Pfirrmann grades of disc degeneration and patient age (▶ Fig. 3.1).20 DWI apparent diffusion coefficient values of normal and degenerated discs show considerable overlap and subsequently may be less useful.21 No prior studies to date have investigated the relationship between biochemical assessment of the IVD with DTI, which may reflect collagen fiber orientation of the disc, and patient symptoms.
3.6 Annulus Fibrosus
Annulus disruption, by conventional wisdom, is the initial mechanism leading to disc degeneration as annular fissures are present in almost all degenerated discs.22,23 The AF is innervated by the recurrent meningeal nerve and thus can be a source of low back pain. Annulus disruption as the causative event of the degenerative disc cascade, however, has not been proven.2 Ye et al. demonstrated that in early stages of disc degeneration, AF disruption was associated with faster subsequent degeneration of the NP,2,24 but a recent longitudinal study found that discs with a Pfirrmann Grade > 2 and an annular fissure were not prone to accelerated degeneration when compared with matched control discs.25
The terms annular fissure and annular tear have been used to describe a localized high intensity zone (HIZ) within the annulus (▶ Fig. 3.5). HIZs reflect granulation tissue and will enhance following contrast administration.26 Most spine societies recommend the term annular fissure be used rather than annular tear due to concern about misconstruing the abnormality as traumatic in etiology. Discography may play an additive role in detecting annular fissures as this modality may reveal fissures not visualized with MRI (▶ Fig. 3.6).26
Fig. 3.5 Lumbar spine magnetic resonance sagittal (a) and axial (b) T2-weighted images in a 47-year-old man demonstrate a broad-based posterior left paracentral annular fissure (arrows).
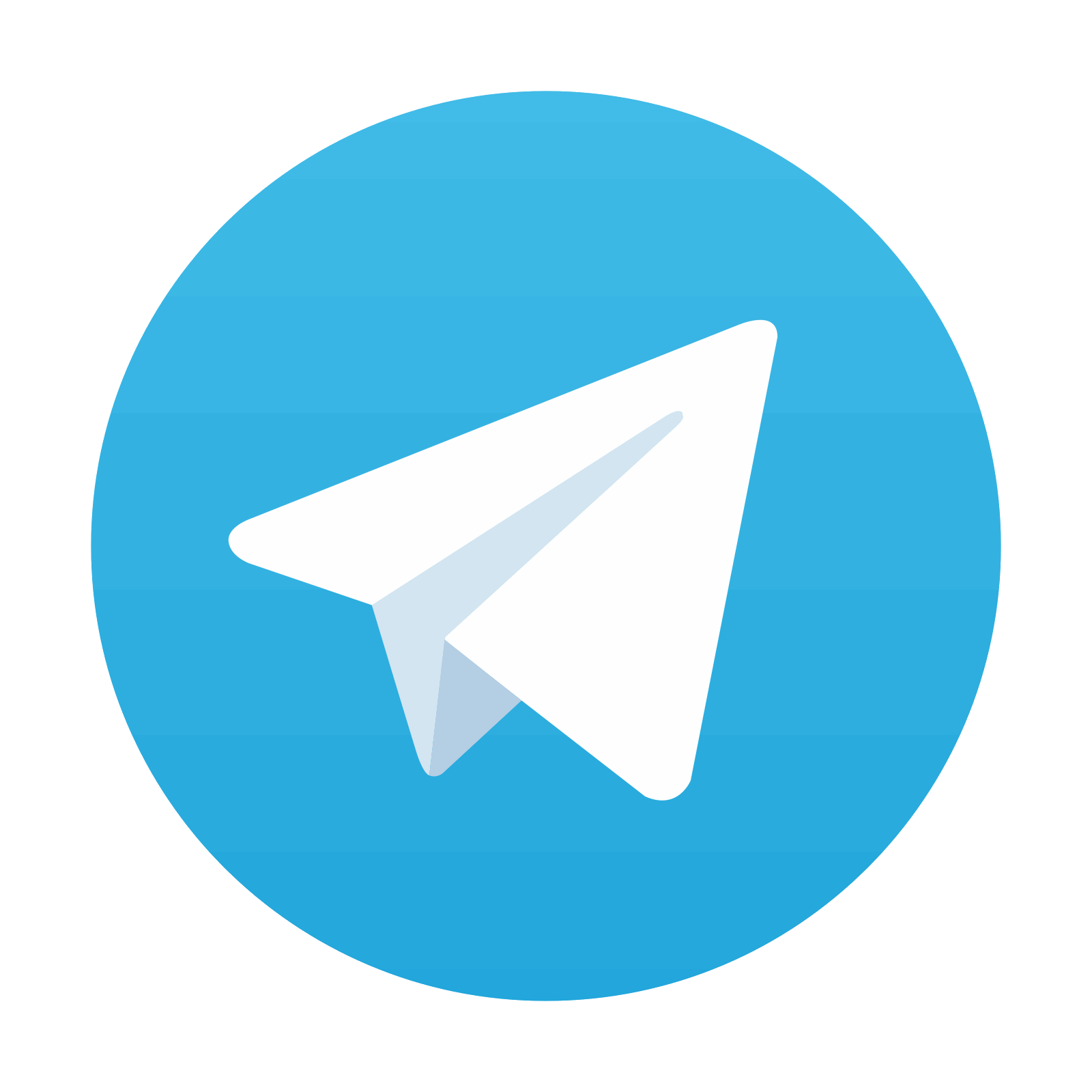
Stay updated, free articles. Join our Telegram channel

Full access? Get Clinical Tree
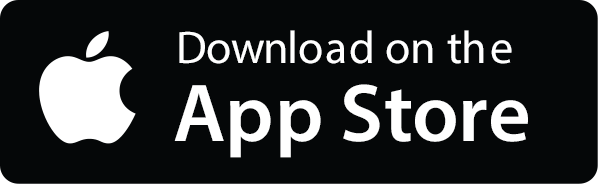
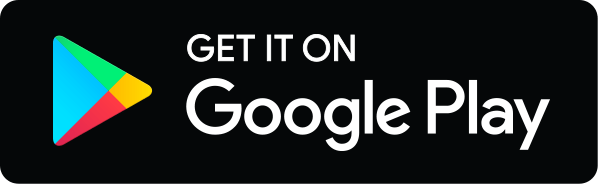