Fig. 10.1
Schematic representation of the basic structure of immunoglobulins. Immunoglobulins are composed of two heavy chains, each consisting of one variable (V H , red) and three constant (C H1 -C H3 , blue) immunoglobulin domains and two light chains, each consisting of one variable (V L , orange) and one constant (C L ) immunoglobulin domain (Paul 2008). Internally the immunoglobulin domains are stabilised by disulphide bonds. The light chain and VH and CH1 domains constitute the Fab (fragment, antigen binding) part of an immunoglobulin, and the CH2 and CH3 domains from the two heavy chains form the Fc (fragment, crystallisable) part. The CH2 and CH3 domains are linked by the hinge region (black), and the hinge regions of the two heavy chain molecules are linked by disulphide bonds (red). The Fc parts of the heavy chains are glycosylated (not shown). Membrane-bound immunoglobulins have an additional, short transmembrane amino acid chain. The paratope is the distal, antigen-binding part, which consists of three hypervariable or complementarity-determining regions (CDR1-3) in the VL and VH domains. Variation in the amino acid sequence of the VL and VH chains arises from somatic recombination of V, D and J gene segments in the immunoglobulin heavy locus (IGH) and somatic recombination of V and J gene segments in the immunoglobulin kappa locus (IGK) and immunoglobulin lambda locus (IGL)
Table 10.1
Immunoglobulin isoforms, subclasses and effector functions. Immunoglobulins differ in their biological function due to differences in their ability to activate the complement cascade and differences in their ability to bind to different Fc receptors on the surface of immune cells, which may have highly variable biological functions (Paul 2008)
Fc binding | Complement activation | Biological role | |
---|---|---|---|
IgA | Mucosal immunity (secreted) | ||
IgA1 | Yes | – | Major IgA subclass |
IgA2 | Yes | – | More prevalent in secreted IgA |
IgD | ? | – | Initial B-cell activation, role in mucosal immunity? |
IgE | Yes | – | Parasite immunity, allergy |
IgG | Broad role in immunity to infectious agents | ||
IgG1 | High affinity | Yes | |
IgG2 | Low affinity | Yes | |
IgG3 | High affinity | Yes | |
IgG4 | Intermediate | – | |
IgM | Yes | Yes | Initial immune responses, natural autoantibodies |
10.2.3 Plasma Cells and Plasmablasts
After activation by binding of cognate antigen to cell surface immunoglobulin, B-cell proliferation is induced, and in the absence of T-cell help, this may be sufficient to induce an antibody response, which is dominated by the secretion of IgM antibodies by plasmablasts. Plasmablasts are B cells that have developed into a phenotype where they retain some B-cell surface markers, but also secrete immunoglobulins (Heyzer-Williams and Heyzer-Williams 2005; Kurosaki et al. 2010). Such T-cell-independent immune responses can be induced by multivalent antigens, e.g. bacterial surface antigens, and elicit initial antibody responses which provide some protection until high-affinity, class-switched T-cell-dependent antibody responses have evolved (Berland and Wortis 2002; Mebius and Kraal 2005).
T-cell-dependent antibody responses develop in germinal centres in secondary lymphatic tissues and involve interactions between antigen-specific TFH cells, B cells and follicular dendritic cells (FDCs) (Victora and Nussenzweig 2012). FDCs are specialised stroma cells that express antigen complexed with complement or immunoglobulins on their surface in the follicular area of secondary lymphoid organs. FDCs are important for the selection of high-affinity, mutated immunoglobulin-expressing B cells (Gonzalez et al. 2011). The interaction between antigen-specific TFH and B cells induces the expression of the enzyme activation-induced deaminase (AID) in B cells. This results in class switch recombination from the initial expression of IgM and IgD to other immunoglobulin isotypes. The precise class switch undertaken is influenced by cytokines present in the environment with, e.g. transforming growth factor-β (TGF-β) inducing class switching to IgA and Th2 cytokines inducing class switching to IgE (Fagarasan et al. 2010). Furthermore, AID induces somatic hypermutation in the variable region of immunoglobulin genes. This stochastic reaction results in the chance generation of B cells expressing immunoglobulin genes, which show increased affinity for antigen (Heyzer-Williams and Heyzer-Williams 2005). Such high-affinity antibody-secreting cells are selected by poorly understood processes and differentiate either to plasma cells or to long-living memory B cells, which are able to generate a swift antibody response upon re-exposure to the same antigen.
10.3 B Cells and Immunoglobulins in the Central Nervous System
10.3.1 CNS Immunoprivilege and B-Cell Responses
The CNS is conventionally considered an immunoprivileged organ (Carson et al. 2006). The presence of a blood-brain barrier, the lack of a conventional lymphatic drainage system and the sparseness of conventional antigen-presenting cells do, indeed, impose constraints on intrathecal immune responses. B-cell responses are, however, readily induced in the CNS and may be of a similar or even higher magnitude than systemic responses to the same antigen (Gordon et al. 1992). Interestingly, it is possible to induce antibody responses to myelin basic protein (MBP) infused into the CSF, and this results in the subsequent protection against experimental autoimmune encephalomyelitis induced by MBP (Harling-Berg et al. 1991). It appears that the administration of antigen to the CSF is especially immunogenic and the induction of an intrathecal antibody response is associated with the development of a clonally restricted, specific antibody response as assessed by analysis for oligoclonal bands (Harling-Berg et al. 1989; Knopf et al. 1995).
10.3.2 Quantitative Assessment of Intrathecal Immunoglobulin Synthesis
Normal CSF contains low concentrations of all immunoglobulins. Immunoglobulins in normal CSF derive from passive diffusion across the blood-CSF barriers (Thompson 1988). Diffusion is size-dependent, and the CSF-plasma concentration quotient for various proteins correlates negatively with the size of the individual molecules (Felgenhauer et al. 1976; Felgenhauer and Renner 1977). The CSF-plasma concentration quotient for albumin (Q alb) is commonly used as a measure of barrier integrity and is used to correct for general blood-CSF protein transfer in formulae for the quantitative assessment of intrathecal immunoglobulin synthesis. The size selectivity of the blood-CSF barriers may, however, become disturbed in disease states, leading to erroneous results when the Qalb is used for correction, especially for larger molecules such as IgA and IgM (Thompson 1988).
There is a close relationship between the Q alb and the CSF-plasma concentration quotient for IgG (Q IgG) in control subjects (Delpech and Lichtblau 1972; Ganrot and Laurell 1974). This relationship led to the development of the IgG index, which is Q IgG/Q alb, for the assessment of intrathecal IgG synthesis (Link and Tibbling 1977). The IgG index assumes that there is a linear relationship between the Qalb and the QIgG. This has, however, been questioned by other researchers, who argue that CSF bulk flow is the major determinant of the CSF protein content and that the diffusion theory indicates that the relationship between the Qalb and the QIgG is better explained by a hyperbolic formula (Reiber 1994). Hyperbolic formulae have also been developed for assessing intrathecal synthesis of IgA and IgM (Reiber 1994).
The hyperbolic model is not universally accepted as other researchers have reported that the relationship between the Qalb and the QIgG is linear in a double logarithmic plot and that there is no statistical evidence suggesting that a hyperbolic formula provides a better fit for the data (Öhman 1994). The relationship observed in double logarithmic plots led to the development of the extended IgG index (Öhman et al. 1989) and was later developed into similar extended indices for IgA and IgM (Öhman et al. 1993).
The extended index model is, indeed, the expected model if a linear relationship between the Qalb and the QIgG is assumed, but measuring inaccuracy is also taken into account (Fig. 10.2). If there is no inaccuracy in the measurements, a linear formula such as the IgG index is well suited to establish increased levels of IgG synthesis. Some measuring inaccuracy is, however, unavoidable, and if the same relative measuring inaccuracy applies both for the Qalb and the QIgG at high and low values, this will result in increased variability in the relationship at higher levels. Thus, the linear formula (the IgG index) will tend to give false-positive results at higher Qalb values (Fig. 10.2a), whereas the formulae based on non-linear relationships, i.e. the extended index (Fig. 10.2b) and hyperbolic formula (Fig. 10.2c), are less prone to this error. Indeed, extended immunoglobulin indices and hyperbolic formulae perform better than conventional indices for the quantitative assessment of intrathecal immunoglobulin synthesis (Öhman et al. 1989, 1993; Sellebjerg et al. 1996). None of the formulae are comparable to the detection of oligoclonal bands for the assessment of intrathecal IgG synthesis (Öhman et al. 1992; Sellebjerg et al. 1996). There may, however, be a role for the assessment of intrathecal IgA and IgM synthesis in the diagnosis of infectious diseases in the nervous system (Deisenhammer et al. 2006).
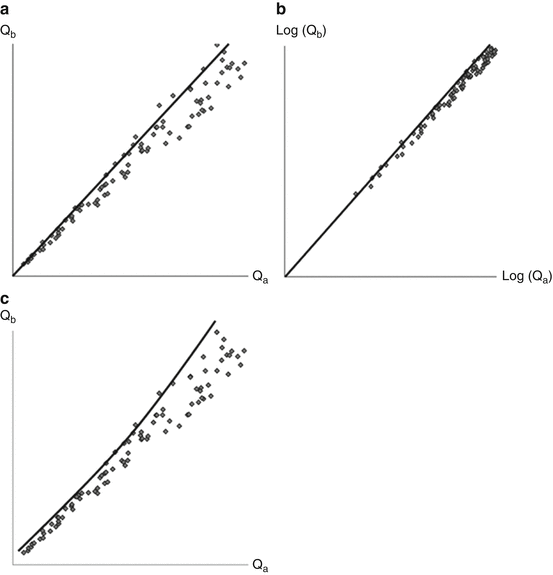
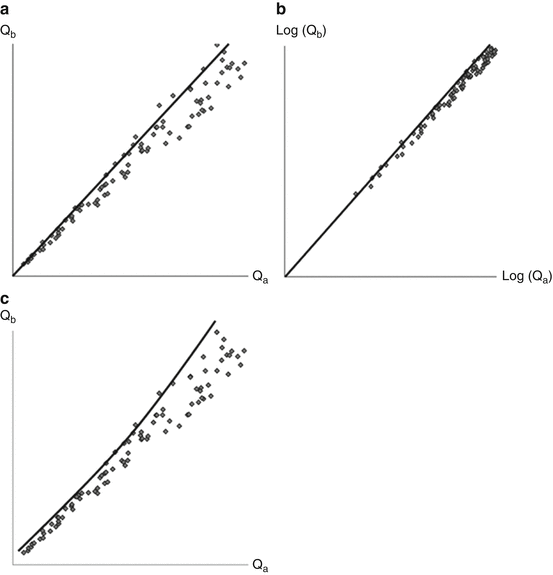
Fig. 10.2
Graphic representation of the consequence of measuring inaccuracy for the use of intrathecal immunoglobulin synthesis formulae (see text for discussion). (a) models a linear relationship, e.g., (b) a double-logarithmic relatoinship, and (c) a hyperbolic relationship between the CSF-plasma quotient of a reference protein (a) and an immunoglobulin of interest (b)
The passive diffusion of immunoglobulins from plasma into the CSF should also be considered when specific antibodies are investigated in patients with CNS infections. Simply calculating an antibody index by comparing the concentration of the specific antibody in CSF and plasma to the CSF and plasma concentrations of IgG is usually sufficient, and similar methods can be applied for the analysis of specific IgM antibodies (Deisenhammer et al. 2006). The application of antibody indices has been used for many antigens, but the results should be interpreted with caution as some patients with MS also show intrathecal synthesis of several virus-specific antibodies, mostly directed against measles, rubella and varicella-zoster virus, the so-called MRZ reaction (Table 10.2) (Felgenhauer and Reiber 1992).
Table 10.2
Selected formulae for assessment of intrathecal immunoglobulin synthesis
IgG index = Q IgG/Q alb | IgA index = Q IgA/Q alb | IgM index = Q IgM/Q alb |
Extended Ig index = Q Ig/Q alb a | ||
Hyperbolic immunoglobulin formulae (Reiber 1994). Hyperbolic 99 % upper reference limits for immunoglobulin quotients are calculated from the Q alb (IgG: a = 0.93, b = 6 × 10−6, c = 1.7 × 10−3; IgA: a = 0.77, b = 2.3 × 10−5, c = 3.1 × 10−3; IgM: a = 0.67, b = 1.2 × 10−4, c = 7.1 × 10−3) | ||
Upper limit of Q Ig = a √(Q alb 2 + b) + c |
10.3.3 Qualitative Assessment of Immunoglobulin Synthesis
The detection of clonally restricted, intrathecally synthesised IgG is a hallmark of multiple sclerosis and is observed in more than 90 % of patients with MS (Freedman et al. 2005). IgG oligoclonal bands can, however, also be observed in other diseases (Kostulas et al. 1987; McLean et al. 1990). An account of the development of oligoclonal band analyses is given in the chapter on the history of the CSF earlier in this book. Briefly, it is recommended that oligoclonal bands are analysed by isoelectric focusing followed by immunochemical detection of IgG (Keir et al. 1990). The simultaneous analysis of the CSF and serum with similar amounts of IgG being analysed is mandatory in order to distinguish between CSF bands resulting from local synthesis and bands derived from plasma (Fig. 10.3).
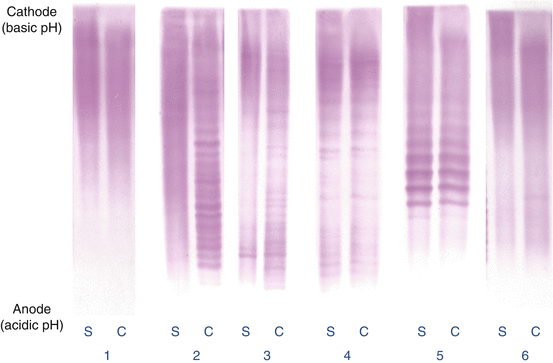
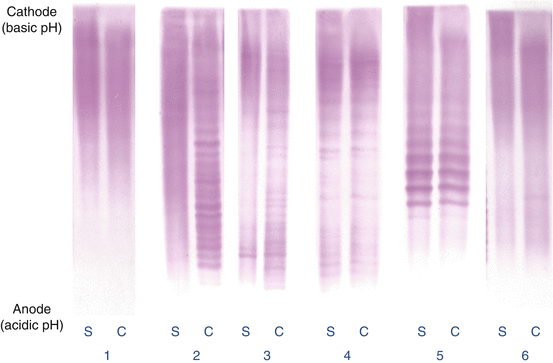
Fig. 10.3
Isoelectric focusing patterns (immunostaining for IgG) showing 1 normal (polyclonal) serum (S) and CSF (C) (no intrathecal IgG synthesis), 2 normal serum and oligoclonal CSF (intrathecal oligoclonal IgG synthesis present), 3 oligoclonal serum and CSF with additional bands in CSF not present in serum (intrathecal oligoclonal IgG synthesis present), 4 identical oligoclonal bands in serum and CSF (mirror pattern, no intrathecal IgG synthesis), 5 paraprotein (monoclonal) resolving in several adjacent bands with a similar pattern in serum and CSF (no intrathecal oligoclonal IgG synthesis), 6 normal serum and single band in CSF (equivocal pattern not considered to represent intrathecal oligoclonal IgG synthesis) (Figure supplied by Poul Erik Hyldgaard Jensen, Neuroimmunology Laboratory, Danish Multiple Sclerosis Center, Rigshospitalet, University of Copenhagen)
It is generally recommended that five different patterns are distinguished: normal pattern, paraprotein, oligoclonal IgG bands only in the CSF, oligoclonal IgG synthesis with identical bands in the CSF and plasma and systemic IgG synthesis with additional bands only in the CSF (Andersson et al. 1994). The latter two patterns are observed in many infectious and inflammatory diseases, whereas the majority of MS patients have IgG oligoclonal bands only in the CSF.
Isoelectric focusing has also been used for the analysis of other immunoglobulin isoforms and for detection of intrathecal synthesis of free immunoglobulin light chains, but these analyses are not in routine use (Deisenhammer et al. 2006; Freedman et al. 2005).
Isoelectric focusing followed by immunoblotting to membranes coated with antigen from infectious agents can be helpful in the diagnosis of CNS infections, but is technically challenging. Interestingly, using methods that provide an estimate of the affinity of the antibody, it has been suggested that antibodies against viral antigens in MS are of low affinity compared to antibodies to the same antigens in patients with viral encephalitis (Luxton et al. 1995; Luxton and Thompson 1990).
10.3.4 Intrathecal IgM Synthesis
Monomers of IgM molecules are joined in pentamers by J chains to form secreted IgM but may also exist as IgM monomers. The high molecular weight of pentameric IgM (900,000 kD) means that quantitative assessment of intrathecal IgM synthesis is especially prone to artefacts due to alterations in blood-CSF barriers. Intrathecal IgM synthesis is observed in MS and infectious and other inflammatory diseases (Öhman et al. 1993; Sellebjerg et al. 1996). The analysis of IgM oligoclonal bands is technically challenging but is less prone to artefacts than quantitative analysis, and it has been suggested that the presence of IgM oligoclonal bands in MS associated with disease activity and a poor prognosis (Sharief and Thompson 1991, 1992; Villar et al. 2002, 2003). Interestingly, intrathecally secreted IgM in the CSF is derived from cells carrying somatic hypermutations, suggesting that they may be derived from an intrathecal germinal centre-like reaction (Beltran et al. 2014).
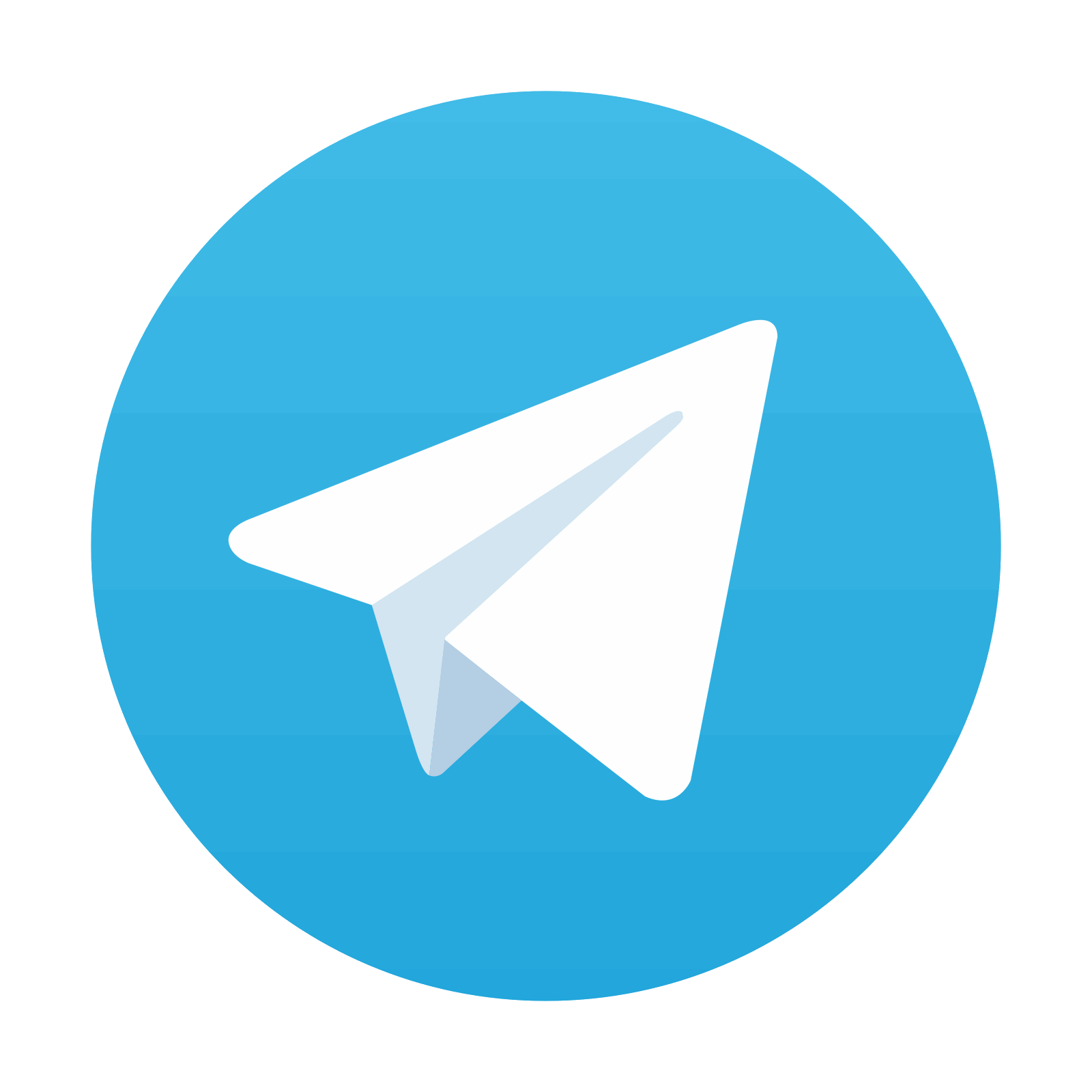
Stay updated, free articles. Join our Telegram channel

Full access? Get Clinical Tree
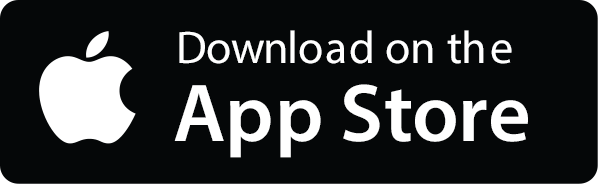
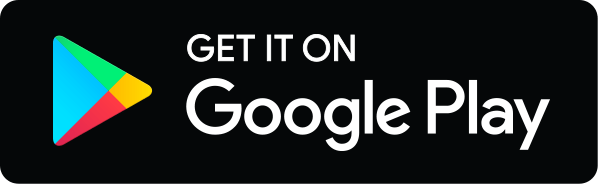