Immunology of the Central Nervous System
The brain is an organ with unique characteristics that function to restrain immunologic responses that could potentially damage tissue. In this chapter, we describe how the immune responses in the central nervous system (CNS) are modulated and regulated. We also discuss the resident immune cells in the CNS, the trafficking of lymphocytes across the blood–brain barrier (BBB), and the chemokines that function to recruit lymphocytes in the CNS microvasculature.
Immunosurveillance of the Central Nervous System
Certain organs, such as the brain, eyes, and testicles, are particularly sensitive to damage due to inflammation. Thus, these tissues have developed singular properties that limit the immunologic responses to antigenic challenge—a phenomenon that has been termed immunologic privilege.1 In agreement with this hypothesis, Sir Peter Medawar observed that the brain does not reject foreign tissue grafts.2 The rationale for the existence of the phenomenon of immunologic privilege is that activation of the immune system in these organs can be extremely deleterious to the organism. The brain is susceptible to increases in tissue volume because of limitations in space imposed by the dura mater and the cranium. Once neurons are damaged, they cannot be replaced. It is well established that the brain and eyes harbor specialized microvasculature where events such as lymphocyte adhesion to the endothelium and the free exchange of solutes are highly regulated.3–5 Furthermore, the CNS lacks classic antigen-presenting cells (APCs), as well as constitutive expression of major histocompatibility complex I and II (MHC I, II). Similarly, the CNS is devoid of lymphatic vessels.
Currently, it is recognized that the CNS immune privilege phenomenon is not complete, and that foreign materials implanted within the CNS can elicit an immune response, although it is delayed and tightly controlled.6 It has also been observed that tissue grafts implanted within the CNS are eventually rejected, and delayed-type hypersensitivity reactions can occur. Pathogenic autoimmune diseases of the CNS exist as well, as demonstrated by experimental autoimmune encephalomyelitis (EAE). Thus, the CNS is more appropriately described as an immunologically specialized organ than as an immunologically privileged one.
Vascular Anatomy
The CNS microvessels are designed to perform two major tasks: (1) prevent ions and other solutes in the blood from entering the parenchyma and (2) enhance regional blood flow wherever needed. The anatomic structures consist of a series of barriers; these include the blood–cerebrospinal fluid (CSF) barrier, which prevents the free exchange of solutes between the blood and the CSF, and the BBB, which is the most prominent interface between the immune and nervous systems. The blood–CSF barrier results from the presence of the choroid plexus epithelium, in which the cells are connected by tight junctions and are responsible for secreting CSF into the brain ventricles. The BBB consists of endothelial cells of the cerebral microvasculature, pericytes, and astrocytes, which have been well characterized in terms of their morphology and biochemistry. The transcellular passage of molecules across the BBB is inhibited as a result of low pinocytotic activity. The elaborate network of tight junctions between the endothelial cells of BBB7 limits the paracellular diffusion of hydrophilic molecules. It is also known that blood molecules are prevented from entering the parenchyma by several permanently active transportation mechanisms.8
The first evidence that T cells can migrate across the healthy BBB into the CNS came from animal models of neurodegenerative diseases, including multiple sclerosis and EAE. Here, it was demonstrated that autoreactive CD4+ T cells recently activated in vitro could migrate into the CNS and initiate a series of events culminating in inflammation, loss of barrier restriction, edema formation, and demyelination.9 T cells were found to reside in the CNS perivascular spaces, allowing encounter with antigen presented by MHC class II–positive perivascular macrophages.10 T cells in the resting state were unable to migrate across the BBB and could not transfer the disease. This indicated an active role for the BBB in regulating the entry of lymphocytes into the CNS by restricting migration to activated T cells. It is interesting to observe that CD3+ T cells can also be found in postmortem samples of choroid plexus in individuals with no known neurologic disorders, indicating that some lymphocytes may enter the CNS during steady state.11
Immune Cells in the Central Nervous System Environment Background Information
Even under normal physiologic conditions, the CNS is not an environment that lacks cells of hematopoietic origin. Various cells reside in the CNS that constitute a resident, quiescent immune system. The distribution of hematopoietic cells resident in the CNS is compartmentalized, indicative of specific functions attributed to cells present in distinct microenvironments. The major cells of hematopoietic origin present in the CNS are microglia and APCs. Microglia are localized predominantly in the parenchyma of the CNS (although also present in the perivascular area) and function in the maintenance of neuronal homeostasis as well as tissue surveillance, whereas APCs reside predominantly in the interface between the CNS and the blood–CSF space.12–17
Antigen-Presenting Cells
Perivascular Antigen-Presenting Cells
Perivascular APCs consist of a heterogeneous population that includes perivascular macrophages, perivascular microglia, and dendritic cells (DCs).18,19 It is thought that perivascular APCs act as sentinels at the BBB, their endocytic and phagocytic activity scanning for both the presence of pathogens and neuronal damage.20 These cells localize to the perivascular spaces around cerebral vessels of small to medium size.21 Perivascular cells are continuously replenished from the bone marrow, and it is estimated that one-third of these cells are replaced during a trimester period in rats, indicating that precursor cells of perivascular APCs (most likely monocytes) can cross the intact BBB.22
Perivascular macrophages have a role in the recruitment of lymphocytes across the BBB and can also promote the invasion of activated T cells into the parenchyma of the CNS.23,24 In addition, it has been shown that these cells have an essential role in the activation of recently immigrated pathogenic CD4+ T cells.18,25 Macrophages and DCs are enriched in the meninges and choroid plexus, probably because of proximity to the CSF space.26 It has been hypothesized that perivascular macrophages sample the CSF for the presence of pathogens, tissue debris, tumor cells, and red blood cells.
Microglia
Microglia are the predominant cell type of the innate immune system within the CNS parenchyma. The distribution of microglial cells varies in the different areas of the CNS, ranging from approximately 5% in the cortex and corpus callosum to 12% in the substantia nigra of the murine brain,27 and from 0.5% in the gray matter of the cerebellum and cerebral cortex to greater than 16% in the pons and medulla in a noninflamed human brain.28,29 Microglia belong to the cell class of hematopoietic mononuclear phagocytic lineage; however, they are less efficient at antigen presentation than DCs. Microglia share many features with other myeloid cells, as they express Fc and complement receptors CD11b and F4/80.30,31 However, some of the molecular differences between microglia and other dedicated phagocytic APCs include secretion of significantly lower levels of superoxide dismutase (SOD) in comparison with macrophages from the spleen or bone marrow.32 Additionally, much of the interaction between microglia and T cells in the parenchyma occurs at a distance and is mediated via cytokine secretion, not antigen specificity.
Microglia appear to survey the microenvironment with highly motile fine protrusions.33 It has been suggested that microglial protrusions survey the environment for inflammatory molecules, thus having an immunologic function. Recent studies, however, have shown that microglia play a role in synaptogenesis,34 and in the monitoring and maintenance of synaptic functions35 as well as the apoptosis of Purkinje cells.36 Thus, microglia appear to have a key function during the development and maintenance of the CNS.37 During embryogenesis, massive developmental apoptosis occurs in the nervous system; twice the number of neurons are generated than is present in the adult organism. Microglial functions have been implicated in the phagocytosis of apoptotic cells. They also promote the death of developing neurons36,38 and are active at synaptic stripping during pathologic processes39 and in synaptic remodeling.29
Another key difference between the function of microglia and that of other APCs is the interaction between microglia and neurons that leads to the inhibition of microglial cell activation. Microglia are the only mononuclear phagocytes that express neurotransmitter receptors, and they are rich in purinoceptors (e.g., dopamine receptors and adrenoreceptors). In the normal, healthy CNS, experiments using two-photon imaging have shown that microglia inspect neuronal synapses in vivo in the adult cerebral cortex.35 Neuron–microglial cell inhibitory signaling can be mediated via several mechanisms; these include contact-dependent inhibitory influences, such as CD172A-CD47,40 CD200-CD200R,41,42 CD22-CD45,42,43 and ICAM5-LFA1,44 as well as soluble inhibitory cytokines, such as CX3CL1-CX3CR1 interactions.45 Microglia sense damage once neuronal inhibition is removed or silenced ( Fig. 1.1 ).
In their homeostatic resting state, microglia express very low levels of MHC class II molecules46 and CD45.47 In the presence of inflammation or pathologic events, microglia become activated and undergo morphological and immunophenotypic alterations, including the upregulation of MHC class II molecules.48 Under these conditions, microglia secrete a diversity of chemokines, such as CCL1, CCL2, CCL5, and CXCL10, that function in the recruitment of lymphocytes and other APCs, such as macrophages and DCs. Evidence for the role of chemokines secreted by microglia in T-cell migration into the CNS parenchyma was provided by a study involving macrophage colony–stimulating factor (M-CSF). Microglial activation is impaired in M-CSF–deficient mice, which significantly reduced T-cell migration but did not affect axonal repair.49 Corroborating evidence for the importance of microglia-derived chemokines in T-cell migration was also provided by a study conducted by Heppner et al, which revealed that microglial paralysis inhibits the development and maintenance of inflammatory CNS lesions in the EAE mouse model.50

T Cells
Under normal physiologic conditions, the CNS tightly regulates cell entry into the parenchyma and localizes immunosurveillance to the perivascular and subarachnoid spaces. The CSF of normal healthy individuals contains approximately 0.15 × 106 T cells, of which greater than 80% are central memory CD4+ T cells (which express CD45RO+/CD27+/CCR7+/CXCR3+ and L-selectin).51 It has been suggested that the central memory CD4+ T cells present in the CSF are responsible for the routine immunosurveillance of the CNS, by searching for recall antigens presented by macrophages.26 It has been estimated that the T cells present in the CSF are replaced by newly immigrating lymphocytes approximately twice per day; this provides a continuous influx of lymphocytes that have different T-cell-receptor (TCR) specificities, which allows a rapid reaction to antigens that are present within the subarachnoid space.51–53
In various different neuropathologic conditions, T cells cross the BBB and participate in inflammatory reactions that are deleterious to the individual. One example is multiple sclerosis (MS), in which the presence of CD4+ T cells that display a proinflammatory Th1 or Th17 profile (along with other inflammatory factors) results in an autoimmune disease characterized by recurrent episodes of demyelination and axonal lesions. The cytokines secreted by Th1 (interferon-γ [INF-γ]) and Th17 (interleukin-17 [IL-17]) result in the different clinical forms of MS.54,55 In the EAE model, although Th1- and Th17-mediated lesions differ in their composition, there is no difference in the localization of lesions within the CNS. The Th17 cells present in lesions can convert to IFN-γ–producing Th1-like cells. There is also a difference between the lytic potential of Th1 and Th17 cells present at EAE lesions; the Th1 cells lysed astrocytes and fibroblasts that presented autoantigen, whereas the Th17 cells acquired cytotoxic potential only after conversion to the IFN-γ-producing Th1 phenotype.56 The presence of Th17 cell infiltrates in the brain has also been shown in the experimental mouse model of malignant glioma and in human gliomas.57
CD4+ regulatory T cells have also been shown to cross over the BBB and infiltrate the CNS in human glioblastoma multiforme,58 other astrocytomas,59 and viral infections such as West Nile virus infection,60 and their presence has been reported in the CSF of patients with MS.61 The presence of regulatory T cells in the peripheral tissues can serve to generate tolerance against self-antigens and thus control autoimmune reactions. The presence of high levels of CD4+ Tregs within the CNS in mouse models of glioma is indicative of poor survival, as shown by the increase in life span after the depletion of Tregs in this model.62 This finding remains to be corroborated for gliomas in humans.
Cytotoxic T lymphocytes (CTLs) facilitate the killing of specific target cells via the formation of the immunologic synapse, in which after antigen recognition, CD3/TCR complex is recruited to the area of contact between the CTL and the target cell and forms a central cluster. The T-cell cytoskeleton and the TCR-rich area undergo a series of alterations that result in the directed secretion of cytotoxic granules. A recent study demonstrates that infiltrating CTLs in glioblastoma can generate immunologic synapses with tumor cells.63 CD8+ T cells that establish immunologic synapses with glioma cells and express polarized granzyme B as an effector molecule may be critical for antitumor-mediated immunologic responses within the CNS in human glioblastoma.
Natural Killer (NK) Cells
Natural killer (NK) cells are recruited to the CNS in response to tissue injury. NK-cell depletion induces severe EAE.64 Impaired recruitment of NK cells is associated with increased mortality related to EAE, as well as spastic paraplegia and inflammatory lesions with hemorrhage. Some studies indicate that NK cells may limit autoimmune responses by killing DCs, regulating T-cell proliferation, or secreting regulatory cytokines, although it seems likely that NK cells differentially regulate inflammatory responses at various anatomic sites.
T-Cell Recruitment into the Central Nervous System
T cells can enter the CNS via several routes, including migration from the blood to the CSF across the choroid plexus or meningeal vessels into the subarachnoid space, migration from the blood to the parenchymal perivascular spaces of the brain, and migration from the blood to the parenchymal perivascular space of the spinal cord. These different migration routes involve the transport of the T cells across various barriers: the blood–CSF barrier, the BBB, and the blood–spinal cord barrier.51–53 Under inflammatory conditions, the expression of adhesion molecules and chemokines is induced on the endothelium at the BBB. This provides trafficking signals for circulating leukocytes to enter the CNS. When the endothelial BBB allows transendothelial migration, T-cell recruitment into the CNS is still highly regulated because the lymphocytes found in the perivascular spaces have a homogeneous effector memory pheno-type that distinguishes them from lymphocytes found in other inflamed tissues.51,65,66 T-cell migration into the CNS involves a three-step interaction between the leukocytes and the endothelium: (1) tethering and rolling of leukocytes on the BBB, (2) activation and firm adhesion, and (3) diapedesis (transendothelial migration). Each of these steps is described in detail below.
Tethering and Rolling of Leukocytes on Endothelium
The contact between vascular endothelium and circulating lymphocytes is the result of fluid dynamics within the blood vessel. The term leukocyte margination describes the phenomenon in which cells flowing within the blood vessel are positioned closer to the vascular wall than to the center of the vessel.67 Here, lymphocytes make a transient and reversible contact with the vascular endothelium. This contact is mediated by adhesion molecules of the selectin family and their carbohydrate ligands, initiating the rolling of leukocytes on the endothelial surface. This interaction, although not strong enough to anchor cells against the force of blood flow, allows cells to roll along the endothelium, continually making and breaking contact ( Fig. 1.2 ).
The microvessels of a healthy brain and spinal cord of SJL/N mice constitutively express vascular cell adhesion molecule-1 (VCAM-1), platelet endothelial cell adhesion molecule-1 (PECAM-1), and intercellular adhesion molecule-2 (ICAM-2). (The latter two molecules are also expressed on vascular endothelial cells at other sites.68) During inflammatory diseases such as EAE, tethering and rolling of leukocytes can be observed in superficial brain and meningeal microvessels by performing intravital microscopy.69 In inflamed murine vessels, CD8+ T cells have been shown to roll by adhering to the endothelium via P-selectin glycoprotein ligand-1 (PSGL-1), whereas CD4+ T cells roll via α4-integrin.70 P- and E-selectin can also mediate lymphocyte rolling in inflamed brain vessels.71,72 During EAE, there is upregulation of both VCAM-1 and ICAM-1 on the CNS endothelium, but not of P- or E-selectin.73 It has been suggested that the α4-integrin/VCAM-1 interactions mediate the steps of adhesion of lymphocytes to the BBB because antibodies that block α4-integrins or endothelial VCAM-1 significantly reduce the adhesion of lymphocytes to the brain vascular endothelium; however, they have little effect on transendothelial migration of autoreactive lymphocytes.74

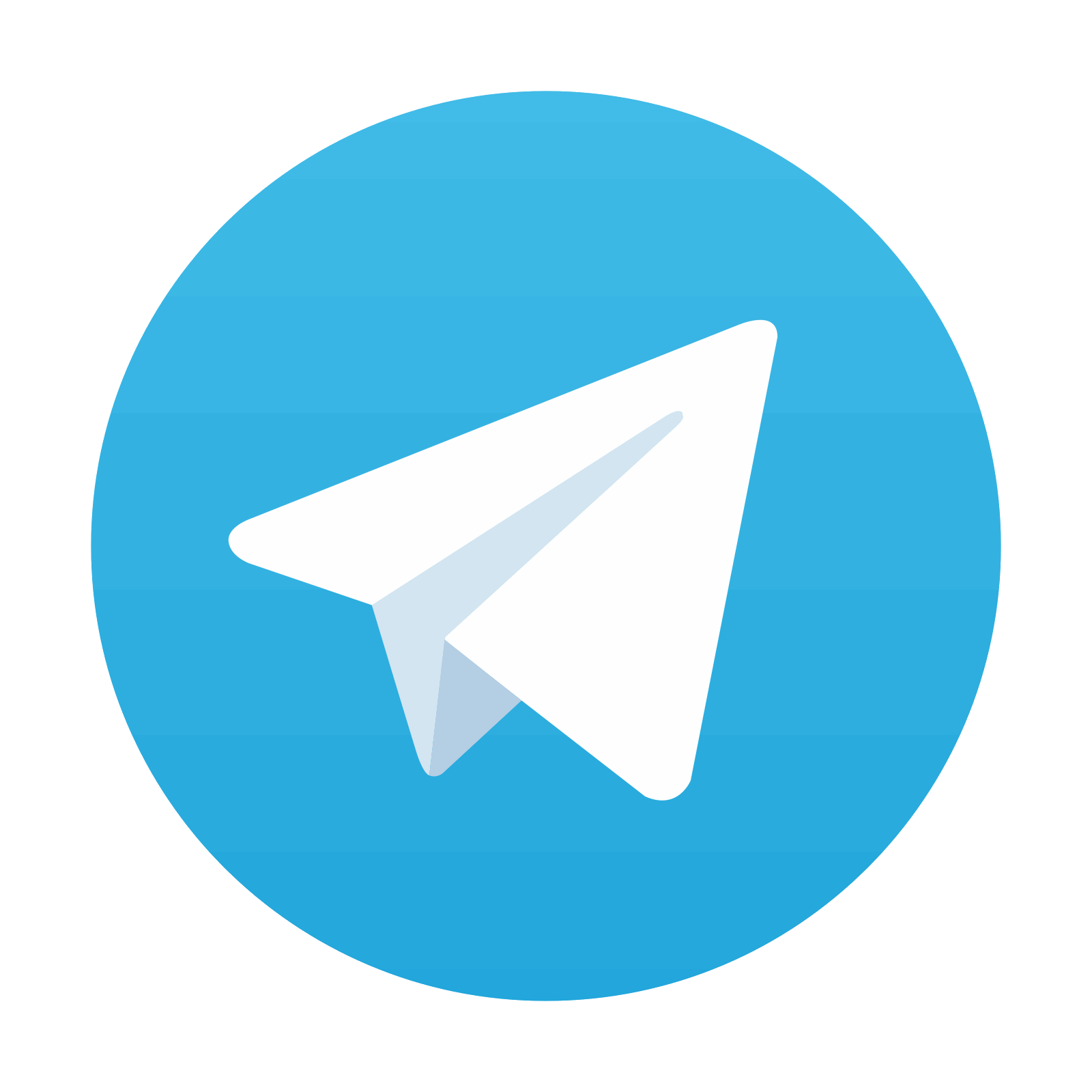
Stay updated, free articles. Join our Telegram channel

Full access? Get Clinical Tree
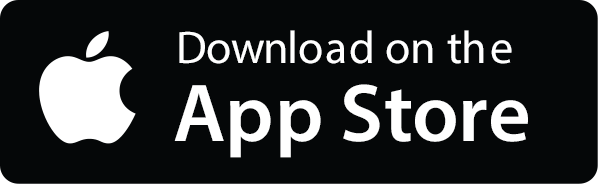
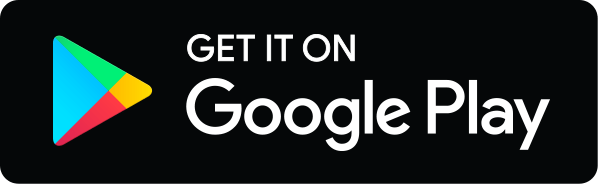
