Several immunostimulant approaches have been studied in the treatment of gliomas. The advent of recombinant DNA technology led to a nonspecific immunostimulation via systemic administration of cytokines. Recently, in attempts to more closely mimic their natural activity, cytokines have been delivered by implanting genetically transduced cells or by using in vivo gene transfer techniques. The latest efforts have focused on immunostimulatory agents that act directly on antigen-presenting cells and effector cells of the immune system via pattern recognition receptors. Combining these strategies with more than one mode of immunotherapy may provide better clinical results.
Despite advances in surgical-, radiation-, and chemotherapy-based strategies, malignant gliomas continue to be associated with a poor prognosis. Immunostimulants offer a novel treatment approach. This article reviews immunostimulant treatment approaches and understanding for malignant glioma and relevant clinical trials. Early attempts at glioma therapy based on immunostimulants failed to demonstrate effectiveness. Current immunostimulant therapies have shifted to a more multifaceted approach combining two or more different immunotherapeutic strategies. As understanding of the immune system’s role in brain cancer increases, continued efforts will move toward potentially more effective therapies.
Glioblastoma mutiforme (GBM) is challenging to treat and associated with a high degree of morbidity and mortality. Standard treatment consists of cytoreductive surgery followed by radiation therapy in combination with temozolomide chemotherapy followed by adjuvant temozolomide, which leads to a median survival of 14.6 months. Based on several meta-analyses, other types of adjuvant chemotherapy, mainly nitrosoureas, seem to add some survival benefit, but the gain is modest. Continuous efforts are ongoing to develop more novel, effective agents or combinations of agents that may improve overall survival or prolong time to progression.
Immunotherapy, and in particular immunostimulants (also known as biologic modifiers), are an example of an area of research into novel therapy for use in high- and low-grade gliomas. Immunotherapy, or treatment that uses the body’s immune system to combat tumors is attractive for cancer therapy for several reasons, including the conviction that it would be less toxic than the traditional cytotoxic therapies and may lead to sustained responses through immunologic memory. Nevertheless, various advances and promising preclinical results seen in both in vitro and animal models have not yet translated into positive human clinical trials for glioma, although these studies provide more insight and guidance for future studies. Consequently, a considerable interest in immunotherapy persists, and combined with accumulating evidence of the immune system’s role (or suppression) in the genesis of cancer, immunotherapy studies in glioma are ongoing and are increasingly frequent. This article highlights the use of immunostimulants in glioma. Other articles in this issue provide a further review of other forms of active immunotherapy and passive immunotherapy.
The immune system’s role in cancer genesis
The genesis of cancer and the genesis of immunosuppression are related. It is thought that under ideal circumstances, immune surveillance identifies neoplastic cells and subsequently destroys them. Tumor cells, however, manage to avoid detection by the immune system, and the cancer cells that avoid surveillance are able to proliferate and acquire additional immunoevasive mechanisms. As such, by the time the tumor is clinically apparent, it is adept at avoiding the immune system in various ways. For example, glioma patients have been found to have decreased T-cell responsiveness, T-cell receptor-mediated signaling, and antibody production. These immunosuppressive effects are mediated by tumor cells in numerous ways, including cytokine dysregulation. For instance, tumor-expressed cytokines like transforming growth factor beta (TGF-β), prostaglandin E2 (PGE2), and interleukin (IL)-10 suppress immune responses, and these cytokines are often overexpressed in glioma. Other immunosuppressive measures consist of down-regulating major histocompatability complex (MHC) molecules, thereby decreasing the body’s ability to see or recognize and destroy tumor. Immunostimulants as a cancer treatment are appealing, because boosting impaired tumor responses can in theory eradicate tumor by overcoming tumor-associated immunosuppression. The complexity of this process continues to be worked out, and a broader understanding of the immunosuppressive environment of the tumor, the low avidity of T-cells for tumor antigen expressed on tumor, and the poor immune response will lead to more effective therapies.
Immunostimulants
Immunostimulants are generally cytokines or chemokines. Not all immunostimulants or biologic modifiers, however, fall into this class of molecules. In fact, the first immunotherapy studies in glioma involved active immunization with agents such as bacilli Calmette-Guerin (BCG) and Imuvert, which is derived from Serratia marcescens bacterium. These studies did not yield positive clinical results for patients with gliomas, but they did serve as the foundation for future studies both in glioma and other cancers. Cytokines have been used in most further immunostimulant studies. Different T-helper cells (Th) secrete cytokines, the characteristics of which vary with the nature of immune response. Cytokines traditionally are divided into three classes:
The Th1 cytokine class, such as interferon (IFN)-γ, interleukin (IL)-2), and IL-12, promotes cell-mediated immune responses and are capable of exerting antitumor responses.
Th2 class cytokines such as IL-4 stimulate humeral responses or may be immunosuppressive like IL-10.
Cytokines in the Th3 class including the transforming growth factor (TGF) family are by and large immunosuppressive.
Immunostimulants may be delivered to patients intravenously or intratumorally to potentiate immune surveillance and cell-mediated antitumor responses. These responses can be divided into one of three categories of response:
- 1.
Exert direct toxic effects on the tumor
- 2.
Enhance tumor immunogenicity alone or in the setting of other therapies, immune-based or not
- 3.
Support depressed antitumor cell-mediated immune responses
Initial studies attempted systemic, intratumoral, or intrathecal delivery, but resulted in significant toxicity. Endeavors to reduce toxicity led to engineering cells, using gene transfer techniques, to secrete cytokines directly. These cells then can be transplanted into central nervous system (CNS) tumors and achieve production of cytokine without the need for systemic administration. More recent strategies use intratumoral delivery of engineered cytokine gene-bearing attenuated viral vectors, which have the advantage of delivering high viral titers into tumor with subsequent infection and cytokine secretion by tumor cells; this manner of viral vector delivery also obviates the concern of immune rejection of transplanted cytokine secreting cells. More recent attention has focused on cell-based cytokine delivery via neural stem cells (NSCs). NSCs are capable of intracranial migration and are tropic for migrating glioma, which invades brain parenchyma beyond the main tumor mass. Cytokine-secreting NSCs are therefore capable of tracking and delivering cytokines to areas of tumor beyond the surgical resection cavity, the radiation field, and perhaps beyond the distance that traditional chemotherapy can penetrate. This neural stem cell system is particularly attractive for glioma, which is a highly invasive and disseminated tumor, a characteristic that has limited treatment success to date.
Immunostimulants
Immunostimulants are generally cytokines or chemokines. Not all immunostimulants or biologic modifiers, however, fall into this class of molecules. In fact, the first immunotherapy studies in glioma involved active immunization with agents such as bacilli Calmette-Guerin (BCG) and Imuvert, which is derived from Serratia marcescens bacterium. These studies did not yield positive clinical results for patients with gliomas, but they did serve as the foundation for future studies both in glioma and other cancers. Cytokines have been used in most further immunostimulant studies. Different T-helper cells (Th) secrete cytokines, the characteristics of which vary with the nature of immune response. Cytokines traditionally are divided into three classes:
The Th1 cytokine class, such as interferon (IFN)-γ, interleukin (IL)-2), and IL-12, promotes cell-mediated immune responses and are capable of exerting antitumor responses.
Th2 class cytokines such as IL-4 stimulate humeral responses or may be immunosuppressive like IL-10.
Cytokines in the Th3 class including the transforming growth factor (TGF) family are by and large immunosuppressive.
Immunostimulants may be delivered to patients intravenously or intratumorally to potentiate immune surveillance and cell-mediated antitumor responses. These responses can be divided into one of three categories of response:
- 1.
Exert direct toxic effects on the tumor
- 2.
Enhance tumor immunogenicity alone or in the setting of other therapies, immune-based or not
- 3.
Support depressed antitumor cell-mediated immune responses
Initial studies attempted systemic, intratumoral, or intrathecal delivery, but resulted in significant toxicity. Endeavors to reduce toxicity led to engineering cells, using gene transfer techniques, to secrete cytokines directly. These cells then can be transplanted into central nervous system (CNS) tumors and achieve production of cytokine without the need for systemic administration. More recent strategies use intratumoral delivery of engineered cytokine gene-bearing attenuated viral vectors, which have the advantage of delivering high viral titers into tumor with subsequent infection and cytokine secretion by tumor cells; this manner of viral vector delivery also obviates the concern of immune rejection of transplanted cytokine secreting cells. More recent attention has focused on cell-based cytokine delivery via neural stem cells (NSCs). NSCs are capable of intracranial migration and are tropic for migrating glioma, which invades brain parenchyma beyond the main tumor mass. Cytokine-secreting NSCs are therefore capable of tracking and delivering cytokines to areas of tumor beyond the surgical resection cavity, the radiation field, and perhaps beyond the distance that traditional chemotherapy can penetrate. This neural stem cell system is particularly attractive for glioma, which is a highly invasive and disseminated tumor, a characteristic that has limited treatment success to date.
Cytokines
Cytokine therapy for cancer has received considerable attention. The initial use of cytokines used systemic recombinant therapy with the intent of delivering a high, potent dose to stimulate tumoricidal responses. This strategy worked in mouse models but was limited in people because of toxicity and short half-lives. In regard to primary brain tumors, the use of cytokine therapy must afford for the blood–brain barrier and the privileged state of the CNS. Given these challenges, some studies have focused on local delivery or intratumoral delivery of cytokines, but these studies also often resulted in significant toxicity.
Particular to glioma, attention has focused on the promotion of T-cell activity by using IL-2, IL-4, IL-12, IFN-α, and IFN-γ. IFN-γ also may inhibit tumor induced neovascularization. Cytokines like tumor necrosis factor (TNF)-α have been used to induce up-regulation of cell surface MHC, thereby increasing visibility of tumor to the immune system. TNF-α associated chemokine, TRAIL (TNF-related apoptosis inducing ligand) also has been used to induce tumor cell death by triggering apoptotic cascades within cancer cells only. Further discussion of the most common cytokines used in glioma studies to date follows.
IL-2
IL-2 was among the first cytokines to be studied in glioma. IL-2 is an important T-cell growth factor involved in the proliferation of CD8+ T-cells and blockade of the immunosuppressive effects of transforming growth factor (TGF)-β. High systemic doses are required to achieve effective levels in the brain for therapy against brain tumors. Early, small clinical trials using IL-2 for patients with glioma, generally in combination with lymphokine-activated killer cells (LAK), did not have positive results. These trials involved systemic, intrathecal, and intratumoral administration of IL-2 but resulted in significant toxicity, including cerebral edema, which was caused by IL-2 increasing vascular permeability. Other trials used IL-2 with IFN-α but had similar negative results and toxicity.
Further preclinical studies concentrated on different delivery methods. For example, in one study, allogeneic fibroblasts were engineered to secrete interleukin IL-2 and subsequently administered either subcutaneously or intracerebrally to mice with intracranial glioma. This treatment led to a significant prolongation of survival in mice with intracranial glioma treated intracerebrally relative to the survival of mice with treated subcutaneously. Other similar studies have shown a cytotoxic antitumor response that leads to significant prolongation of survival in mice challenged with melanoma. More recent studies demonstrated that local IL-2 therapy in the brain not only generates an immediate local antitumor immune response, but also establishes long-term immunologic memory capable of eliminating subsequent tumor challenges within and outside of the CNS. IL-2 also has been combined with traditional cytotoxic chemotherapy in a study that showed that the combination of paracrine immunotherapy, with nonreplicating genetically engineered tumor cells that produce IL-2, and local delivery of chemotherapy by biodegradable polymers prolonged survival in a synergistic manner in mice challenged intracranially with murine brain tumor. Finally, an injectable polymeric system for the long-term localized delivery of IL-2 has been studied in the rat brain; antitumor efficacy studies performed in brain tumor models in rats appeared promising and indicative of long-lasting immunity.
More recent studies in human patients used IL-2 as a transgene and in combination with HSV-tk transgene; the genes were carried by a retroviral virus on vector-producing cells. Twelve patients with recurrent GBM were treated with an intratumoral injection of the construct followed by systemic administration of acyclovir. The treatment was tolerated well, and several patients had radiographic responses. Another clinical trial in 40 patients with recurrent GBM performed by Dillman and colleagues used IL-2 to generate autologous LAKs. After generating LAK, these cells were injected intratumorally. This approach was tolerated well, and a clinical benefit was seen, with a median survival of 9 months, and a 1-year survival rate of 34%. Further studies for both the aforementioned clinical trials are being considered.
IL-4
IL-4 overexpression may impair tumorigenicity of glioma, and several studies have determined that IL-4 may have a role in tumor treatment. For example, the ability of IL-4 to mediate an antitumor response to human gliomas was studied in nude mice using a transfected tumor cell line expressing IL-4. This study showed a significant inhibition of growth of U87 human glioma cells, with a prolongation of survival when compared with control nude mice. Histologic analysis revealed the presence of an eosinophil infiltrate and tumor necrosis.
Other evidence of the antitumor effect of IL-4 was provided after in vivo gene transfer into malignant gliomas. In this study, GBM cells and retroviral producer cells, secreting 20 ng and 50 ng of IL-4 were implanted into rats. Several rats were cured of their tumors and developed an immunologic memory and thus rejected a rechallenge with tumor cells. Immunohistology showed inflammatory infiltrates in IL-4-treated tumors in which CD8+ T-lymphocytes were more abundant, although CD4+ T-lymphocytes, B-lymphocytes, and macrophages were also present. Another study found that implantation of gliosarcoma cells expressing IL-4 into rats induced a specific, protective, immune response against rechallenge with tumors and suggested that tumor-specific reactivity among CD4+ T-cells was critical for developing tumor resistance. Other findings support the use of tumor cell vaccines expressing IL-4. Benedetti and colleagues have described the use of IL-4 secreting neural stem cells for treating mice with intracranial gliomas, and results are indicative of promise, although more work needs to be done. Overall, these findings suggest that IL-4 gene transfer is a new, promising approach for treating malignant gliomas. Interestingly, it also has been shown that dexamethasone significantly reduced the antitumor effect of IL-4. This result is relevant to patients with brain tumors who often take steroids for cerebral edema.
IL-12
IL-12 is a cytokine that promotes an antitumor Th1-type pattern of differentiation in naïve T-cells. Recent studies also have found that IL-12 is needed to overcome the immunosuppressive effects of TGF-β. A recent, extensive review of immunotherapy by IL-12 based cytokine combinations also describes several other potential effects of IL-12 on the immune system.
Despite its therapeutic success in multiple animal cancer models, the utility of systemically administered recombinant IL-12 has been limited by its toxicity. This has encouraged the development of local IL-12 delivery systems through gene transfer. Using such techniques and direct intratumoral administration of IL-12 into mice with gliomas, researchers demonstrated that survival was prolonged significantly, and immunohistochemistry demonstrated robust CD4+ and CD8+ T-cell infiltration in these mice compared with the two control groups. Another study demonstrated that local delivery of IL-12 into the rat brain by genetically engineered gliosarcoma cells significantly prolonged survival time in animals challenged intracranially with a malignant glioma. Survivors appeared to develop an antitumor immunologic memory, as surviving rats were immune to rechallenging with a second injection of tumor cells. Other studies have shown the potent antitumor effects observed for paracrine gene-delivered administration of IL-12 in multiple tumor cell types and in multiple murine strains. Interestingly, IL-12 secreting neural stem cells also have been studied preclinically, and it has been shown that these cells exhibit tropism for tumor and result in long-term survival.
Kikuchi and colleagues conducted a study with IL-12 that included 15 people varying gliomas. Treatment consisted of subcutaneous administration of recombinant IL-12 in combination with a fusion of autologous dendritic and glioma cells. Augmentation of CD8+ cytolytic activity on glioma cells was documented after treatment, and there was a modest radiographic response.
Granulocyte–macrophage colony-stimulating factor
Granulocyte-macrophage colony-stimulating factor (GM-CSF) is secreted by macrophages, cells, mast cells, endothelial cells, and fibroblasts. GM-CSF stimulates stem cells to produce granulocytes (neutrophils, eosinophils, and basophils) and monocytes. Monocytes exit the circulation and migrate into tissue, whereupon they mature into macrophages. It is thus part of the immune/inflammatory cascade, by which activation of a small number of macrophages can rapidly lead to an increase in their numbers, a process crucial for fighting infection.
GM-CSF exerts its antineoplastic effects by producing a cytotoxic T-cell response. GM-CSF also induces the recruitment of antigen-presenting cells and may activate antigen presentation pathways for MHC class 1-mediated processes. Most studies relevant to glioma have used GM-CSF to derive dendritic cells by exposing monocytes to GM-CSF. GM-CSF also has been shown to stimulate intracranial responses to irradiated tumor cell vaccines. Clinical studies with GM-CSF as an immunotherapy for gliomas are limited, but GM-CSF may serve as a useful vaccine adjuvant with limited toxicity in human clinical trials of antiglioma vaccine therapy. In fact, it is being used currently as an adjuvant in a phase 2 study of CDX-110 in patients with newly diagnosed GBM. Objectives of the study are to investigate the anticancer activity, impact on survival, and safety of CDX-110 plus GM-CSF when administered concurrently with temozolomide and continuing until disease progression. Of note, CDX-110 is a vaccine-based therapy that targets the tumor-specific molecule called EGFRvIII, a functional variant of the epidermal growth factor receptor (EGFR), a protein that has been validated as a target for cancer therapy. Unlike EGFR, EGFRvIII is not present in normal tissues, suggesting this target will enable the development of a tumor-specific therapy for cancer patients. More information is available at: http://www.celldextherapeutics.com/wt/page/cdx_110 .
TNF-α
TNF-α, also known as cachexin or cachectin, is a cytokine involved in systemic inflammation, and it is a member of a group of cytokines that stimulate the acute phase reaction. The primary role of TNF-α is in the regulation of immune cells. TNF-α is also able to induce apoptotic cell death, to induce inflammation, and to inhibit tumorigenesis and viral replication. Dysregulation and, in particular, overproduction of TNF, has been implicated in various human diseases, including cancer. Built on proof of concept from prior studies, a 2009 study of retroviral vector-mediated TNF-α given with IFNs (see section on IFN) increased tumor antigen expression through the up-regulation of MHC class 1 and 2 molecules. Tumor regression was seen in animal models, but the benefit to people remains uncertain. It is worth mentioning that glioma-bearing mice deficient in TNF-α developed larger tumors and had reduced survival compared with their wild-type controls.
Miscellaneous cytokines
Several other cytokines have been used in glioma studies. For example, recent studies have incorporated the Flt3L ligand, which can expand dendritic cells and enhance immunogenicity and can promote tumor death when given with other immunotherapies. Further studies on this novel cytokine demonstrate that Flt3L has distinct effects on dendritic cell development, suggesting an important role for Flt3L in generating dendritic cells that have tolerogenic effects on T-cells.
IL-21 also has received relatively recent interest. IL-21 has potent regulatory effects on cells of the immune system, including natural killer cells and cytotoxic T-cells that can destroy virally infected or cancerous cells. Preclinical studies have focused on gene-modified cell delivery and generally in tandem with other cytokines. Interestingly, researchers discovered that IL-21 is produced by Hodgkin’s lymphoma (HL) cells (which was surprising, because IL-21 was thought until then to be produced only by T-cells). This discovery may explain a great deal of the behavior of classical HL, including clusters of other immune cells gathered around HL cells in cultures. Targeting IL-21 may be a potential treatment or possibly a test for the disease.
IFN
INFs are glycoproteins, which are cell-signaling molecules produced by the cells of the immune system in response to challenges such as viruses and tumor cells. IFNs assist the immune response by inhibiting viral replication within host cells, activating natural killer cells and macrophages, increasing antigen presentation to lymphocytes, and inducing the resistance of host cells to viral infection. Early preclinical studies with IFN-α and IFN-β showed promise, but these clinical studies suffered from poor design and lack of strict patient eligibility and selection. Imaging analysis and tumor grading also made it difficult to appropriately assess efficacy.
IFN-α
IFN-α is associated with accumulation of CD4+, CD8+, and dendritic cells within the established tumor, demonstrating induction of antitumor immune responses. Preclinical testing has led to various clinical trials. For example, IFN-α was tested in a phase 3 clinical trial for patients with recurrent high-grade gliomas. The study included 214 patients with anaplastic astrocytoma, anaplastic oligiodendroglioma, GBM, and gliosarcoma. The patients initially were treated with radiation and BCNU (carmustine). Following radiotherapy, patients without disease progression were stratified and randomized into two groups: BCNU and BCNU plus IFN-α. Unfortunately, there was no difference in response rates between the two treatment arms in the final evaluation. A recent phase 1 clinical trial combined IFN-α with BCNU wafers in patients with recurrent GBM. Patients were treated with surgical resection and implantation of BCNU wafers; then a week later, IFN was initiated three times a week at a dose of 3 mU/m(2), which was escalated in increments of 3 mU/m(2). Dose-limiting toxicity in the form of fatigue occurred at 9 mU/m(2). There were nine evaluable patients, and two had complete imaging responses. Further studies are in the planning stages.
IFN-β
IFN-β exerts its antitumor effect by inhibiting glioma in the S phase, enhancing natural killer cell and cytotoxic T-cell activity, and perhaps synergizing with cytotoxic chemotherapies. IFN-β has been delivered successfully to the brains of mice using bone marrow cells. A small phase 1 study of seven patients in 1989 used recombinant IFN-β in escalating dosages intravenously three times weekly. There was no evidence of response in any patient, either on clinical examination or by computerized tomography (CT), and four patients had immediate progression of disease. Another phase 1 study administered IFN-β intrathecally three times weekly to 20 patients with recurrent malignant gliomas. Adverse effects occurred in only one patient and consisted of nausea, vomiting, fever, and chills. Problems with the Ommaya reservoir (obstruction in two patients and infection in four patients) led to six patients being terminated from the study. Although this was primarily a study of toxicity, of 12 evaluable patients, 3 had stable disease for 148, 192, and 539 days, respectively; 9 had progressive disease. Other similar clinical studies have yielded mixed results. A Japanese cooperative group has just opened a multicenter phase 1 clinical trial, the Integrated Japanese Multicenter Clinical Trial: A phase I study of interferon-beta and temozolomide for glioma in combination with radiotherapy (INTEGRA Study). This study is being conducted for patients with high-grade glioma to evaluate the safety, feasibility, and preliminary clinical effectiveness of the combination of IFN-β and temozolomide. The primary endpoint is toxicity, and secondary endpoints are progression-free survival time and overall survival time. In addition, objective tumor response will be evaluated in a subpopulation of patients with the measurable disease. Planned enrollment is 10 newly diagnosed and 10 recurrent patients.
IFN-γ
IFN-γ causes increased major histocompatability complex expression on brain cells, class 1 antigen on local endothelial and ependymal cells, and class 2 antigen on microglial, ependymal, and perivascular cells. It also recruits lymphocytes and other inflammatory cells.
IFN-γ has demonstrated tumoricidal effects in various cancers. Preclinical studies show that IFN-γ produces an antiglioma response mediated predominantly by natural killer/lymphokine-activated killer cells. Studies have made use of in situ adenoviral-mediated IFN-γ gene transfer in glioma-bearing rodents and have found that survival was prolonged. These effects were accompanied by significant up-regulation of tumor MHC-1 and MHC-2 expression. In addition, therapy down-regulated the expression of endothelial Fas ligand, a cell membrane protein implicated as a contributor to immune privilege in cancer. Other studies using IFN-γ secreting allogeneic cells showed that the survival of mice with glioma injected with the cells was prolonged significantly relative to the survival of mice receiving equivalent numbers of glioma cells alone. A clinical trial in pediatric high-grade glioma patients treated with IFN-γ and low-dose cyclophosphamide after radiation has been performed. Forty patients were enrolled (median age: 8.5 years). The median overall survival of 1 year was not significantly different from a historical control group.
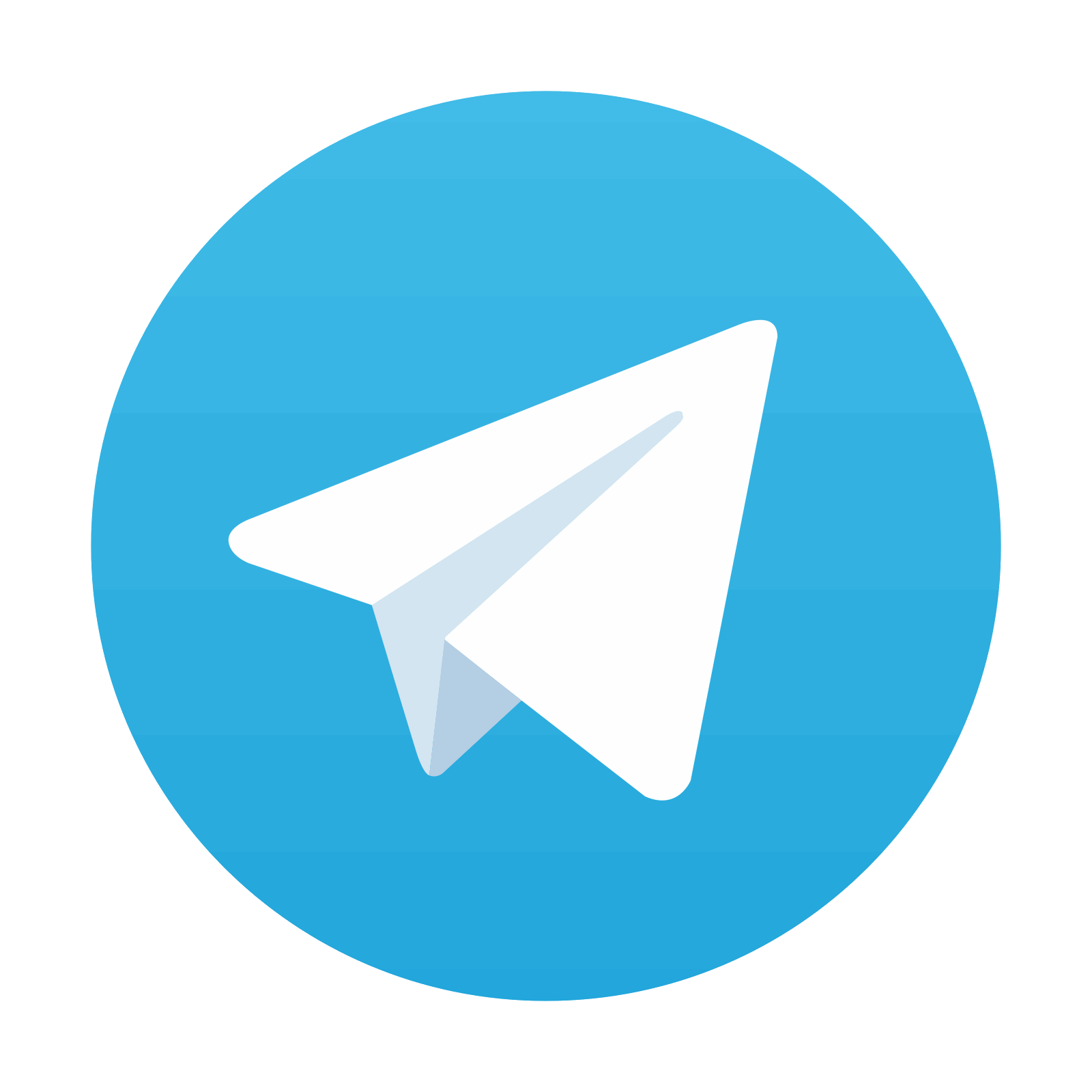
Stay updated, free articles. Join our Telegram channel

Full access? Get Clinical Tree
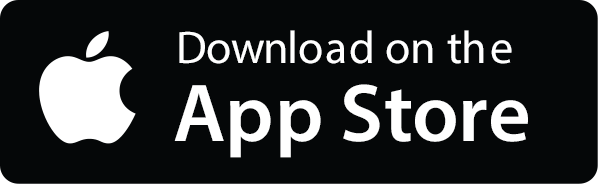
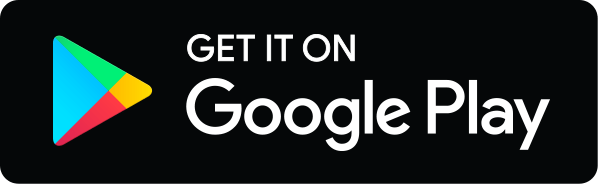