Fig. 25.1
Local administration of Ad-TK+Ad-Flt3L enhances the therapeutic efficacy of systemic antitumor DC vaccines. (a) DC vaccines were obtained from bone marrow precursors incubated in the presence of Flt3L, then they were loaded with Ad-TK+GCV-induced apoptotic tumor cells and activated with the TLR9 agonist CpG. DCs were administered subcutaneously in rats bearing large (day 10) intracranial CNS-1 tumors. DC vaccination was repeated 8 days later. Tumor-loaded DCs migrate to the lymph nodes and trigger an antitumor immune response that leads to tumor regression in ~10% of the animals. (b) Combined conditional cytotoxic/immune stimulatory gene therapy was performed by intratumoral injection of Ad-TK+Ad-Flt3L, administered in a single dose 10 days after tumor implantation. Ad-TK-induced tumor cell death leads to release of tumor antigens and intracellular inflammatory molecules, such as TLR2 agonist HMGB1, which activate DCs recruited upon Ad-Flt3L expression. Tumor-loaded DCs migrate to the draining lymph nodes and trigger an antitumor immune response that leads to tumor regression in ~50% of the animals (c) Kaplan–Meier survival curves of rats treated in situ with Ad-TK+Ad-Flt3L in combination with systemic DC vaccines. *, p < 0.05 vs. saline, ^ p < 0.05 vs. Ad-TK+Ad-Flt3L alone (Log rank test). Note that in situ immunogene therapy in combination with systemic DC vaccination significantly enhances the therapeutic efficacy of either therapy alone, leading to brain tumor regression and long-term survival in about 90% of animals
An important feature of gene therapy vectors to be injected into the brain is to exhibit a safe neuropathological profile. Since gene therapy vectors designed to treat GBM patients are injected into the tumor bed after surgical resection, preclinical assessment of the neuropathology in naïve brain parenchyma is crucial in the evaluation of translational gene therapies for GBM. Intracranial injection of Ad-Flt3L and Ad-TK into the naïve brain of rats and dogs exhibits a very safe neuropathological profile, with mild acute local inflammation and without apparent signs of neurotoxicity (Candolfi et al. 2012). Nevertheless, the use of inducible promoters such as the tetracycline-responsive element (TRE), which depends on the presence of the antibiotic tetracycline or its analogs, allows control of transgene expression by withdrawing the inducer if putative side effects develop (Curtin et al. 2008). Flt3L expression can be tightly regulated using the TRE promoter in murine, canine, and human GBM cells (Curtin et al. 2008). Expression of therapeutic transgenes under the control of inducible promoters further increases the safety of this therapeutic approach.
Type I IFNs
The delivery of IFN-α has been evaluated as a therapeutic method for the treatment of GBM. In view of its effects on antitumor immunity, angiogenesis, and tumor cell proliferation and death, IFN-α emerges as a very attractive target for GBM therapy. IFN-α acts as an antiangiogenic factor in preclinical intracranial GBM models, it directly inhibits the proliferation of GBM cells, and sensitizes them to pro-apoptotic factors. IFN-α is also a powerful stimulant of the host immune system, as it activates dendritic cells, upregulates MHC-I and –II expression, recruits immune cells into the tumor mass and enhances cellular and humoral immune responses. Although intratumoral administration of Ad-IFN-α alone does not have therapeutic benefit in intracranial rat CNS-1 tumors, its combination with Ad-TK leads to tumor regression and long term survival in approximately 50% of the animals (Candolfi et al. 2012). Administration of IFN-β has also been explored as a therapeutic strategy for GBM. Administration of IFN-β gene therapy as a single therapeutic agent did not improve the survival of mice bearing intracranial GL261 GBM (Saito et al. 2004). However, combination of IFN-β gene therapy with vaccination with tumor cell lysate-pulsed dendritic cells resulted in long term survival in 50% of treated mice (Saito et al. 2004). Sequential intratumoral delivery of Ad-IFN-α and bone marrow-derived dendritic cells led to CD8+ T-cells-dependent tumor regression in 50% of GL261 tumor-bearing mice (Tsugawa et al. 2004). DCs injected intratumorally migrated to the cervical lymph nodes, where specific cytotoxic T lymphocyte (CTL) activity was detected, suggesting that this combination therapy exerts a specific antitumor immune response.
Although IFN-α was the first cytokine to be approved for cancer treatment, its considerable toxicity has limited its use. Administration of Ad-IFN-α into the naïve rat brain leads to overt local inflammation with loss of brain tissue, necrosis, and hemorrhages, as well as systemic side effects (Candolfi et al. 2012), making this vector unsuitable for intracerebral administration. However, the use of inducible or cell type-specific promoters may improve the safety profile of this approach, as they allow temporal and topographic control of transgene expression. A dual glial-specific and hypoxia-induced promoter has been recently described (Kim et al. 2011). In this dual specific vector transgene, expression is controlled by the nestin intron 2-SV40 promoter and erythropoietin enhancer for glial cell and hypoxia specific gene expression (Kim et al. 2011). Radio-inducible promoters are also useful tools to limit the toxicity of this approach. The radio-responsive early growth response gene 1 promoter has been used to drive the expression of cytotoxic molecules, such as TRAIL allowing for spatially and temporally controlled transgene expression (Tsurushima et al. 2007).
IL-2
IL-2 is a Th1 cytokine released from helper CD4+ T-cells that enhances CTL-mediated anti-tumor immunity, which makes it an attractive target for immunotherapy of gliomas. However, its systemic administration is limited by the toxicity of this molecule, and local delivery of IL-2 requires multiple or continued intracranial administration. Gene therapy vectors are useful tools for the long term delivery of this cytokine in the brain. Delivery of IL-2 using gene therapy vectors has been combined with proapoptotic strategies in order to promote anti-glioma immunity. Plasmids and vaccinia viruses encoding IL-2 and the proapoptotic molecules p53 and/or Bax were administered intratumorally in C6 rat gliomas in nude mice (Haghighat et al. 2000). Although these treatments showed antitumor activity without apparent side effects, the animal model used to evaluate this therapy has the vital limitation of lacking an intact immune system.
An adenoviral vector encoding IL-2 for the treatment of the experimental RG2 rat GBM model in combination with Ad-TK and Ad-Flt3L has also been used (Mineharu et al. 2012). Rats bearing intracranial RG2 tumors constitute a challenging GBM model, since these tumors have been shown to be refractory to most therapeutic approaches, including radiation, chemotherapy, and immunotherapy (Mineharu et al. 2012). Overexpression of IL-2 within the RG2 tumor microenvironment stimulates the recruitment of effector T-cells and reduces the intratumor infiltration of Tregs, enhances CTL-mediated antitumor immune responses, and successfully extends the median survival of RG2 tumor-bearing rats when combined with Ad-Flt3L+Ad-TK.
Other Cytokines
Another strategy for the delivery of therapeutic transgenes into brain tumors is the use of neural stem cells (NSC), which have been reported to display similar migratory activity to that of GBM cells within the brain parenchyma (Aboody et al. 2000). NSCs have been used to deliver pro-inflammatory cytokines such as IL-4 and IL-12 into intracranial gliomas in vivo, leading to tumor regression in mouse and rat models of GBM (Vetter et al. 2009). NSCs exhibit tropism for disseminating glioma cells, and IL-12-secreting NSC therapy has been linked to enhanced T-cell infiltration in tumor microsatellites, promoting antitumor immunity (Vetter et al. 2009). Tumor rejection was shown to be dependent on CD8+ T-cells (Vetter et al. 2009). Although neurotoxicity remains to be evaluated, local grafting of NSC emerges as a useful tool to deliver therapeutic cytokines for the treatment of GBM.
Gene therapy-mediated overexpression of proinflammatory cytokines can also be exploited in order to enhance the efficacy of antiglioma vaccines. 9L rat GBM tumor cell vaccines engineered to produce IL-4 and TK were employed to treat intracranial GBM (Okada et al. 1999). Subcutaneous immunization of rats with nonirradiated 9L-IL-4 cells or 9L-IL-4-TK cells followed by treatment with GCV protected rats from subsequent intracranial implantation of wild-type 9L tumors (Okada et al. 1999). Treatment of small but established (day 3) intracranial 9L tumors induced cellular antitumor immunity and promoted long-term survival (>100 days) in 25–45% of the rats (Okada et al. 1999), indicating that gene therapy-mediated engineering of antitumor vaccines may enhance antitumor immunity.
Blockade of Local Immunosuppressive Targets
TGF-β
One of the major immunosuppressive molecules produced by GBM cells is Transforming Growth Factor-β (TGF-β), which inhibits T and B cell proliferation and activation, reduces the secretion of pro-inflammatory molecules, and downregulates the expression of MHCII in GBM cells. In order to overcome the immune evasion of GBM, blockade of TGF-β activity has been attempted using several gene therapy approaches. Systemic delivery of TGF-β antisense oligonucleotides has been performed using polybutyl cyanoacrylate nanoparticles in rats bearing intracranial F98 GBM, in order to improve the antitumor immune response induced by peripheral immunization with tumor cells infected with Newcastle Disease Virus (Schneider et al. 2008). Although this treatment did not lead to long term survival, it improved median survival of tumor-bearing rats and led to increased levels of activated T-cells, suggesting that blockade of TGF-β may have therapeutic value as an adjuvant to immune-stimulant strategies.
Another strategy to block TGF-β activity is to overexpress soluble TGF-β receptors, which compete for the binding of the ligand to its receptor, abrogating TGF-β effects. Delivery of soluble TGF-β receptors using adenoviral vectors delays the growth of intracerebral human GBM xenografts in nude mice in vivo (Naumann et al. 2008). Although in this tumor model the immune effects of this strategy cannot be assessed, the direct antitumor effects of TGF-β blockade warrant further experimentation using this vector in relevant syngeneic murine models of GBM. Blocking TGF-β activity by overexpressing decorin, a small proteoglycan that binds and inactivates TGF-β, using adenoviral vectors in the CNS-1 rat GBM model has been attempted (Biglari et al. 2004). Decorin expression was controlled by the ubiquitous hCMV promoter and the glial cell-specific GFAP promoter. Decorin overexpression slows glioma progression in vivo (Biglari et al. 2004). Decorin overexpression also reduces TGF-β bioactivity and expression in human cells in vitro and increases the local infiltration of activated T-cells in vivo in preclinical GBM models (Stander et al. 1998). Taken together, these findings indicate that gene therapy-mediated delivery of decorin may prove a useful adjuvant for the treatment of GBM.
FasL
Another mechanism by which GBM cells are thought to evade the immune system is the expression of FasL. Expression of FasL has been detected in human and experimental GBM and has been shown to support the growth of experimental intracranial gliomas. FasL expression has been detected in specimens of human GBM and negatively correlates with the degree of intratumoral CD4+ and CD8+ T-cell infiltration. FasL expression in human GBM is detected in tumor cells and endothelial cells of the tumor blood vessels, which has been proposed as a mechanism to deplete tumor infiltrating Fas+ T-cells. Although an Ad vector encoding FasL (Ad-FasL) exerts cytotoxic effects in CNS-1 cells in vitro and in vivo, its administration in combination with the immune-stimulant Ad-Flt3L fails to induce tumor regression in rats bearing intracranial GBM (Candolfi et al. 2009).
Knock down of FasL expression using shRNA in rat GBM cells reduced their ability to induce T-cell apoptosis and increased tumor infiltration of T-cells, leading to an inhibition of intracranial tumor growth (Jansen et al. 2010). Mice bearing intracranial GL26 tumors have been shown to double the expression of FasL when compared to tumors growing in the flank (Badie et al. 2001). These findings were attributed to the expression of FasL in microglia, which is absent in subcutaneous tumors (Badie et al. 2001). Blockade of FasL activity in GL26 GBM growing in the brain altered the local immunosuppressive milieu, increasing the infiltration of leukocytes (Badie et al. 2001). These findings indicate that blockade of FasL expression may improve the response of GBM to immunotherapeutic approaches.
STAT3 Signaling Involvement in Glioma Immunotherapies
The STAT3 transcription factor has proven to be a fundamental component of tumor growth and progression. Multiple growth factors and cytokines frequently overexpressed in cancer such as EGF, FGF and IL-6 activate Janus kinase 2 (JAK2). When activated, JAK2 phosphorylates STAT3 at its Tyr705 residue. Phosphorylated STAT3 translocates to the nucleus where it activates the transcription of genes which promote the evasion of apoptosis, cell division, angiogenesis, invasion, and metastases. Histopathological analysis of brain tumors found STAT3 to be overexpressed in 53% patients with grade III anaplastic astrocytomas, grade IV GBMs, or gliosarcoma but not in patients with low grade tumors. STAT3 levels correlated with the infiltration of T-cells and median survival time of tumor bearing patients (Abou-Ghazal et al. 2008). Expression of the DNA repair enzyme MGMT was directly influenced by the presence of STAT3. Inactivation of STAT3 by small hairpin RNA leads to a marked sensitivity to the alkylating agent temozolomide (Kohsaka et al. 2012). Preclinical studies using dominant negative vectors, small molecule inhibitors, peptidomimetics, and decoy antisense oligonucleotides have convincingly demonstrated that aberrant STAT3 signaling is crucial for the survival and growth of various brain tumor types (Doucette et al. 2012).
STAT3 has also been shown to be an important mediator of immune suppression. Tumors frequently secrete factors that inhibit the maturation and activation of multiple immune lineages. The production of pro-inflammatory cytokines such as TNF-α, IL-6, RANTES, IFN-Β, and IP-10 from multiple tumor lines has been shown to be controlled by STAT3 (Wang et al. 2004). The presence of conditioned media from v-src transformed 3T3 cells in cultured dendritic cells promotes a tolerogenic profile (low MHC-II, CD80, CD86 expression, low IL-12 release) which can be reversed by disruption of STAT3 (Wang et al. 2004). STAT3 is also believed to mediate the inflammatory response in humans diagnosed with GBM. Peripheral and tumor infiltrating macrophages isolated from resected tumors produce elevated levels of pro-inflammatory cytokines when treated with a small molecule inhibitor of STAT3 lending support to the idea that STAT3 signaling is responsible for the anti-inflammatory process and associated immune-suppression (Hussain et al. 2007).
Owing to the multiple roles of STAT3 in the survival and expansion of tumors, various groups have tried to use STAT3 inhibitors to treat tumors. Kortylewski et al. (2005) have shown that deletion of STAT3 in hematopoietic cells can strengthen the immune response of several different hematopoietic lineages and restrict the growth of B16 melanoma tumors. Tumor infiltrating T-cells isolated from B16 tumor-bearing STAT3 null mice produced an abundance of IFNγ relative to wild type mice. In addition, NK cells had increased cytolytic activity. In a different tumor model, improved inhibition of tumor growth was observed after vaccinating with STAT3 deficient DCs (Iwata-Kajihara et al. 2011). In addition to an increase of IL-12, IFNγ ELISPOT assays indicated a stronger immune response in STAT3 null mice. Adoptive T-cell transfer is another way of inducing an immune response against experimental tumors. Ablating STAT3 in T-cells prior to their transfer resulted in robust antigen-specific tumor T-cell activity and growth inhibition in B16 tumors (Kujawski et al. 2010). The ability of STAT3 to conduct a diverse set of immune suppressive instructions makes it an attractive potential target as adjuvant therapy with tumor immunotherapy. Although compounds such as AZD1480 and WP1066 hold promise as potential inhibitors, they await clinical confirmation. With the use of structure-activity relationships that facilitate the screening of large libraries of compounds, the stage is set for the development of safe and efficacious STAT3 inhibitors.
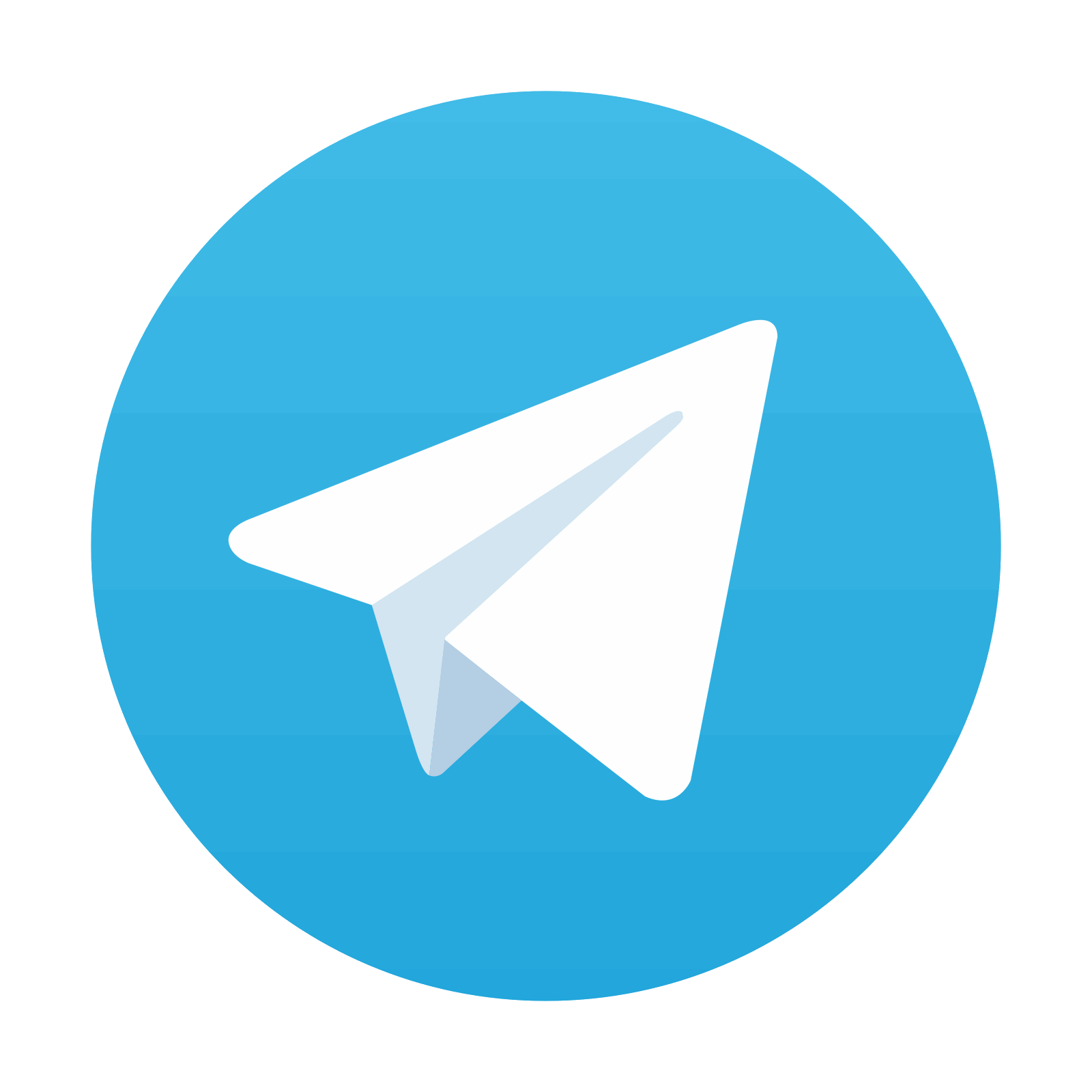
Stay updated, free articles. Join our Telegram channel

Full access? Get Clinical Tree
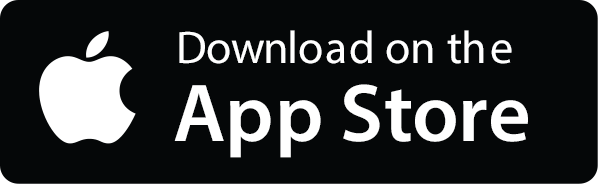
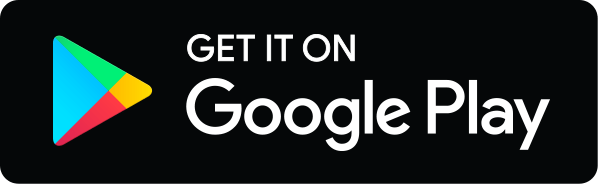