Impact of Vascular Disorders on Sleep
Jennifer Parr-Christmas
Monica M. Henderson
James D. Geyer
Paul R. Carney
LEARNING OBJECTIVES
On completion of this chapter, the reader should be able to:
1. Explain the autonomic physiology of sleep.
2. Explain the relationship between sleep and hypertension.
3. Describe the relationship between sleep and stroke.
4. Explain the relationship between sleep and heart failure.
5. Describe the effect of treatment with positive airway pressure on vascular disease.
KEY TERMS
Myocardial infarction
Heart failure
Metabolic syndrome
Sympathetic
Parasympathetic
Hypertension
Arrhythmia
CPAP
ECG
Catecholamines
VASCULAR DISORDERS AND SLEEP
Vascular diseases, including myocardial infarction, stroke, cerebrovascular disease, heart failure (HF), and cardiac arrhythmias, are the most common causes of morbidity and mortality in industrialized countries. Sleep-related breathing disorders are also widespread and increasing in prevalence because of the obesity epidemic, with approximately 20 million Americans (1) suffering from obstructive sleep apnea. Given the frequency of the two diseases, sleep disorders and vascular disorders, it is not surprising that they often coexist. The interactions between obstructive sleep apnea/hypopnea syndrome and vascular diseases are much more complex than originally thought, and their comorbidity is more than mere coexistence. Sleep-related breathing disorders, insomnia, and even normal autonomic changes associated with rapid eye movement (REM) sleep can adversely affect vascular diseases. This chapter will review the normal autonomic processes that are seen in sleep and discuss the role that sleep disorders play in heart disease, hypertension, and stroke.
NORMAL AUTONOMIC PHYSIOLOGY OF SLEEP
The autonomic nervous system regulates the involuntary, automatic functions of the visceral organs. It comprises the parasympathetic and sympathetic divisions, two opposing systems, the balance of which determines autonomic function. The sympathetic system has a stimulant effect and is responsible for the “fight or flight” response. Its primary neurochemical mediator is noradrenaline, an adrenaline-like substance that produces an increased heart rate, increased respiratory rate, vasoconstriction of blood vessels in visceral organs with vasodilatation of blood vessels in skeletal muscles, increased blood pressure, pupillary dilatation, plus inhibition of digestion, urination, and defecation. In contrast, the parasympathetic system counterbalances these effects. Its primary neurotransmitter is acetylcholine and its effects include slowing of the heart rate and respiration, vasodilatation of visceral organs with decreased blood pressure, pupillary constriction, increased peristalsis, plus emptying of the bladder and the rectum. Because vasoconstriction and hypertension are major contributors to myocardial infarction and stroke, it is useful to examine the body’s normal sympathetic and parasympathetic responses during sleep.
In general, at sleep onset, there is a reduction in sympathetic tone and a concomitant increase in parasympathetic tone, which causes a lowering of the heart rate, blood pressure, respiration rate, and tidal volume which continues during non-REM (NREM) sleep (2). With the transition to tonic REM sleep, the parasympathetic activity continues to increase and the sympathetic activity is further suppressed. During phasic REM
sleep, there are bursts of sympathetic activity (2). Heart rate variation during NREM sleep follows a sinusoidal pattern (2).
sleep, there are bursts of sympathetic activity (2). Heart rate variation during NREM sleep follows a sinusoidal pattern (2).
A normal respiratory sinus arrhythmia is produced by the normal respiratory activity coupled with cardiorespiratory center activity. During normal inspiration, the heart rate has a brief acceleration in order to accommodate venous return and increased cardiac output (2). During expiration, there is a progressive decrease in heart rate. This normal cardiac rhythm variability is a marker for cardiac health and its absence is associated with increasing age and possible cardiac disease. During REM sleep, it is normal for the heart rate to become increasingly variable, with episodes of moderate tachycardia and bradycardia. Respiratory patterns are also irregular and may result in mild oxygen desaturations. The neurons controlling the principal diaphragmatic respiratory muscles typically are not inhibited during REM sleep, but accessory muscles in the ribcage (the intercostal muscles) and neck may have partial muscle atonia. This causes the diaphragm to bear most of the load of respiration, which leads to partial diaphragmatic fatigue, hypoventilation, and decreased oxygenation over a period of several minutes in duration. These episodes are referred to as REM sleep hypoventilation episodes and should be scored as hypopneas if they produce an oxygen desaturation of 4% or greater over a period of time of 10 seconds or more (2).
There may be a transient 35% increase (3) in the baseline heart rate especially during phasic REM sleep because of a burst of sympathetic activation. Beta-blockers such as atenolol tend to reduce this phenomenon. Increased parasympathetic activity during NREM sleep contributes to cardiac electrical stability and helps decrease cardiac metabolic activity, reducing the risk of cardiac arrhythmia. The decreased blood pressure produced by the parasympathetic system during NREM sleep, however, can contribute to decreased blood flow to the coronary arteries and result in myocardial hypoperfusion, increasing the risk of infarction in patients with significant coronary atherosclerosis (2). The surges in autonomic activity and increased heart rate during REM sleep increase the risk for ventricular arrhythmias. Sympathetic activity not only results in increased oxygen consumption by the cardiac muscle but also produces coronary vasoconstriction, decreasing the blood flow to the heart, thus increasing the risk of cardiac ischemia. It has been documented that a significant number of myocardial infarctions occur in the early morning hours, upon awakening, or shortly after awakening; however, the relationship among sleep state, cardiac ischemia, and myocardial infarction is incompletely understood at this time.
Cerebral blood flow is closely linked to cardiac output, and the factors described earlier also impact blood flow to the brain. During REM sleep, there is an increase in blood flow to the limbic system and the brainstem, with circulation to these structures decreasing during NREM sleep. As brain activity increases during REM sleep, the cerebral requirements for glucose and oxygen both increase, and there is a compensatory increase in oxygenated hemoglobin delivery accompanying the transition from NREM sleep to REM sleep. Mild hypercapnia develops during NREM sleep and appears to counteract the circulatory effect of the decreased cerebral metabolic rate during NREM sleep. PaCO2 is an important determinant of respiration and hence cerebral blood flow during sleep in obstructive sleep apnea and other related disorders (2).
VASCULAR PATHOLOGY AND SLEEP
Hypertension
The Sleep Heart Health Study was designed as a prospective cohort study to investigate obstructive sleep apnea and other sleep-disordered breathing as a risk factor for the development of hypertension and cardiovascular disease (4). The results of the study suggest that the elevated sympathetic activity associated with sleep-related breathing disorders likely represents the primary mechanism in the pathogenesis of developing subsequent hypertension. This would typically begin as a loss of nocturnal dipping, the normal drop in blood pressure which occurs during sleep (5). Following the loss of nocturnal dipping, some patients develop elevated nocturnal arterial blood pressure, which can then progress to an elevated daytime blood pressure. Obstructive sleep apnea is an independent risk factor for the development of both nocturnal and daytime arterial hypertension (6). There appears to be a linear association between the severity of obstructive sleep apnea and the likelihood of developing subsequent hypertension. According to the Wisconsin Sleep Cohort Study, a patient with an apnea/hypopnea index greater than 15 events per hour has a 2.9-fold relative risk of developing hypertension compared with the normal patient population. This implies that many patients thought to have essential hypertension actually have hypertension secondary, at least in part, to obstructive sleep apnea.
The Joint National Committee on Prevention, Detection, Evaluation, and Treatment of High Blood Pressure has recommended that obstructive sleep apnea be excluded as a contributing cause of medically refractory hypertension. With the new tighter controls recommended for hypertension, screening for these contributing causes becomes increasingly important, especially in patients who have already had a heart attack or stroke.
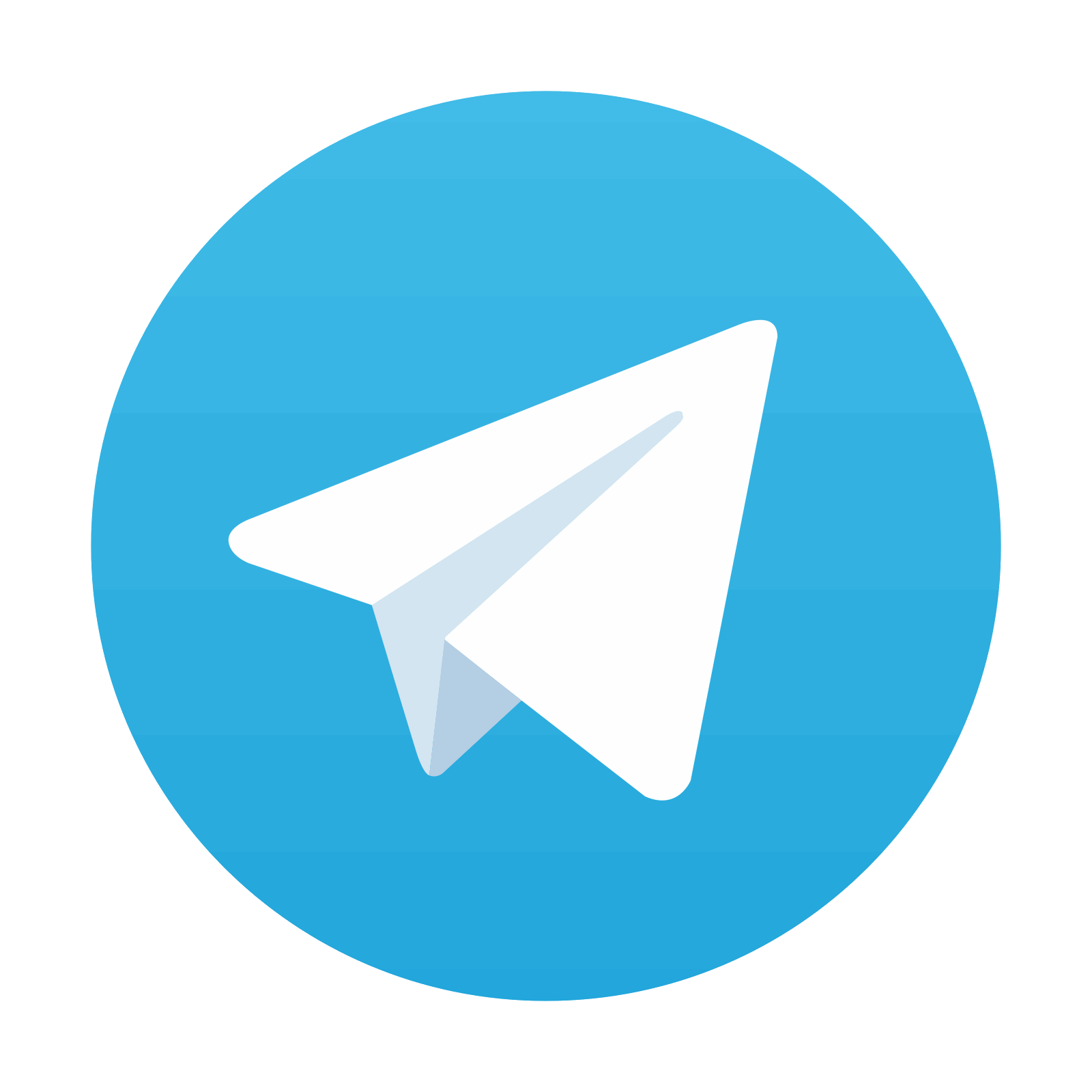
Stay updated, free articles. Join our Telegram channel

Full access? Get Clinical Tree
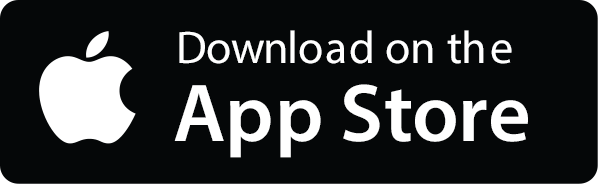
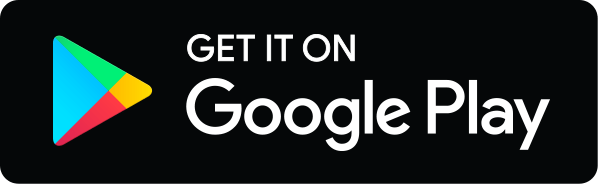