Implanted Devices and Central Nervous System Infection
The use of implanted devices is commonplace in most neurosurgical practices. Because of the tendency of implanted hardware to harbor microorganisms, the rate of postsurgical infections is generally higher, and the treatment of such infections often necessitates surgical removal of the involved hardware. In this chapter, we review the diagnosis, microbiology, and treatment of these challenging infections by category. We also review current strategies for prevention, which are discussed from other viewpoints in other chapters of this volume.
Shunt Infections
Hydrocephalus, estimated to affect nearly 1 in every 500 children,1 is one of the most common pediatric pathologies requiring neurosurgical intervention. This disorder may coexist with a variety of congenital or acquired brain disorders, most commonly myelomeningocele2 or intraventricular hemorrhage,3 followed by aqueductal stenosis, tumor, prior central nervous system (CNS) infection, and head injury. In contrast, common forms of hydrocephalus in adults include idiopathic normal-pressure hydrocephalus and obstructive hydrocephalus; it is also caused by cysts, tumors, hemorrhage, head injury, and meningitis.4 Although a variety of other treatments have been utilized, such as choroid plexectomy, choroid plexus cauterization, and carotid ligation, high morbidity and failure rates have forced these methods to be abandoned. The development of endoscopy has increased the use of third ventriculostomy, but a cerebrospinal fluid (CSF) shunt is the current mainstay of treatment for hydrocephalus.5
CSF shunts have three main components—a proximal ventricular catheter, a unidirectional valve, and a distal catheter—that function by diverting fluid from the ventricles to other body cavities, most commonly the peritoneum and rarely the right atrium or pleural space. The first shunts were used in the 1950s,6 and they currently account for nearly 70,000 hospital discharges and 36,000 surgical procedures in the United States, costing over $100 million annually.2,7 Although some studies suggest that the incidence of hydrocephalus and corresponding shunt insertion is decreasing,8,9 others have found that the prevalence has increased because of the improved survival of premature infants and older children with hydrocephalus.10 Despite nearly 60 years of experience with CSF shunts, their placement remains fraught with complications, and overall failure rates remain high, at nearly 40% in the first year.11,12 Malfunction due to mechanical obstruction or disconnection remains the most common complication, but infection is arguably the most dangerous, resulting in serious patient morbidity and mortality, long hospital stays, and costly interventions.5
Epidemiology and Risk Factors
The incidence of shunt infection varies greatly, with most modern studies reporting rates ranging from 2.1 to 12% per procedure4,9,13–27 and from 6.3 to 18% per patient.15,23,25 Following surgery, the median time to infection ranges from 10 to 72 days,4,14,21,26 with approximately 60% presenting within the first month17 and nearly 90% presenting in the first 6 months.28 Investigators have attempted to identify factors predicting the length of time to the development of infection, such as type of bacteria28 and whether the shunt is primary insertion or a revision14; however, most studies have not identified such a correlation. Although it is difficult to establish significant differences between infection rates in ventriculoperitoneal (VP), ventriculoatrial (VA), and ventriculopleural shunts because of small sample sizes of the latter two, it has been suggested that the infection incidence is lowest for VP shunts.9
There is a clear association between age and the incidence of infection, with the highest incidence noted in children. Tulipan and Cleves documented a rate of 15.7% in patients younger than 11.3 years of age, statistically higher than the rate of 6.7% in adults, with the highest incidence of 23% in infants less than 1 month old.16 These results have been corroborated in multiple studies,20,27 with a demonstrable inverse relationship between age and the incidence of infection.21 Most investigators note the highest infection rates in children younger than 1 year of age, many citing early gestational age, preterm birth, hydrocephalus etiology of intraventricular hemorrhage, or younger age at the time of shunt placement as independent risk factors for infection.15,24,25,28 Although the basis for this trend remains unclear, possible reasons include a relative immunodeficient response to bacterial infection in the first 6 months of life, higher bacterial density on the skin, longer hospital stays, and prior exposure to antibiotics. A multitude of patient, surgeon, and operative factors have been examined to identify risk factors for infection. In addition to age, patient factors found to increase the risk for infection include prior CSF leak, shunt revision, and infection.3,4,21,28,29 Demographic factors such as race, insurance type, and chronic medical conditions were found to be significant risk factors in one study15; however, these results were not widely reproduced. Studies have shown mixed results for the etiology of hydrocephalus as a risk factor for infection. Some suggest that etiologies such as intraventricular hemorrhage or prior shunt infection15,28 increase risk; however, others have not found any difference between etiologies.4,30 General risk factors for infection, such as prolonged hospitalization, prolonged steroid treatment, immunosuppressive states, and poor skin condition, may also play a role. Several surgeon and operative factors have also been reported to affect the incidence of infection, such as surgeon experience or case volume,9,19 time of day and length of surgery,4,31 use of a neuroendoscope,21 and the number of times the shunt is handled during surgery.24
Pathogenesis and Microbiology
The bacteria most often responsible for shunt infection are commensal, low-virulence, slowly replicating organisms. By far the most commonly observed pathogens (up to 71.8%)32 are coagulase-negative staphylococci, with Staphylococcus epidermidis the most often reported causative organism ( Table 16.1 ). Other gram-positive pathogens observed to cause infection include Staphylococcus aureus, particularly in patients with concomitant skin infections,4 and less frequently viridans-group streptococci, pneumococci, Enterococcus, Propionibacterium acnes, and Corynebacterium. Gram-negative bacteria (e.g., Escherichia coli, which accounts for more than 50%26 of cases, Klebsiella, Proteus, and Pseudomonas) account for a lower percentage of infections than gram-positive organisms and are usually associated with corresponding abdominal pathology. Although generally considered uncommon, polymicrobial infections account for up to 20% of infections and are often associated with an abdominal origin or contaminated head wounds.20 Fungal infections, most commonly involving Candida, are seen infrequently, usually in premature infants or immunocompromised patients.28
Given the frequency with which infection occurs soon after shunt surgery and the predominance of common skin flora as the causative organisms, it is logical to hypothesize that the majority of infections are the result of contamination at the time of surgery; indeed, Conen et al associated 72% of shunt infections with intraoperative sources.29 Although Bayston and Lari reported wound contamination in 58% of cases at incision closure,33 the origin of the such organisms was unclear. In a study comparing skin cultures with infection culture results, Shapiro et al noted that 22% of patients were infected with organisms identical to their skin flora, 22% had infections associated with other identifiable causes, and 55% were infected with typical skin flora that were different from the patients’ isolates.27 In addition to intraoperative contamination, other sources of infection include skin dehiscence over the shunt tract, hematogenous spread, and bowel perforation by the distal catheter tip, resulting in an ascending infection.29 Although VA shunts are more prone to infection through bacteremia, VP shunts are more susceptible to distal infection by intestinal pathogens.
Once contamination occurs, bacteria colonize the shunt lumen, which is an immune-protected space, and exposure to CSF aids bacterial adherence to the tubing.34 The prevalence of infection with coagulase-negative staphylococci is particularly important in the pathogenesis of shunt infection because these organisms are able to form an extracellular matrix made of glycoproteins, called a biofilm, that further facilitates adhesion and protects the bacteria from the immune system and antibiotics.28 Formation of the biofilm and peritoneal inflammation that walls off the distal end of the shunt catheter by forming a pseudocyst are two major contributors to the association between shunt infection and malfunction.35
Diagnosis
Clinical Presentation
The presentation of a shunt infection may be insidious and nonspecific, with variable clinical features that depend on the site of the infection. The most common manifestation of infection is fever with signs and symptoms indicative of shunt malfunction, such as headache, irritability, nausea and vomiting, and lethargy.28 Wounds with localized signs and symptoms, such as erythema, pain, swelling, CSF accumulation along the shunt track, and with progression purulent discharge, particularly in children with poor skin quality, may provide a visible source of infection, with at least one of these signs observed in up to half of cases.29 Less commonly, patients may present with neck stiffness or frank meningismus and, more rarely, seizures20 or glomerulonephritis.36 In the case of the VP shunt, abdominal symptoms mimicking common viral syndromes may confound the diagnosis, with signs of intra-abdominal infection being pain, vomiting, food intolerance, and peritonitis.
Diagnostic Course
In patients presenting with nonspecific signs and symptoms, particularly those with recent shunt surgery, a high level of suspicion for shunt infection should be maintained; however, it is always important, particularly with children, to complete a full history and physical examination to exclude other common infectious sources. Routine blood tests often show a peripheral leukocytosis22; positive blood culture in the setting of VP shunt infection is infrequent. Elevated C-reactive protein (CRP) levels may also be observed in nearly three-fourths of cases.37 Additionally, any apparent sites of infection, such as open wounds, should be cultured.
The most definitive way to diagnose a shunt infection is through the direct sampling of CSF from the shunt, known as a shunt tap, with the observation of organisms on either Gram stain or culture.39 Culture positivity is highest when sampling is done directly from the valve or reservoir (> 90%) rather than from a lumbar puncture, which yields a positive culture in fewer than half of cases.29 CSF cultures should be held for a minimum of 7 to 10 days to ensure growth of fastidious organisms.36,40 Although positive cultures provide a definitive diagnosis, studies have reported negative CSF cultures in infections confirmed by later culture of the shunt tip anywhere from 8%21 to 55.8%16 of the time, indicating that a negative culture does not rule out infection but may be due to several factors, such as prior antibiotic use. CSF findings supporting infection include leukocytosis with a predominance of neutrophils, eosinophilia,22 elevated protein, decreased glucose, and elevated lactate. Although all of these criteria may not be met, CSF analysis is completely normal in fewer than 3% of patients,29 and the combination of fever and ventricular neutrophils above 10% has a reported specificity of 99% and a positive predictive value of 93% for infection.22
Imaging in the diagnosis of shunt infection may include a shunt series to establish continuity of the shunt hardware. Computed tomography (CT) or magnetic resonance (MR) imaging should be used to examine the size of the ventricles, which may be enlarged with concurrent shunt malfunction. CT may show signs of infection in approximately 10% of cases, manifested mainly as meningeal enhancement or the presence of a brain abscess.29 For suspected cases of distal infection, older studies have suggested the use of ultrasound35; however, CT has since been found to be more reliable than ultrasound, demonstrating signs of infection such as inflammation of the fat or muscle around the shunt, thickened intestinal wall, abscess, shunt tip dislocation, or pseudocyst in three-fourths of episodes, in contrast to only half of episodes in which ultrasound was used.29 In summary, the most important method of diagnosis is a high level of clinical suspicion at the time of presentation, supported by CSF studies and imaging.
Management
Once the diagnosis of a shunt infection is made, the mainstay of treatment includes antibiotics with or without surgical management. Immediate clinical decisions include the choice of antibiotics, route of administration (systemic with or without intraventricular administration), and the fate of the shunt. Review of the literature reveals three general approaches to the management of an infected shunt38:
A two-stage surgical procedure with removal of the entire shunt and external drainage of the CSF, either by an external ventricular drain or by periodic tapping of the ventricles, with antibiotics administered systemically, intraventricularly, or both ways, followed by replacement of the shunt when the CSF is sterile
A one-stage procedure with removal of the entire shunt and immediate replacement, with antibiotics administered systemically, intraventricularly, or both ways
Antibiotic therapy alone, administered systemically, intraventricularly, or both ways
Additional surgical options in combination with antibiotics may include distal externalization of the peritoneal catheter in cases with a mild suspicion for infection or isolated abdominal infection and malfunction,35,36 complete removal of the shunt without replacement, and complete removal with the later performance of a third ventriculostomy.23 The majority of patients will continue to require management of their intracranial pressure through CSF drainage, making the latter two options viable in only a small subset of patients.
Antibiotics
The choice of antibiotics is highly dependent on the presumed etiology of the infection, patterns of susceptibility, and penetration of the blood–brain barrier (BBB). Typical empiric, broad-spectrum antibiotics initiated before culture and sensitivity results are obtained may include vancomycin, a third-generation cephalosporin, and a third agent to cover anaerobes.36 First-generation cephalosporins should be avoided because of their poor BBB penetration.39 Because of high rates of resistance to methicillin (> 60%38), oxacillin, and penicillin (33%20), vancomycin is the drug of choice against staphylococci. Oral or intravenous rifampin may be added in combination with vancomycin because it is able to penetrate the biofilm and attain bactericidal levels at the shunt surface38; however, it should not be used alone because of the development of resistance. CSF Gram stain may be of early assistance in choosing an antibiotic regimen, and CSF culture and sensitivity results will allow antibiotic coverage to be tailored to specific pathogens.
All patients should receive systemic antibiotics. Whether or not to add intraventricular antibiotics to the regimen is a point of contention. The logical rationale behind the use of intraventricular antibiotics is to overcome the poor BBB penetration achieved with intravenous administration, especially in shunt infections caused by low-virulence bacteria associated with minimal meningeal inflammation. Intraventricular administration allows maximal drug concentration at the site of infection, with higher peak concentrations and better maintenance of therapeutic levels, as opposed to the observed ratios of CSF concentrations to blood concentrations after systemic antibiotic administration, which can range from 0 to 30%.41 Reported complications with intraventricular antibiotic administration are infrequent, with the most serious being focal motor seizure.42
The appropriate duration of therapy is not well defined in the literature and is predominately based on the observation of clinical improvement combined with sterilization of the CSF. The reported mean duration of antibiotics ranges from 9 to 18 days.14,29,38,39 Arnell et al reported that clinical improvement paralleled CSF sterilization and suggested performing repeat CSF culture 2 to 3 days after diagnosis, then if the cultures are negative, completing a total of 5 to 10 days of antibiotics, and if they are positive, continuing daily CSF cultures.40 Anderson and Yogev reported cost and health benefits for shorter periods of antibiotics and recommended as few as 3 days of therapy for the mildest infections and 10 days for persistent cases.39 The definition of infection “cure” varies; it may be limited to clinical improvement and CSF sterilization or include an extended period of infection-free follow-up. After cure of the immediate infection, if it has been surgically managed with shunt removal and later replacement, antibiotics are typically discontinued on the day of shunt replacement.
Medical Management
Treatment with antibiotics alone has the benefit of avoiding surgery and its associated morbidity, especially in patients with multiple retained catheters. Simon et al managed 13.3% of patients with antibiotics alone with a statistically significant shorter length of hospital stay, but they also identified a trend toward higher reinfection rates.14 In contrast to most reported cure rates with antibiotics alone,40 Brown et al achieved a cure rate of 84% over all and in 93% of patients with S. epidermidis infection.38 Importantly, this study excluded patients with S. aureus infection because of a high early failure rate, as well as patients with shunt malfunction, redundant shunts, brain abscess or subdural empyema, external infection, fungal infection, or local abdominal infection, thus making the study population quite specific. Additionally, all patients received intraventricular antibiotics through a ventricular catheter, which was placed if the patient did not have one before presentation. Although likely not the best choice for a majority of patients, this study suggests that antibiotic therapy alone may be efficacious for a carefully selected group of patients.
Surgical Management
Despite the potential complications associated with undergoing a surgical procedure in view of the adherence of bacteria to shunt material, removal of the hardware is the definitive method of eliminating persistent contamination that could cause recurrent infection.36 Two primary surgical approaches dominate the treatment of shunt infection: a two-stage and a one-stage procedure. Most institutions report surgical treatment as the standard of care,3,14,29,39,40 with a majority employing the two-stage method. In the case of a two-stage treatment with placement of an external ventricular drain, the average length of external ventricular drain placement is approximately 1 week; however, reinternalization should not be unnecessarily delayed because reinfection rates correlate with the duration of external ventricular drain placement.39 Kestle3 reported a mean time to replacement of 13 days after CSF sterilization; however, in a study of more “aggressive” treatment, James and Bradley reported similar success rates with shunt replacement after antibiotic treatment for less than 12 days and after CSF cultures were negative for 24 compared with 48 hours.42
The choice of optimal treatment of shunt infection is hampered by the lack of prospective, randomized clinical trials. An older published trial compared antibiotics alone with one- and two-stage surgical approaches, all patients receiving both systemic and intraventricular antibiotics.43 This study reported a cure rate of 100% with a two-stage approach, 90% with a one-stage approach, and 30% with antibiotics alone. The antibiotic group also exhibited a higher mortality rate (20% versus 0% in other groups) and longer hospital stays (45, 33, and 24 days for antibiotics, one-stage procedures, and two-stage procedures, respectively). Although this trial was limited by the small sample size, modern reviews of published studies have corroborated the results. In 1985, Yogev reviewed 18 studies and documented cure rates of 96%, 65%, and 36% for two-stage procedures, one-stage procedures, and antibiotics alone, respectively.44 Similarly, in 2002, Schreffler et al performed a decision analysis and reviewed 17 studies with corroborating cure rates of 87.7%, 64.4%, and 33.5% for each approach.45
With respect to morbidity and mortality, historical studies have shown that morbidity, measured as average number of subsequent hospital admissions and average number of days in the hospital for each infection, is lowest for surgical treatment, as is mortality.23 In a more recent study of treatment approaches, a trend toward lower mortality was documented for a two-stage approach,37 and reviews have documented 5.7%, 11.1%, and 20.4% mortality rates for two-stage procedures, one-stage procedures, and antibiotic treatments, respectively.45 The decision analysis for this same study assigned utility values to each approach based on cure, morbidity, and mortality and concluded that the two-stage approach has the highest utility. Although the two-stage approach is not without complications, it has been established as the current standard of care by resulting in the highest cure rate with lowest morbidity and mortality rates.
Outcomes
Long-term outcomes after shunt infection have serious implications for the health and well-being of patients. After an initial infection, the risk for reinfection is higher, with 16 to 25% of patients admitted with reinfection within 12 months.3,14 Although infections are usually insidious, nearly 20% of patients are admitted to neurosurgical intensive care units.29 Infection can lead to the development of multiloculate hydrocephalus, with an increased need for repeat surgery and lifelong shunt dependence,36 an increased risk for seizures and decreased intellectual performance and cognitive function, and a twofold higher increase in long-term mortality rate.23,46,47
Prevention
Although preoperative antibiotics are widely employed,18 many studies suggest that the common use of cephalosporins, such as cefazolin, may not decrease the risk for infection,4,34 especially as first- and second-generation antibiotics do not have good BBB penetration. Intravenous vancomycin has been shown to be more effective (14% versus 4% infection rate) than cephalosporins, with lower mortality rates and shorter lengths of stay18 in hospitals that have a high prevalence of methicillin-resistant S. aureus (MRSA). The intraoperative use of intraventricular antibiotics, specifically vancomycin and gentamicin, resulted in one study in a decrease in the infection rate from 6.5% to 0.412% with no adverse effects.17 Meticulous operative technique and control of the operative theater are thought to be the best prevention,1,48 with the inclusion of double gloving into standard surgical practice.16 The introduction of antibiotic-impregnated ventricular catheters has provided some evidence for the prevention of infection13; however, results have been mixed.49,50
Spinal Instrumentation–Associated Infections
The multiple indications for spinal fusions include treatment of scoliosis, stenosis, spondylosis, spondylolisthesis, trauma, tumor, and instability. Because of the advancing age of the baby boomer generation, the percentage of the total population comprising older adults in the United States will continue to increase, and it is estimated that there will be 87 million Americans past the age of 65 by the year 2050, representing more than 20% of the total population.51 In turn, the growing number of older Americans with spinal pathology requiring operative intervention will lead to an increase in the number of fusion procedures performed. This trend in spinal surgery has already begun, and based on recent estimates, over 460,000 spinal fusions were performed in 2010, with a total cost of upward of $43 billion. In comparison, in 1997, roughly 130,000 spinal fusions were performed in the United States, with an estimated aggregate charge of slightly over $3 billion.52
Definitions
The Centers for Disease Control and Prevention (CDC) classify surgical wound infections as either incisional site infections or organ/space infections, with further stratification of incisional surgical site infections (SSIs) based on the tissue compartment involved ( Table 16.2 ).53 A superficial incisional SSI involves the skin and/or subcutaneous tissues, whereas a deep incisional SSI involves the fascial and subfascial layers. In the lumbar spine ( Fig. 16.1 ), deep infections occur subjacent to the lumbodorsal fascia with a posterior lumbar wound and below the anterior abdominal fascia with an anterior approach. The ligamentum nuchae and fascial layer serve as the anatomic landmarks to delineate the deep layers of the posterior cervical tissue, whereas deep wound infections after anterior cervical surgery are located deep to the platysma.54 Of note, postoperative infections may also involve the intervertebral disks, the vertebral bodies, and/or the epidural space, resulting in diskitis, osteomyelitis, or epidural phlegmon/abscess.
Epidemiology
The development of an infection is a known complication following surgery and is a common cause of patient morbidity in the postoperative period. Wound infections, particularly deep wound infections of the spine, have significant consequences for patients and for the health care system at large. Postoperative spinal wound infections generally lead to longer in-hospital lengths of stay, higher costs, and the potential for reoperation. Moreover, these complications are known to increase patient morbidity and mortality.55–59 Achieving a decrement in the rate of postoperative wound infections has been the premise behind many centers’ adopting various guidelines for perioperative patient care; however, the prevention of wound infections involves consideration of pre-procedural, intra-procedural, and post-procedural sources of microbial colonization and thus requires a multifaceted approach to patient management.

Patient-specific risk factors, such as advanced age, a history of drug and alcohol abuse, smoking, diabetes, obesity, malnutrition, and immunologic compromise,60 reduce the patient′s ability to mount an adequate inflammatory response against pathogens.61 Smoking and diabetes, specifically, are believed to lead to microvascular damage and tissue ischemia, which in turn increase the risk for SSI development.62–66 In terms of pre-procedural care, advocating for measures such as smoking cessation and improved preoperative diabetic control and nutritional status should be employed to optimize patient risk factors. In addition, surgeries performed for patients with traumatic spinal injuries or neoplastic processes are associated with an increased incidence of SSIs.61
As surgeries become more complex, with an increasingly wide variety of pathologies treated and instrumentation used, the likelihood of infectious complications increases.67,68 Studies have estimated that the incidence of deep wound infections following instrumented lumbar fusion surgery ranges between 1 and 14%.55–58,67,69–79 Repeat surgery, a greater number of levels fused, a posterior approach, longer operative times, blood transfusions, and increased intraoperative blood loss are all associated with postoperative SSI.60,68,80–82 Another risk factor that has been identified is the presence of more people in the operating theater, a finding especially germane to spinal instrumentation surgeries, in which the surgeon, assistants, anesthesia team, circulators, and equipment representatives all share the operating room, increasing traffic and adding potential sources of infection.83
Minimally invasive approaches have been shown to compare favorably with traditional, open surgical approaches with respect to the incidence of wound infections.84,85 Fewer postoperative deep wound infections are seen after instrumented cervical fusion surgery; however, as recent reports demonstrate, patients who undergo operative fixation and arthrodesis via an isolated posterior approach have a higher rate of wound infection than those treated anteriorly.86,87 In patients who manifest with deep wound infections after undergoing anterior cervical diskectomy and fusion, the clinician must have a high index of suspicion for esophageal injury, which carries significant morbidity and mortality.88
Understanding surgery-specific risk factors, adhering meticulously to aseptic technique, and employing measures to decrease the potential for microbial colonization are crucial to minimizing the potential for developing subsequent deep wound infections, as highlighted by recommendations published by the CDC for SSI prevention.53
As previously noted, measures to reduce the risk for developing a postoperative wound infection are not relegated to the preoperative and immediate perioperative periods. As such, the postoperative management of patients is also important in preventing surgical site complications. Open and effective communication between the patient, nursing staff, and physicians is integral to optimizing patient outcomes. Protecting the surgical wound with a sterile dressing for 24 to 48 hours postoperatively, washing hands before and after dressing changes and when any contact with the surgical site is anticipated, and using sterile technique are recommended.53 Administration of antibiotics is discouraged beyond 24 hours as there is scant evidence to support this practice. Additionally, prolonged administration of antibiotics has been shown to result in the selection of antibiotic-resistant bacterial species.89 Although closed suction drainage has traditionally been employed because of its potential to promote wound healing and also prevent bacterial colonization and growth within the surgical bed, there is little evidence to support its use.90 The results of recently published works illustrate that drains may not lead to any reduction in wound infections,90,91 and with prolonged use, they are an independent risk factor for subsequent development of an SSI.92
Microbiology
Most commonly, S. aureus is responsible for SSI development. Recent reviews have shown that the incidence of MRSA isolates in patients manifesting with SSI is upward of 34%.83 S. epidermidis, Enterococcus faecalis, Pseudomonas species, Enterobacter cloacae, and Proteus mirabilis are other, less commonly isolated bacterial pathogens. Patients with traumatic injuries to the spine and those with urosepsis are particularly vulnerable to infection by gram-negative organisms.61
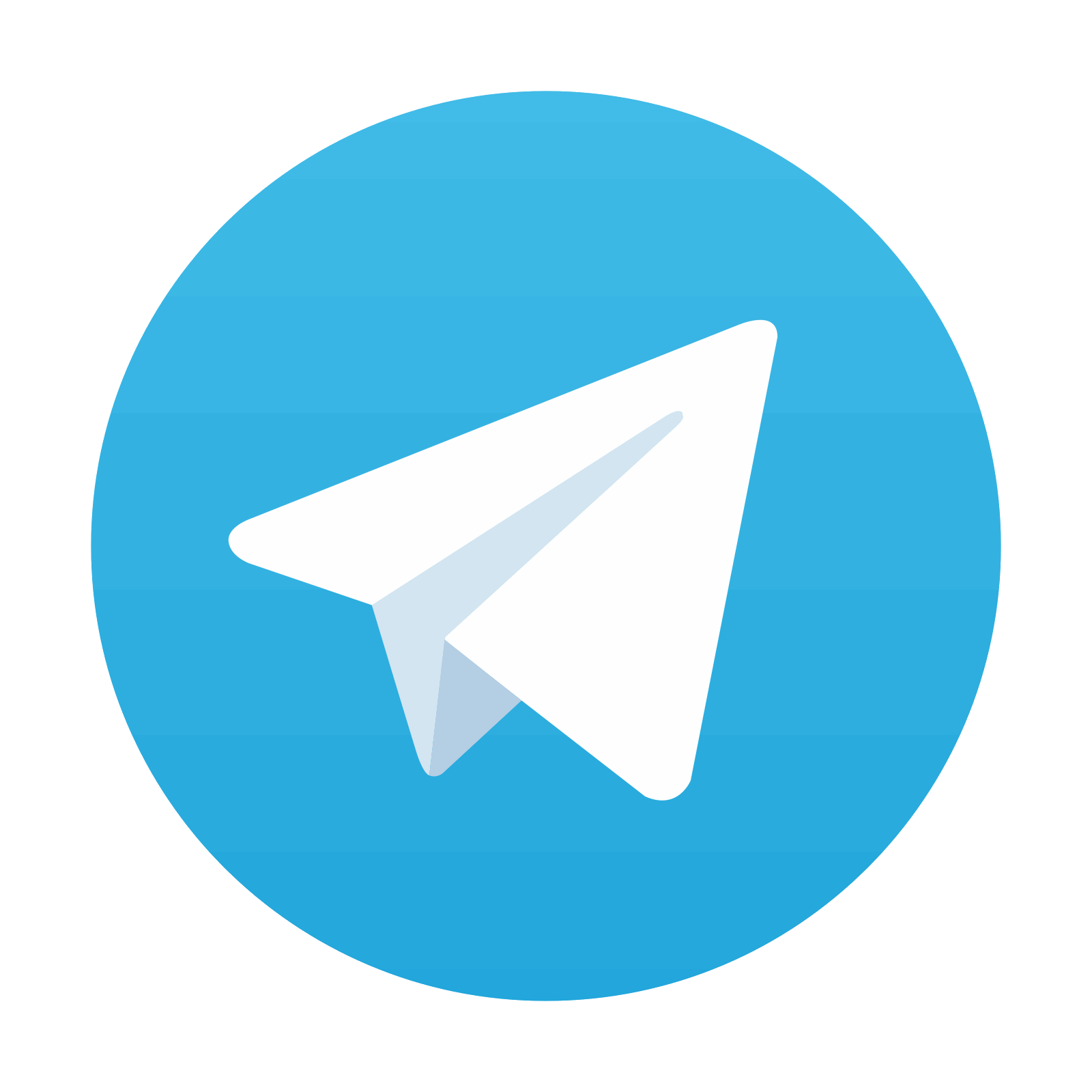
Stay updated, free articles. Join our Telegram channel

Full access? Get Clinical Tree
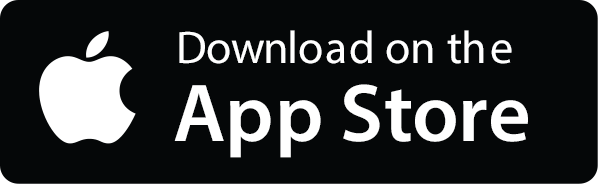
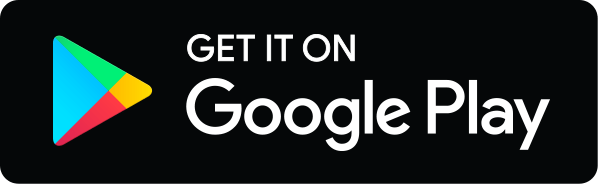
