Fig. 5.1
Flowchart of pre-analytical steps in CSF analysis and common variability factors influencing outcome measures
To generate recommendations for pre-analytical procedures, a distinction can be made between analysis of a specific biomarker in the clinical diagnostic setting and biobanking for research purposes, where samples are usually stored to identify novel biomarkers with unknown pre-analytical specifics. To illustrate this, adding Tween-20 to a CSF sample prevents amyloid beta (Abeta)(1-42) peptides from absorbing to several lab plastic surfaces (Pica-Mendez et al. 2010). Adding Tween-20 will decrease pre-analytical variation in these samples when they are collected for amyloid determination only. On the other hand, they will be unserviceable to many other purposes, e.g., studies targeting potential new biomarkers, because Tween-20 might interact with CSF components or the assays. In biobanking practice, we therefore advise to not add any substance to CSF samples in order to avoid possible adverse effects.
Another major difference between routine analysis and biobanking for research purposes is the storage duration, which can be as short as one hour for routine tests which are often automated, including cell counts, protein concentration, and IgG index, up to 1 month for special tests as oligoclonal bands, IgG index in some settings, and Alzheimer biomarker analysis by ELISA. The value of a biobank in the research setting, in contrast, increases upon long-term follow-up of patients and when cohort sizes are allowed to increase. Thus, storage of material will be rather multiple years than months. Therefore, protocols for long-term biobanking purposes are more stringent and require storage at −80 °C.
One approach to reduce variation due to patient issues and laboratory processing is to standardize CSF collection protocols as much as possible, which has been addressed by several consortia in recent years. The current protocols are in the majority based on the initial consensus guideline of the BioMS consortium (Teunissen et al. 2009) that felt that the first step to improve the quality of biomarker studies and minimize variation between studies was to standardize the collection procedures. The most updated version of the protocol is presented in Table 5.1, which includes a standardized protocol for blood collection as well. The idea behind the protocols is to standardize every aspect during the CSF process to minimize variation, even if the rationale for specific decisions cannot as yet be given due to lack of experimental data.
Table 5.1
Collection protocol for CSF and blood pairs for biobanking
Item no | Procedure | Ideal situation for CSF | Blood |
---|---|---|---|
A. Collection procedures | |||
1 | Time of day of withdrawal and storage | Record date and time of collection | Same as for CSF |
2 | Preferred volume | At least 12 ml. First 1–2 ml for routine CSF assessment. Last 10 ml for biobanking | 10 ml EDTA plasma, 10 ml serum |
Record volume taken and fraction used for biobanking, if applicable | |||
3 | Location | Intervertebral space L3–L5(S1) | Venipuncture |
4 | If blood contamination occurred | Do not process further | Na |
Criteria for blood contamination: more than 500 red blood cells/μl | |||
Record number of blood cells in diagnostic samples | |||
5 | Type of needle | Atraumatic | Standard needles, e.g., 21–23 G |
6 | Type of collection tube | Polypropylene tubes, screw cap, volume >10 ml | For serum: no clotting activator or gel |
For EDTA plasma: no protease inhibitors | |||
7 | Other body fluids that should be collected simultaneously | Serum | Na |
8 | Other body fluids that should be collected simultaneously | Plasma: EDTA (preferred over citrate) | Na |
B. Processing for storage | |||
9 | Storage temperature until freezing | Room temperature before, during, and after centrifugation | Same as CSF |
10 | Centrifugation conditions | 2,000 g (1,800–2,200), 10 min at room temperature. 400 g if cells are to be preserved | 2,000 g (1,800–2,200), 10 min at room temperature |
11 | Time delay between withdrawal, processing, and freezing | Between 30 and 60 min. Max 2 h | Between 30 and 60 min. Max 2 h |
After centrifugation, samples should be aliquoted and frozen immediately, with a maximal delay of 2 h | Less than 1 h is optimal for proteomic discovery studies. Serum must clot minimal 30 min | ||
12 | Type of tube for aliquoting | Small polypropylene tubes (2 ml for routine diagnostics; 0.5 ml for biobanking) with screw caps. Record manufacturer | As CSF |
13 | Aliquoting | A minimum of two aliquots is recommended. The advised research sample volume of 10 ml should be enough for >10 aliquots | As CSF |
14 | Volume of aliquots | Minimum 0.1 ml. Depending on total volume of tube: 0.2, 0.5, and 1 ml. Preferably, the tubes are filled up to 75 % of the volume | As CSF |
15 | Coding | Unique codes. Freezing-proof labels. Ideally barcodes to facilitate searching, to aid in blinding the analysis, and to protect the privacy of patients | As CSF |
16 | Freezing temperature | −80 °C | As CSF |
We used the framework of Fig. 5.1 to discuss effects of pre-analytical variation on CSF biomarker concentrations in the next paragraphs.
5.2 Patient-Related Factors
Patient misidentification or incorrect requesting are major sources of variation in laboratory settings and are not specific for CSF. These can be improved, e.g., by the introduction of electronic patient records and linkage of these systems to research databases. For example, one study reported a striking reduction of 80 % in errors, where major factors in which a reduction in errors was attained were the correct information on the sample tube (patient name, unique patient ID number, date of collection), the correct sample received, and the availability of a clinical history in primary care setting (Turner et al. 2013). The future of sample labeling in biobanking for research presumably lies in 2D barcoding, which will serve automated sample retrieving and picking, will further reduce mistakes, and facilitates research using pseudonymized samples.
5.2.1 Item 1 of Table 5.1: Time of the Day of Withdrawal and Storage
Patient-related pre-analytical factors also can influence biomarkers outcomes (Fig. 5.1). Such factors include effects of fasting, smoking, alcohol use, caffeine intake, and exercise. It is conceivable that dietary factors would primarily affect specific blood markers rather than CSF biomarkers. However, fluctuations in fatty acid amide concentrations were measured in CSF of rats between day and night, due to differences in food intake (Murillo-Rodriguez et al. 2006). Experimental evidence in humans is lacking, and where compounds, such as alcohol or smoking, have effects on neurons (Downer and Campbell 2010; Oliveira-da-Silva et al. 2009), they may also have an effect on CSF. Thus, there are limited descriptions of CSF biomarkers being affected by these lifestyle factors. However, if there are effects of smoking or drinking on CSF biomarker concentrations, it will be hard to define if these are trait markers or should be seen as direct pre-analytical confounders. For all these factors, documentation is important for long-term biobanking and future research.
Studying the effect of circadian rhythm (and fasting) of specific proteins in CSF is not easy to perform, as repeated sampling within a person needs to be performed. However, some recent reports showed fluctuations in CSF protein levels due to diurnal rhythm. For example, Kang and colleagues detected a diurnal rhythm of Abeta(1-42) levels in a group of ten healthy individuals, with a variation of 27.6 % between morning and evening (Kang et al. 2009). A variation of 5.5–12.2 % was found in levels of Abeta(1-42), Abeta(40), Tau, and pTau, measured once per 6 h, in patients who were on CSF drainage as part of their clinical routine since they were suspected of idiopathic normal pressure hydrocephalus (n = 9) or pseudotumor cerebri (n = 1). This circadian variation was only slightly higher than the interassay coefficient of variation, and there was too much variation in rhythm between individuals to indicate a circadian fluctuation at group level (Moghekar et al. 2012). Another study from the Bateman Group reports diurnal fluctuation of amyloid CSF biomarkers (sAPPalpha, sAPPbeta, Abeta(42), and Abeta(40)) in three cohorts: a young cohort and two older cohorts, one with and one without amyloid pathology present. Amplitudes of the biomarkers were fluctuating in the range of 2–20 % over 36 h, dependent on group (diminishing with age) and specific per biomarker (Dobrowolska et al. 2014). Thus, the effects of circadian fluctuations were small since they marginally exceed the variation of the assay. The peak hours of biomarker fluctuation could not be patterned (Dobrowolska et al. 2014), indicating that standardizing time of withdrawal will not eliminate pre-analytical variation on an individual basis.
Taken together, the only option to document this variation seems to be to drain CSF during 24 h and monitor circadian protein fluctuation per individual. This is however too time-consuming, not cost-efficient, and uncomfortable for patients to realize in practice. Given the fact that most lumbar punctures are performed during clinical work-up, it will be hard to standardize time of withdrawal. Standardizing time of withdrawal is thus not recommended, also since biomarker fluctuations differ per person and accounted so far only for minor variation in outcome, which may also be even less in the short time window of office hours. Since effects cannot be excluded for every biomarker, recording time of withdrawal is important for biomarker development.
5.3 Laboratory Processing
In this paragraph, we discuss the guidelines for CSF processing and biobanking as presented in Table 5.1, supported by experimental research when available.
5.3.1 Item 2 of Table 5.1: Volume of Withdrawal of at Least 12 ml
The CSF volume taken can influence the concentration of biomarkers. If a small volume is taken, the CSF will reflect the composition of the lumbar dural sack, whereas large volumes may reflect the rostral spinal or even ventricular CSF. Most molecules and cell numbers are not equally distributed throughout the spinal fluid, and it is known that an increasing gradient in protein concentration from ventricular to lumbar CSF exists (Reiber 2003; Tarnaris et al. 2011). Therefore, if biomarker levels in a sample from a puncture of 2 ml are compared to that in a puncture of 15 ml, this can lead to erroneous comparisons for specific proteins.
In a recent study, CSF protein concentrations of the neuron-specific protein S100B and the neuron-specific enolase did not differ between ventricular and lumbar CSF in the same patients, though the leptomeningeal beta-trace and the blood-derived albumin did (Brandner et al. 2013). A proteomic study comparing the first and the tenth ml from one CSF withdrawal revealed only one protein, apolipoprotein C1, to be significantly increased among 41 identified masses of which 11 proteins were identified (Simonsen et al. 2010). Probably, the production location of a protein is crucial for the presence of a ventriculo-lumbar gradient in CSF (Tumani and Brettschneider 2005); however, this does not solve the uncertainty for CSF gradients being present for individual proteins, especially not if they are relatively unknown proteins. Therefore, we generally recommend to puncture a standard volume of CSF, especially for biobanking, but at least record the volume. The first 2 ml can be used for basic CSF analysis, and the remainder of the sample should be pooled before aliquoting. If this is not possible, the fraction of each portion must be recorded. Of course, a larger volume of spinal fluid will facilitate the number of possible analyses. It is well established that the volume of collected CSF does not correlate with the risk of post-lumbar puncture headache (Grant et al. 1991; Kuntz et al. 1992) (Chapter 4).
5.3.2 Item 3 of Table 5.1: Location of Puncture, L3–L5
Usually, diagnostic CSF is obtained by lumbar puncture, and the rationale for the location in relation to patient safety and to avoid discomfort is described in chapter 5. In practice, CSF will be taken only rarely from other locations than L3–L5, such as the cervical cisterns or from the lateral ventricles (e.g., ventricular drainage). Location of the puncture should thus be recorded since it matters for a few peptides (Tarnaris et al. 2011; Brandner et al. 2013) and it might affect concentrations of not yet detected CSF proteins.
5.3.3 Item 4 of Table 5.1: Removal of Bloody CSF Samples
A traumatic tap causing blood contamination of CSF occurs in about 14–20 % of standard lumbar punctures (Petzold et al. 2006). For markers that have high blood concentrations, such as coagulation factors, blood contamination can lead to false-positive results, while no effects have been reported for proteins from neuronal origin as neurofilament light and heavy chains (Koel-Simmelink et al. 2014). Vascular endothelial growth factor (VEGF) and neuron-specific enolase (NSE) are predominantly present in blood platelets and are CSF biomarker candidates for some neurological and neurodegenerative diseases. NSE levels linearly rise with increased hemolysis of both serum and CSF (Ramont et al. 2005). Also, the presence of cellular components in the CSF influences the levels of these proteins, the effect of which will be minimized by centrifugation. For example, VEGF levels remained relatively stable after spinning (Yang et al. 2011). In addition, the presence of blood proteins leads to suppressed MALDI-TOF proteomic patterns in CSF. This suppression by blood proteins is, however, highly reduced after removal of the blood cells by centrifugation of the sample prior to initial freezing and subsequent analysis (Berven et al. 2007; Jimenez et al. 2007). Recording of erythrocyte count is essential to select CSF samples appropriate for these measurements. Based on the proteomic studies, CSF samples with an erythrocyte count up to 500/μl can be included for biomarker studies, though lower percentage contamination is preferred to avoid effects on as yet unknown molecules. It is highly recommended though to spin samples to avoid blood contamination. The use of alternative markers as quality indicators to indicate hemolysis of CSF (e.g., hemoglobin alpha and beta chains) is indicated (Jimenez et al. 2007), but thresholds remain to be defined.
5.3.4 Item 5 of Table 5.1: Use of Atraumatic Needles
There is no evidence that the type of needle for lumbar puncture influences biomarker concentrations. However, atraumatic or small-gauge needles are best tolerated by patients and are associated with a lower risk for post-lumbar puncture headache (chapter 5). An advantage of the traumatic needles is an increased CSF flow. Selecting the optimal type of needle for CSF sampling is elaborated in detail in chapter 5.
5.3.5 Item 6 of Table 5.1: Use of Polypropylene Collection Tubes
Proteins can stick to lab plastics, a characteristic that is exploited during, e.g., ELISA assays. This characteristic becomes disadvantageous though when the type of collection tube influences biomarker outcomes. Moreover, many collection tubes release polymeric components (Bowen et al. 2005) that can in some cases affect immunoassays. The Abeta(1-42) peptide in particular proves sensitive to different tubes used (Lewczuk et al. 2006; Lehmann et al. 2014; Perret-Liaudet et al. 2012; Toombs et al. 2014). The use of polypropylene tubes, with their low protein-binding potential, was proposed for collecting CSF. Actually, experimental evidence did not confirm the advantage of tubes purely composed of polypropylene (Perret-Liaudet et al. 2012), and comparing multiple polypropylene tubes did not give congruous results (Lehmann et al. 2014). Thus, harmonization of the tubes proves a worthwhile effort to reduce variation and introducing one tube type with the lowest binding capacity for universal use still lies ahead. The European JPND “BIOMARKAPD” consortium was initiated to standardize and harmonize the use of biomarkers for AD and Parkinson’s disease, and it is addressing many of the before-mentioned topics. This consortium decided to use so far a Sarstedt polypropylene tube (cat nr: 62.610.018, 10 ml collection tube, round base; cat no. 62.554.502, 15 ml tube with conical base to use if a pellet is to be kept). An additional component causing variation is transferring CSF to new tubes: the more transfers, the more protein concentrations are reduced, especially for sticky peptides such as Abeta(1-42) (Toombs et al. 2014). The focus currently is on detailed examination of tube types, tube transfers, and additional elements such as tube caps in order to further optimize the recommendation of the use of specific tubes for CSF collection.
5.3.6 Items 7 and 8 of Table 5.1: Withdrawal of Serum and Plasma Linked to the CSF Sample
It is important to collect matched serum and/or plasma samples for evaluation of CSF biomarkers because the concentration of the marker in blood often influences that in CSF. Blood collection is moreover needed to calculate the IgG index for diagnostic purposes and to define the intrathecal origin of a biomarker and thus its specificity for the CNS (Deisenhammer et al. 2006). For novel biomarkers of neuronal origin, it is relevant to determine the presence of a candidate CNS marker in serum/plasma. Blood tests are ideal for monitoring biomarker concentrations over time for monitoring of disease progression or even population screening.
For work-up, vacutainer tubes that use EDTA (in dried format) are preferred over those that use citrate (in solution) because if tubes containing a standard volume of citrate solution are filled incompletely, the final biomarker concentration is diluted artificially. Serum/plasma samples should not be hemolyzed or lipemic, and a standardized blood collection protocol is also provided in Table 5.1.
5.3.7 Item 9 of Table 5.1: Storage at Room Temperature Until Spinning and Aliquoting
For CSF, there are no data available yet that support a preference for leaving the samples at either room temperature or 4 °C until processing. A direct comparison was made between neurofilament protein levels stored at room temperature or 4 °C, and no difference in protein levels was found (Koel-Simmelink et al. 2014). CSF enzymes, such as adenosine deaminase and acid sphingomyelinase, do not have a decreased enzymatic activity when stored for a maximum of 24 h at room temperature (Lu and Grenache 2012; Mühle et al. 2013). Therefore, processing at room temperature for both serum/plasma and CSF, including during and after spinning, is suitable for most studies. However, if RNA is to be sampled from CSF immune cells, the CSF should be spun immediately or stored at 4 °C until processing.
5.3.8 Item 10 of Table 5.1: Standardized Spinning Conditions
We propose to adhere to a standardized spinning protocol of 400 g for 10 min at room temperature for CSF if cells are to be collected, otherwise between 1,800 and 2,200 g (10 min at room temperature). For serum, we recommend to spin at 2,000 g for 10 min at room temperature. Not many experimental reports on this topic exist. A preliminary study showed lower concentrations of CSF protein DJ-1 with spinning at 2,000 g compared to spinning at 1,300–1,800 g; however, the effect was small and possibly due to other pre-analytical factors which differed between the experimental groups (Salvesen et al. 2014). For plasma and serum, temperature of processing is known to be critical for specific biomarkers, such as TIMP-1, probably due to degranulation of platelets at room temperature (Lomholt et al. 2007).
5.3.9 Item 11 of Table 5.1: Standardization of Time Delay Between Withdrawal, Spinning, and Freezing
Proteomic studies proved stability of CSF proteome for up to 2 h after withdrawal prior to further processing (Berven et al. 2007; Jimenez et al. 2007). Similar stability was shown for neurofilament heavy- and light-chain proteins for up to 24-h time delay between lumbar puncture and spinning, independent of the temperature as indicated at item 9 (Koel-Simmelink et al. 2014). Also after spinning, a delay of 2 h before storage did not result in changes in CSF protein profiles (Klener et al. 2014; Rosenling et al. 2011), whereas delayed storage of more than 6 h significantly changes peptide profiles (Bruegel et al. 2009). A proton nuclear magnetic resonance study showed stability of myoinositol, glucose, acetate, and alanine levels; a substantial decrease of citrate; and an increase in lactate, glutamine, creatine, and creatinine levels after a 72-h delay in processing of CSF at room temperature (Levine et al. 2000).
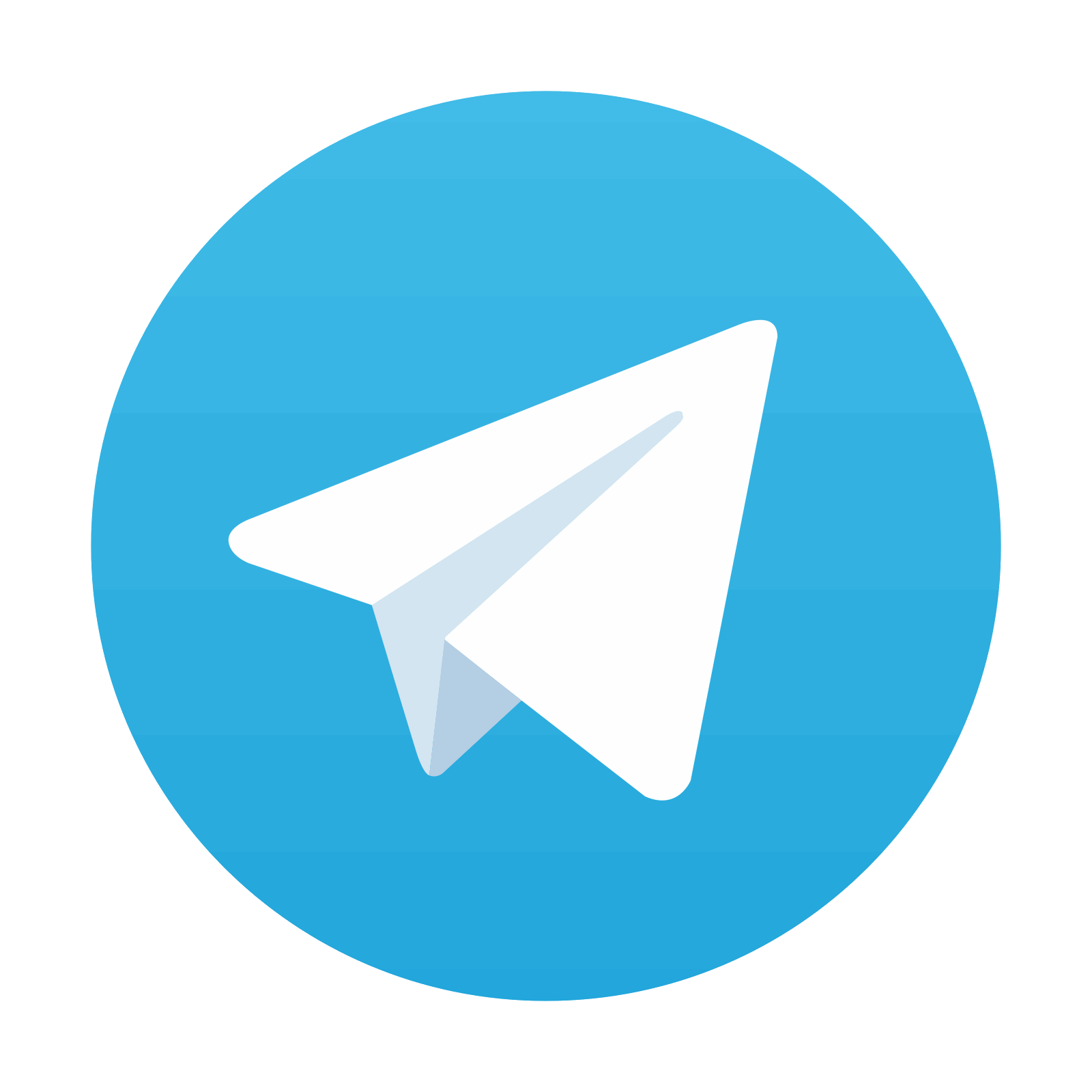
Stay updated, free articles. Join our Telegram channel

Full access? Get Clinical Tree
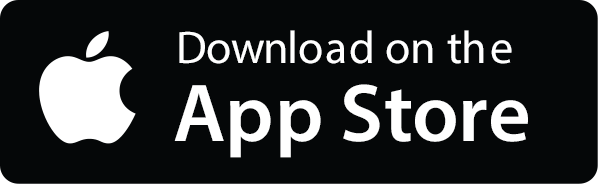
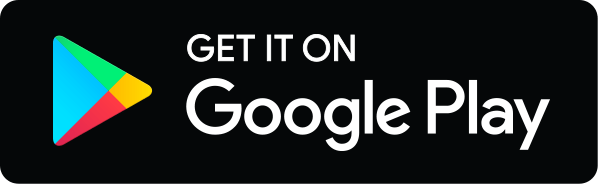