, Nozipho Nyakale1 and Mike Sathekge1
(1)
Nuclear Medicine Department, Steve Biko Academic Hospital, University of Pretoria, Voortrekkers Road and Malan Street, Capital Park, Pretoria, South Africa
Abstract
Impulsivity is a complex and multidimensional trait that represents a core aspect of several neuropsychiatric conditions and, as such, provides an interesting target for diagnosis, appropriate treatment selection and response evaluation.
It is conceived as the result of a variety of dysfunction and dysregulation within an intricate network of neurotransmitter systems, including dopamine (DA), serotonin (5-HT) and noradrenaline (NA). Hence, regional investigations of one single neurotransmitter may not be sufficient to disentangle the pathophysiology of impulsivity. Thus individual variation in limbic striatal D2/D3 receptor availability, diminished functioning of the highly diverse serotonergic system and the variation in norepinephrine density appear to be central to gaining insight into the aetiology and treatment of impulsivity.
Convergent data from neuroimaging and neuropsychology studies consistently point to the aberrations in especially the fronto-striatal, parieto-temporal and fronto-cerebellar networks. Functional imaging with SPECT and PET provides a useful tool in elucidating the neurobiological underpinnings of impulsive aggressive behaviour in ADHD, Parkinson’s disease (PD), bipolar disorder (BP), conduct disorder (CD), substance abuse/dependence and schizophrenia.
Although findings are still heterogeneous, functional brain imaging may provide novel insight into the underlying neural disturbances of pathological impulsivity. In addition, its role in monitoring of treatment response in the various neuropsychiatric disorders, characterized by impulsive behaviour, is emerging as a potentially valuable tool in the long-term management of these patients.
25.1 Overview
25.1.1 Introduction/Definition
“Impulsivity” is a broad term, which in general refers to an inability to resist an impulse, drive or temptation to perform an action. It tends to occur without the necessary expected forethought, planning or consideration of long-term effects and therefore frequently results in unwanted or harmful outcomes to self or others.
The diagnostic and statistical manual of mental disorders (American Psychiatric Association 2000) defines impulsivity as “the failure to resist an impulse, drive or temptation to perform an act that is harmful to the person or to others”.
Impulsive individuals have a tendency to express repetitive deliberate self-destructive acts, such as self-mutilation and suicidal behaviour, and/or outward aggression to others. Impulsivity is considered central to neuropsychiatric conditions such as attention deficit hyperactive disorder in relation to depression and anxiety and cluster B personality disorders, such as antisocial and borderline.
Impulsivity appears to be a complex multidimensional concept (Moeller et al. 2001), which consists of cognitive, behavioural, attention, motor and non-planning aspects for which to date no consensus over a single precise definition exists. Some authors have made a distinction between motor (or behavioural) and cognitive (or choice) impulsivity in an attempt to separate causes from consequences of impulsivity and in an attempt to improve validity of measurements.
In a 2006 review on impulsivity, Estíbaliz Arce and Carmen Santisteban provided an in-depth exploration of the various definitions and aspects of impulsivity (Arce and Santisteban 2006).
The following excerpts are from the aforementioned review:
Kagan (1994) proposed behavioral inhibition as a type of temperament in the child that presents a unique combination of behavioral and physiological responses to novelty. Furthermore, he believed this temperament was associated with future development of anxiety disorders in adulthood. From a behavioral perspective, impulsivity can be defined as “a wide range of actions that are poorly conceived, prematurely expressed, unduly risky, or inappropriate to the situations and that often result in undesirable outcomes” (Evenden 1999).
More simply, it is described as the inability to delay gratification or the inverse of self-control (Monterosso and Ainslie 1999). In the context of experimental behavioural science, impulsivity is commonly viewed as a trait shown by some subjects that, when presented with a variety of outcomes, choose poorer immediate rewards rather than greater delayed rewards (Ainslie 1975). Ho and colleagues include in their definition the importance of punishment, “the selection of small immediate gains in preference to larger delayed gains, or the selection of large delayed penalties in presence to smaller immediate penalties” (Ho et al. 1998).
Brunner and Hen (1997), Evenden (1999), Bechara et al. (2000) and Bechara (2002) have distinguished motor (or behavioural) from cognitive (or choice) impulsivity. The former is usually studied in animals and is equivalent to response inhibition. Cognitive impulsivity is considered the inability to weigh the consequences of immediate and future events and, consequently, delay gratification. This has been measured in tasks of decision-making such as the Iowa gambling task (Bechara et al. 1994).
Brunner and Hen (1997) further distinguish between an impulsive act (behaviour) and impulsivity per se (underlying psychological process) (Brunner and Hen 1997).
From a bio-psycho-social perspective, and in an attempt to combine the various aspects, Moeller, Barratt, Dougherty, Schmitz and Swann (2001) pointed out that a general definition of impulsivity should include the following aspects: “(1) decreased sensitivity to negative consequences; (2) rapid, unplanned reactions to stimuli before complete processing of information; and (3) lack of regard for long-term consequences”. In the context of psychopathology, impulsivity has been defined in three different ways: (1) “swift action without forethought or conscious judgment, (2) behaviour without adequate thought, and (3) the tendency to act with less forethought than most individuals of equal ability and knowledge” (Arce and Santisteban 2006).
The focus of this chapter is on the imaging of impulsivity as its different aspects manifest in the various neuropsychiatric disorders (notably ADHD, PD and the cluster B personality disorders).
25.1.2 Why Is the Study of Impulsivity Important?
Impulsivity forms an integral part of several neuropsychiatric disorders, such as ADHD, Parkinson’s disease (PD), bipolar disorder (BP), conduct disorder (CD), substance abuse/dependence and schizophrenia, or as part of a personality disorder (antisocial and borderline personality disorders). Clearly, these disorders may result in significant functional and occupational impairment with important social and judicial implications.
Assessment of impulsivity forms a crucial part in establishing whether a neuropsychiatric patient poses a potential threat to himself or others. It also provides an important target for choosing the most appropriate treatment and in the subsequent evaluation of treatment response (Moeller et al. 2001).
25.1.3 How Is Impulsivity Currently Diagnosed or Measured?
Non-imaging measurements that are currently available and widely used include self-report measures such as the Barratt Impulsiveness Scale, behavioural laboratory measures and event-related potentials in addition to the history and patient interview.
The most important human measurement tools will be briefly mentioned here as discussed in recent publications by Aichert et al. (2012) and Arce and Santisteban (2006).
However, since the focus of this chapter is on the imaging of impulsivity as a potential measurement tool, readers are referred to the aforementioned publications for more in-depth information.
Self-report measures of impulsivity are useful in allowing measurement of a broad range of cognitive and behavioural styles in different social contexts. The Barratt Impulsiveness Scale is one of the most commonly used self-report measures and consists of a three-factor model that includes both motor and cognitive impulsivity. The scale has 30 items, which are grouped into three subscales of factors: attentional (inattention and cognitive instability), motor (motor impulsiveness and lack of perseverance) and non-planning (lack of self-control and intolerance of cognitive complexity). Due to its simplicity and rapid administration, this instrument has been widely used in studies of bipolar disorder, alcohol and substance use and personality disorders amongst others (Patton et al. 1995).
A variety of experimental, multifaceted paradigms have been developed to assess other components of impulsivity such as the ability to inhibit impulsive or inappropriate responses. These paradigms assess cognitive, motor and emotion disinhibition and delay discounting in reward choices, decision-making processes or time estimation biases.
In their recent publication, Aichert et al. focused on prepotent response inhibition due to the importance of these in the cognitive and clinical neuroscience literature. The table below from the aforementioned article provides a summary of the prepotent response inhibition measures. The complete table also provides neural correlates to which readers are hereby referred to for a more detailed discussion (Aichert et al. 2012) (Table 25.1).
Table 25.1
Non-imaging measurement tools of impulsivity
Task/questionnaire | Characteristics of the task/questionnaire |
---|---|
Antisaccade task | The antisaccade task is considered a measure of oculomotor response inhibition. |
Participants are required to avoid a prosaccade to a sudden onset peripheral target and instead initiate a volitional saccade towards the mirror image position. | |
The key dependent variable of oculomotor response inhibition is the percentage of directional errors. | |
Stroop task | Color words are presented in different ink colors (e.g., green is printed in red ink). |
Participants are required to name the word color and avoid the automated response of reading the word. | |
Go/no-go task | The go/no-go task is considered a measure of selective motor response inhibition. |
Participants are required to respond with a fast motor response when a frequent go-stimulus appears but to withhold the motor response when an infrequent no-go-stimulus is presented. | |
The key dependent variable is the frequency of commission errors, i.e., failures to suppress the response to the no-go stimulus. | |
Stop-signal task | In stop-signal tasks, a stop-signal sometimes appears unpredictably shortly after the go-signal, demanding the later stage inhibition process of interrupting a motor response that is already triggered and under way |
Inhibitory performance in this task is estimated by the latency of the stopping process known as the stop-signal reaction time (SSRT; Logan et al. 2014). | |
BIS-11 questionnaire | BIS-11 (Patton et al. 1995) is a 30-item self-report questionnaire designed to assess trait impulsivity. |
It comprises six first-order factors: attention, motor impulsiveness, self-control, cognitive complexity, perseverance, cognitive instability. | |
These first-order factors were combined to three second-order factors: attentional impulsiveness (inability to focus attention or concentrate), motor impulsiveness (acting without thinking), and nonplanning impulsiveness (lack of “futuring” or forethought). |
The above-mentioned measures of impulsivity suffer from several limitations to varying degrees. There appears to be a lack of clear unidimensional definitions with significant conceptual overlap between self-report and behavioural lab tasks used to measure impulsivity. These issues were explored in a recent publication by Cyders and Coskunpinar (2011). Other methodological problems with the study of impulsivity include the lack of control for potentially confounding variables such as age, IQ, socio-economical status and gender in some instances (Brunner and Hen 1997).
An in-depth discussion of the various limitations of individual assessment tools falls outside the scope of this chapter. It does appear, however, that there is a need for a standard, objective, quantifiable, non-invasive and repeatable diagnostic modality, which corresponds to the underlying brain function. Neuroimaging in the forms of SPECT, PET and functional MRI may provide such a diagnostic or measurement tool.
25.1.4 What Is the Pathophysiology of Impulsivity?
Various anatomical structures and functional areas or circuits have been implicated in the pathophysiology of impulsivity although a complete explanation remains elusive for now. The following structures or areas appear crucial in impulse control: the sub-thalamic nucleus, orbito-frontal, right inferior frontal gyrus and the nucleus accumbens.
The frontal cortex is one of the association cortices and has been implicated in cognitive behaviour and complex motor actions. Lesions in the prefrontal cortex have been shown to produce disinhibition and to cause personality changes. This cortex is also the main source of input to the midbrain serotonergic neurons of the dorsal raphe nuclei. This is important since the serotonin system has been implicated in the evolution of impulsive behaviour (Yang and Raine 2009).
Although many (especially earlier studies) have focused on the role of serotonin regulation, current emphasis appears to have shifted to the importance of the dopaminergic and noradrenergic systems.
25.1.5 The Role of Functional Imaging
Functional imaging has played an important role in establishing the underlying aetiology and pathophysiology in many neuropsychiatric conditions.
Both PET and SPECT imaging allow for the selection of radiotracers based on the aspect of the pathophysiology of interest, which can then be quantified. These include tracers for cerebral perfusion, metabolism, blood-brain barrier integrity testing, neurotransmitters and a multitude of receptor targets. These are then labelled to a radioactive isotope suitable for either PET or SPECT imaging, taking into account the availability, expertise and economic implications. Radioisotopes that are typically used include 11C, 13N, 15O and 18F for PET and 99mTc and 123I for SPECT. Radioisotopes for the imaging of brain neurotransmitter receptors and transporters are based structurally on receptor agonists or antagonists (vast majority of tracers used) and do not illicit pharmacological effects due to the very low (tracer) doses used.
25.1.6 How Can We Image Impulsivity?
The role of dopamine (DA) and its regulation and, to a lesser degree, those of serotonin (5-HT) and noradrenaline (NA) appear central in impulsivity. An important starting point, therefore, would be an overview of the functional imaging of these neurotransmitters and their receptors.
25.1.6.1 Dopaminergic Neurotransmission
The function of dopamine (DA) in the brain includes the mediation of cognition, emotion and movement, and it is involved in both the production and inhibition of several primary biological drives. Removal of free dopamine from the synaptic cleft is one of the primary mechanisms for regulating dopaminergic tone, with the reuptake of free dopamine from the synaptic cleft mediated by a macromolecular transporter located in the axonal membrane (DAT).
The majority of neuropsychiatric disease and drugs cause compensatory changes in the dopamine transporter before affecting the concentration of the postsynaptic dopaminergic receptors. These striatal transporters are present exclusively on dopamine-producing neurons where they play a key role in the regulation of DA levels in the synaptic cleft. Dopamine transporters are markers of the integrity of presynaptic dopaminergic neurons and as such provide an ideal target for imaging with either SPECT or PET. Figure25.1 below provides an overview of the most important functional imaging targets and some of the more commonly used SPECT and PET tracers (Fusar-Poli et al. 2012).
There is growing evidence to suggest that impulsiveness is caused by dysregulation of brain dopaminergic neurotransmission (Dalley et al. 2008). Areas that are often implicated include the dopaminergic neuronal projections from the midbrain to the ventral striatum (nucleus accumbens). These are associated with the integration of motivational and reward-based processing (Cardinal et al. 2004).
There has been rapid growth in the literature describing the dopaminergic basis of human behaviour, with a parallel increase in tracer development for quantification of dopamine release via D2/D3 receptor occupancy imaging.
In a recent publication by Reeves et al. (2012), the association between reduced striatal D2/D3 receptor availability and higher levels of impulsivity was explored in 23 healthy volunteers. The authors wanted to evaluate whether a relationship between limbic (ventral) striatal D2/D3 receptor availability and the individual components of impulsivity exist. They made use of 11C-raclopride PET imaging in 23 healthy volunteers which was compared to the various components of impulsivity (attention, motor and non-planning), assessed using the Barratt Impulsiveness Scale. After the exclusion of potential dissimulators, a significant association was found between non-planning impulsiveness and limbic D2/D3 receptor availability. The authors concluded that non-planning impulsiveness may be associated with individual variation in limbic striatal D2/D3 receptor availability and that different facets of impulsivity may have specific neurochemical correlates (Reeves et al. 2012).
In another recent study, Costa et al. (2013) investigated the dopaminergic basis of impulsivity and other ADHD-related traits in healthy individuals by evaluating the association of such traits with striatal dopamine transporter availability. The group imaged 38 healthy males with 123I-FP-CIT SPECT; they measured impulsivity with the Barratt Impulsiveness Scale (BIS) and hyperactivity–impulsivity and inattention with the Adult ADHD Self-Report Scale (ASRS). The authors found that greater dopamine transporter availability was associated with higher impulsivity as measured on BIS but was not associated with ADHD-related traits. This association with BIS was significant even after accounting for individual differences in age and neuroticism. The authors suggested in conclusion that individual differences in the dopamine system may be a neural correlate of trait impulsivity in healthy individuals (Costa et al. 2013).
25.1.6.2 Serotonergic Neurotransmission
The serotonergic system is one of the most important and complex neurotransmitter systems in the human brain, with 14 receptors identified to date, many of whose functions remain elusive and many of which play a role in several different processes. Serotonin transmission also plays an important role in several neuropsychiatric conditions and has been strongly implicated in impulsivity, together with the dopaminergic system.
Extensive investigation of the role of serotonin followed the discovery that the metabolite 5-HIAA is often low in the CSF of people with impulsive and aggressive behaviour. The incidence of suicidal acts was also related to the level of 5-hydroxyindoleacetic acid (5HIAA) in the cerebrospinal fluid, where patients with low levels of 5-HIAA (below 15 ng/ml) attempted suicide significantly more often than those with high levels and used more violent means (Asberg et al. 1976) (Fig. 25.2).
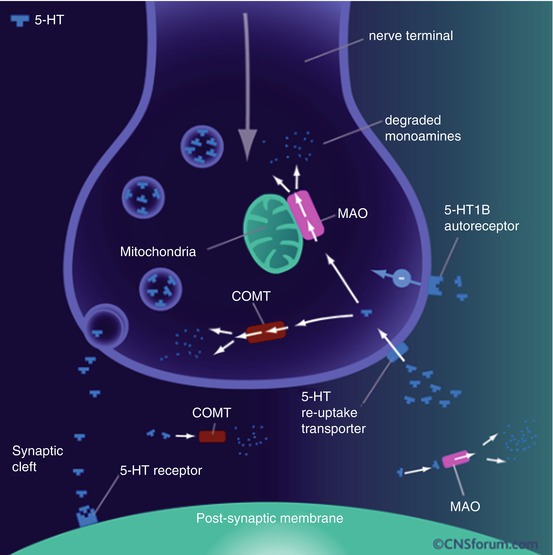
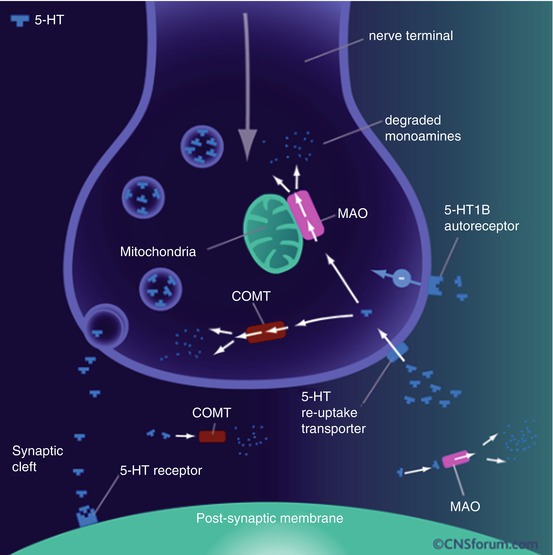
Fig 25.2
5-HT neurotransmission
In the CNS, 5-HT is synthesized in the raphe nuclei of the human midbrain and brainstem as well as their projection sites. From the raphe nuclei, projections run to the forebrain, reaching nearly all parts of the brain. Tryptophan hydroxylase (TPH) plays an important role in the synthesis of serotonin by synthesizing l-tryptophan to 5-hydroxy-l-tryptophan, which in turn is synthesized by amino acid decarboxylase to serotonin. Serotonin reuptake from the extracellular space and the synaptic cleft is carried out by the SERT, which is an important therapeutic target in the treatment of many psychiatric disorders. The vesicular monoamine transporter type 2 (VMAT2) is a non-serotonin-specific transporter, which also transports other neurotransmitters such as dopamine, norepinephrine and histamine. The enzyme monoamine oxidase A (MAO-A) is in charge of degrading serotonin, and the resulting aldehyde is oxidized by aldehyde dehydrogenase to 5-hydroxyindoleacetic acid (5-HIAA).
Paterson and co-workers (2013) reviewed the history and current status of radioligands used for both SPECT and PET imaging of the human serotonin receptors, the serotonin transporter (SERT) and 5-HT synthesis rate. The following table has been taken from their paper and provides a nice summary of the imaging options and studies so far (Paterson et al. 2013) (Table 25.2).
Table 25.2
Promising SPECT and PET tracers for serotonergic imaging
Target | Radioligand | First in man | Animal studies | Human studies | Research institutions |
---|---|---|---|---|---|
5-HT1A | [11C]WAY-100635 | 1995 | 10 | 80 | 12 |
[18F]MPPF | 2000 | 27 | 21 | 6 | |
[18F]FCWAY | 2000 | 7 | 11 | 1 | |
[11C]CUMI-101 | 2008a | 2 | 1a | 1 | |
5-HT1B | [11C]AZ10419369 | 2008 | 3 | 1 | 1 |
[11C]P943 | 2009 | 1 | 3 | 1 | |
5-HT2A | [123I]-5-I-R91150 | 1997 | 9 | 19 | 6 |
[18F]setoperoneb | 1990 | 3 | 36 | 7 | |
[18F]altanserin | 1994 | 5 | 51 | 10 | |
[18F]deuteroaltanserin | 1998 | 2a | 5a | 1 | |
[11C]MDL100907 | 1998 | 5 | 6 | 5 | |
5-HT4 | [11C]SB207145 | 2008 | 3a | 3a | 2 |
5-HT6 | [11C]GSK215083 | 2008 | 1 | 2 | 1 |
SERT | Beta-[123I]CITb | 1993 | 20 | 87 | 18 |
[123I]ADAM | 2005 | 11 | 28 | 12 | |
[11C]DASB | 2000 | 17 | 48 | 16 | |
[11C]MADAM | 2005 | 1 | 7 | 2 | |
5-HT | [11C]-AMT | 1997 | 2c | 17d | 2 |
Synthesis | [11C]-HTP | 1991a-d | 4 | 10 | 3 |
Saulin et al. (2012) published a review, which summarizes the distribution and main functions of the serotonin (5-hydroxytryptamin, 5-HT) receptors as well as that of the serotonin transporter (SERT, 5-HTT), the vesicular monoamine transporter 2, monoamine oxidase type A and 5-HT synthesis in the human brain. They included recent advances in in vivo quantification of these different receptors and enzymes that are part of the serotonergic system using PET. A summary from their publication can be found below (Saulin et al. 2011) (Table 25.3).
Table 25.3
Overview of frequently used PET radioligands for measurement of the serotonergic system in the human brain, as well as recent promising radioligands from animal and human PET studies
Receptor/transporter/enzyme | Frequently used PET ligands in humans | Recent promising PET ligands (animal, humans) | References |
---|---|---|---|
5-HT1A | [Carbonyl–11C]-WAY | [18F]MefWAY (primate) | Wooten et al. (2011) |
[11C]WAY100635 | [11C]CUMI-101, (baboon, Papio anubis, human) | ||
5-HT1B | [11C]AZ10419369 | – | Varnäs et al. (2011a) |
[11C]P943 | Murrough et al. (2011) | ||
5-HT1C | – | – | – |
5-ht1E | – | – | – |
5-HT1F | – | – | – |
5-HT2A | [18F]altanserin | [11C]CIMBI-36 (pig) | Ettrup et al. (2011) |
[11C]MDL100,907 | (R)-[18F]MH.MZ (rodent) | Debus et al. (2010) | |
5-HT2B | – | – | – |
5-HT2C | – | – | – |
5-HT3 | – | – | – |
5-HT4 | [11C]SB207145 | [11C]13 (guinea-pig) | Xu et al. (2010) |
5-ht5 | – | – | – |
5-HT6 | – | [11C]GSK210583 (pig, human) | Parker et al. (2012) |
5-HT7 | – | – | – |
SERT | [11C]DASB | [18F]FPBM (rat) | Wang et al. (2010) |
[11C]MADAM | [18F]ADAM (rat, monkey) | Huang et al. (2010) | |
VMAT2 | [11C]DTBZ | [18F]AV133 (mouse, human) | Zhu et al. (2010) |
[11C]MTBZ | Okamura et al. (2010) | ||
MAO-A | [11C]harmine | [11C]RS 2360 (mouse) | Soliman et al. (2011) |
[11C]clorgyline | Fowler et al. (2005) | ||
[11C]befloxatone | Bottlaender et al. (2003) | ||
De Bruyne et al. (2010) | |||
5-HT synthesis | [11C]AMT | – | Visser et al. (2011) |
[11C]5-HTP | |||
Endogenous 5-HT | [18F]MPPF | – | Derry et al. (2006) |
Yatham et al. (2001) | |||
Varnäs et al. (2011b) |
Saulin and co-workers (2011) mention the following in their review, which relates to impulsivity.
In 5-HT1B knockout mice, an increase in impulsive behaviour and defective regulation of impulsivity was observed (Meneses 2007; Meneses and Perez-Garcia 2007).
The 5-HT1B receptor has been implicated in various psychiatric disorders. Studies have found it to be involved in alcoholism (Hu et al. 2010; Soyka et al. 2004) and substance abuse (Huang et al. 2003; Neumaier et al. 2002) (but see Cigler et al. (2001) for different findings). It has also been implicated in disorders such as attention deficit hyperactivity disorder (ADHD) (Quist et al. 2003) and aggression (Olivier and van Oorschot 2005; Saudou et al. 1994). In studies by Knudsen and co-workers, OCD has been linked to elevated levels of 5-HT2A binding in the caudate nucleus (Adams et al. 2005), as well as to Tourette’s syndrome which was associated with increased binding in orbitofrontal cortex, anterior cingulate, frontal cortex and other regions of the brain (Haugbøl et al. 2007).
By means of the highly specific radioiodinated 5-HT2A receptor antagonist 4-amino-N-[1-[3-(4-fluorophenoxy) propyl]-4-methyl-4-piperidinyl]-5-iodo-2-methoxybenzamide or 123I-5-I-R91150, Audenaert and co-workers demonstrated with high-resolution SPECT that the 5-HT2A in deliberate self-harm patients was significantly reduced in the frontal cortex (after correction for age) when compared with healthy controls. They also found that the reduction was more pronounced amongst deliberate self-injury patients (Audenaert et al. 2001).
Rylands et al. also conducted a study in which 11C-MDL100907 was used to measure the availability of 5-HT 2A receptor in males with impulsive aggression. These patients fulfilled the DSM-IV diagnostic criteria of antisocial personality disorder (ASPD) and borderline personality disorder (BPD) and were divided into a high-impulsive aggression (high-IA) and a low-impulsive aggression (low-IA) group. Those with a history of past alcohol and drug dependence, current or past DSM-IV Axis I disorder, current Axis II cluster A personality disorder or use of 3,4-methylenedioxymethamphetamine (ecstasy) were excluded from the study. PET imaging was performed, which demonstrated that 5-HT 2A receptors were lower throughout the brain cortex of highly impulsive individuals. This indicates abnormal postsynaptic mechanisms of serotonin in these impulsive patients (Rylands et al. 2012).
Abnormalities of 5-HT2 receptors in the brain cortex are viewed as representative of 5-HT2A receptors in cortex due to the extremely low density and binding of the other two subtypes, 5-HT2B and 5-HT2C (Meyer et al. 2008).
In schizophrenia, increased binding was observed in the caudate nucleus (Erritzoe et al. 2008), but decreased levels were found in the dorsolateral prefrontal cortex (dlPFC) and the para-hippocampal gyrus (Burnet et al. 1996). Moreover, the 5-HT2A polymorphism-1438G/A has been suggested to play a role in OCD (Enoch et al. 1998).
Most prominently in the clinical population (for review, see Daws and Gould (2011)), SERT has been found to play a major role in depression (e.g. Tsao et al. 2006) and bipolar disorder (e.g. Lesch et al. 1995). Other studies suggested SERT to be implicated in OCD (Hesse et al. 2011; Simpson et al. 2003) and substance abuse (Brown et al. 2007), especially MDMA (McCann et al. 2005; Selvaraj et al. 2009). SERT has further been linked to obesity (Erritzoe et al. 2010) and Parkinson’s disease (e.g. Wang et al. 2010).
Changes in 5-HT synthesis have been associated with the treatment of major depression, showing lower 5-HT synthesis rates in patients with major depression (Rosa-Neto et al. 2004). Decrease of tryptophan has also been associated with Parkinson’s disease, especially in the caudate (Kish et al. 2008). Leyton and colleagues found borderline personality disorder to be negatively linked to 5-HT synthesis rates as measured with PET in the medial frontal gyrus, anterior cingulate gyrus, superior temporal gyrus and corpus striatum. They also found reduced synthesis rates in the medial frontal gyrus, anterior cingulate gyrus, temporal gyrus and striatum in subjects scoring high on measures of impulsivity (Leyton et al. 2001).
Drug-free suicide attempters compared with matched controls showed a negative correlation between SERT and impulsiveness with no significant difference demonstrated concerning the regional levels of SERT binding potentials and DAT binding potentials in the two groups. However, a significant regional correlation was seen in the suicide attempters between Marke–Nyman temperament test (which assesses the level of impulsiveness and mental energy) and the SPECT findings. This finding was not evident in the control group and was attributed to an inability of the suicide attempters to regulate their serotonin and dopamine levels in response to external stress (Ryding et al. 2006).
123I − β − CIT, a mixed monoamine transporter tracer which is a potent ligand for both dopamine and serotonin reuptake sites, was used by Lindstrom et al. to evaluate 12 patients (10 men and 2 women) who had previously had a serious suicide attempt and their matched healthy controls. SPECT imaging demonstrated a correlation between SERT and DAT in the suicide attempters which was not evident in the control group indicating that both systems may play a role in impulsivity (Lindström et al. 2004).
25.1.6.3 Noradrenergic Neurotransmission
The noradrenaline transporter (NAT) is located at the presynaptic terminal of noradrenergic neurons where it plays an integral role in noradrenergic neurotransmission. It regulates the concentration of noradrenaline in the synaptic cleft via a reuptake mechanism. Brain structures known to be rich in norepinephrine (NET) include the locus coeruleus, thalamus, hippocampus and throughout the cerebral cortex, whereas low levels are found in cerebellum and striatum.
Alterations in synaptic noradrenaline levels have been implicated in various neuropsychiatric and neurodegenerative disorders (such as ADHD, anxiety and Alzheimer’s disease. More importantly, NAT is a major therapeutic target for these disorders and provides SPECT and PET with an imaging target to assess drug occupancy levels, amongst other applications (Fig. 25.3).
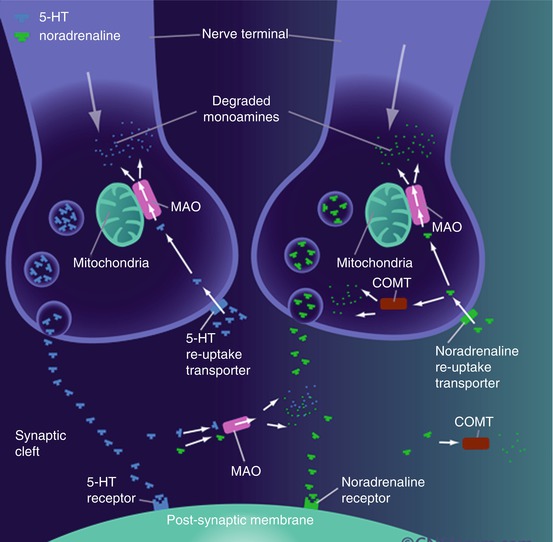
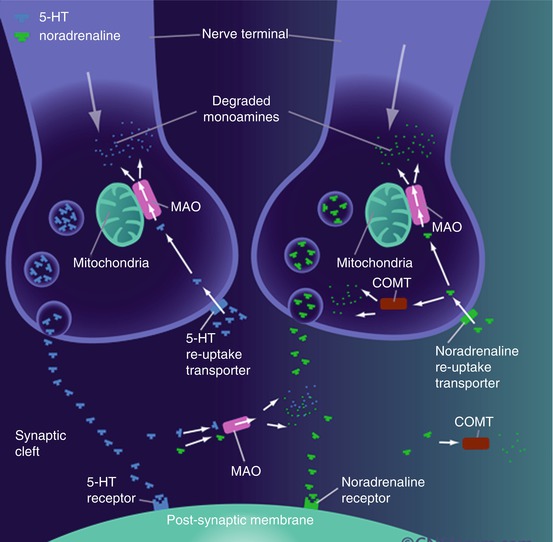
Fig 25.3
Noradrenergic neurotransmission
Most of the initial work on SPECT radiotracers for imaging of the noradrenaline transporter (NAT) has focused on the development of radioiodinated analogues of the noradrenaline reuptake inhibitor, reboxetine. It has lagged behind the tracer development for DAT and SERT somewhat, due to limited availability of suitable radioligands. A few tracers that are selective for NET have recently emerged with several research groups having found the reboxetine derivatives preferable to the nisoxetine series.
Tamagnan and co-workers (2007) worked on the development of SPECT imaging agents for the norepinephrine transporter. They synthesized a series of reboxetine analogues, which was then evaluated for in vitro binding as racemic mixtures. [123I]INER was considered to be the best candidate and was synthesized as the optically pure (S,S) enantiomer. The in vivo binding of [123I]INER was determined by SPECT imaging in an animal model, and the authors found that the tracer’s in vivo specificity, selectivity and kinetics made it a promising agent for SPECT imaging of norepinephrine in vivo (Tamagnan et al. 2007).
Jobson and co-workers (2008) developed a new route for the synthesis of iodinated reboxetine analogues to image the noradrenaline transporter (NAT) with SPECT. The authors prepared 2S,3S- and 2R,3R-iodoreboxetine and performed biological testing against various monoamine transporters, which demonstrated these compounds to be potent and selective for NAT (Jobson et al. 2008).
A specific tracer for quantification of the variation in norepinephrine density will be valuable in gaining insight into the aetiology and pathophysiology of neuropsychiatric disorders such as ADHD and Alzheimer’s. This will especially be of value in identifying therapeutic targets and monitoring treatment response in newly emerging drug treatments, such as atomoxetine. This is the first selective, non-stimulant treatment for ADHD.
25.1.6.4 The Potential Role of PET and SPECT in Evaluating Response to Treatment, Prognosis and Drug Development
Although clinical application may include a primary role in diagnosis, the more promising role of functional imaging seems to be in the fields of drug development, in the evaluation of treatment response and in providing an idea of the severity and prognosis of these conditions.
In addition to the role of functional neuroimaging in the assessment of neural connections involved in impulsivity, several authors have also reported the potential role of molecular imaging in treatment response assessment. By labelling an appropriate tracer with an affinity and selectivity for the molecular target in question to a suitable radioisotope, it is possible to evaluate the kinetics, biodistribution, metabolism and toxicity of particular drugs (Guilloteau and Chalon 2005).
New et al. used 18F FDG-PET/CT to assess metabolic changes in specific cortical areas in patients with impulsive aggression following treatment with selective serotonin reuptake inhibitors (SSRIs). This group demonstrated increased metabolism in the prefrontal and medial temporal regions (commonly implicated in impulsive aggression) in patients treated with fluoxetine. These patients demonstrated decreased tracer uptake in the aforementioned areas on their baseline scans during their treatment-naive state (New et al. 2004).
Rosa-Neto et al. and Krause and co-workers also used molecular imaging in assessing response to methylphenidate in patients diagnosed with ADHD (Rosa-Neto et al. 2004; Krause et al. 2000). Dose-dependent occupancy of DAT with methylphenidate had been demonstrated by Volkow et al. (1998). Therapeutic doses of this drug have been shown to increase extracellular dopamine concentration. Using 11C-raclopride PET, Rosa-Neto et al. went on to demonstrate a 12 % reduction in the binding potential of striatal dopamine D2/D3 receptors during treatment with methylphenidate compared to the baseline pretreatment assessment of adolescents with ADHD. The binding potential assessed with 11C-raclopride PET was found to correlate with the cognitive measures using the TOVA test. They further demonstrated a correlation between the severity of the impulsivity and the magnitude of the binding potential of 11C-raclopride in the striatum of these patients, suggesting a potential role in the evaluation of the prognosis of patients with impulsivity with molecular imaging (Rosa-Neto et al. 2004).
The role of molecular imaging may further be extended to the development of drugs for neuropsychiatric disorders.
This was discussed by Guilloteau and Chalon who explored the potential role of PET and SPECT imaging through in vivo exploration of monoamine transporters. (These are believed to be responsible for the homeostasis of the neurotransmitter pools at the nerve endings.) The authors concluded that in vivo molecular imaging could be used to define the involvement of a specific neurotransmitter and therefore to explain the mechanism of action of drugs through binding site occupancy studies. This therefore makes it possible to evaluate disease evolution and therapeutic effects of treatment and to elucidate the mechanism of action of new drugs (Guilloteau and Chalon 2005).
25.2 Clinical Applications
As mentioned before, impulsivity represents a core aspect of several neuropsychiatric conditions and, as such, provides an interesting target for diagnosis, appropriate treatment selection and response evaluation. Attention deficit hyperactivity disorder (ADHD) is considered by many to be the “archetypal disorder of impulsivity”, and as such it was considered an appropriate starting point for discussion. It should also serve as a model to illustrate the role of functional imaging in all of the aforementioned aspects of neuropsychiatric disease evaluation.
25.2.1 Neurology
25.2.1.1 Attention Deficit Hyperactivity Disorder (ADHD)
ADHD is a common and debilitating neuropsychiatric disorder, which frequently starts in childhood and is characterized by age-inappropriate symptoms of hyperactivity, inattentiveness and impulsivity. According to the fourth edition of the Diagnostic and Statistical Manual (DSM-IV), there are three subtypes of ADHD: the predominantly inattentive subtype, the predominantly hyperactive–impulsive subtype and the combined subtype. However, evidence from various neuropsychological studies suggests that “poor inhibitory control is central to ADHD” (Durston 2003).
The understanding of the underlying pathophysiology is complicated by the variety of clinical symptoms with which the condition may present as well as the high incidence of comorbidity. Imaging studies of the past 20 years have shown aberrations in especially the fronto-striatal, parieto-temporal and fronto-cerebellar networks.
25.2.1.2 Pathophysiological Changes
Several anatomical studies have shown diffuse volume reductions, which affect the cerebrum and cerebellum. Studies of the basal ganglia have resulted in inconsistent results with some reporting reductions in the right caudate volume and others in the left and no changes noted in the putamen (Castellanos 1996). A meta-analysis by Nakao et al. (2011) has demonstrated that low grey matter in the basal ganglia is the most consistent abnormal finding (Nakao et al. 2011).
The central role of striatal dopamine levels appears undisputed. Hypotheses have linked cognitive impairments to a decrease in DA availability in the prefrontal cortex, while hyperactivity and impulsivity have been linked to a secondary increase in striatal DA (Solanto 2002).
A 2003 review by Sarah Durston provides a comprehensive overview on the anatomical and functional information obtained from various imaging studies prior to 2003, which is conveniently grouped and summarized in table form and which readers are hereby referred to (Durston 2003).
In a recent meta-analysis by Fusar-Poli et al. (2012), the authors evaluated the inconsistent results so far on striatal dopamine receptor density by combining the results of nine nuclear medicine imaging studies, involving either PET or SPECT.
This resulted in a total of 169 ADHD patients with 173 normal controls matched for age, gender and IQ. They extracted striatal-binding potentials (BP) together with clinical, demographic and methodological variables from all publications. However, significant heterogeneity was present and the authors had to use a random-effects model. No publication bias was present and the robustness was proven by sensitivity analysis.
The following studies were included (Table 25.4):
Table 25.4
PET or SPECT studies included in a meta-analysis of striatal dopamine transporter density in ADHD patients and healthy comparison subjects
Study and group | Radiotracer | Technique | N | Age (years) | ADHD treatment status | ADHD/comparison ratio of dopamine transporters | ||
---|---|---|---|---|---|---|---|---|
Total | Female | Mean | SD | |||||
Dougherty et al. (1999) | [123I]altropane | SPECT | Drug-free | 1.70a | ||||
ADHD | 6 | 4 | 41.33 | 4.46 | ||||
Comparison | 30 | — | 40.80 | —b | ||||
van Dyck et al. (2002)
![]() Stay updated, free articles. Join our Telegram channel![]() Full access? Get Clinical Tree![]() ![]() ![]() |