div class=”ChapterContextInformation”>
7. Neuroimaging in Cluster Headache and Trigeminal Autonomic Cephalalgias
7.1 Introduction
Within the last 20 years, the tremendous progress of neuroimaging techniques has provided an unprecedented impact on the comprehension of pathological processes at the basis of several neurological conditions.
Cluster headache has greatly benefitted from these technical and theoretical advancements: neuroimaging indeed shifted the core understanding of this neuropathological condition from neurovascular mechanisms to dysfunctions of the central nervous system. Here, we present a comprehensive review of the neuroimaging studies that have revolutionized the comprehension of this neuropathology.
7.2 Structural Imaging
7.2.1 Voxel-Based Morphometry
Voxel-based morphometry (VBM) is a widely used method based on high-resolution MRI images, which aims at identifying focal morphometric changes in grey and white matter volume. Simply speaking, VBM is a comparison of grey and white matter concentrations between two groups of subjects [1]. To function well, high-resolution images of the single subjects have to be realigned and warped to ensure congruity of brain regions between subjects. VBM is further based on voxel-based image segmentation into grey and white matter images taking into account intrinsic intensity information of the single volumes as well as a priori information and is thus strongly depending on grey and white matter contrast of the respective images. Consequently, VBM is an apt method for cerebral structures, while performance on the level of the cerebellum and brainstem is poor. Furthermore, VBM is susceptible to a lot of confounders, such as poor image realignment or misclassification of tissue types [2]. Although grey matter changes found using VBM are usually interpreted as a local increase or decrease in grey matter volume and thus as a marker of neuronal density and plasticity, it is in fact not clear what is really the structural correlate to the so-called VBM grey matter changes. Nonetheless, VBM is until today widely used in pain and headache research.
VBM has a long history in cluster headache: it was in fact the first method ever to be used to depict changes in brain structure in cluster headache patients [3] and has since been used in multiple consecutive studies. Back in 1999, May and colleagues were able to correlate functional changes observed within the posterior hypothalamic area in acute cluster headache attacks with bilateral grey matter changes in the same area [3] in cluster headache patients both within and outside the bout. This very important early study in the field of VBM had—taken together with the functional imaging results—high therapeutic impact: in the following years, over 50 otherwise intractable cluster headache patients were treated successfully with deep brain stimulation of the posterior hypothalamic area [4–7]. This study was over the next nearly 20 years followed by various other studies: Matharu et al. conducted a VBM study of 66 episodic cluster headache patients and 96 healthy controls but did not find any structural alterations between cluster headache patients and control participants despite the fact that they used a predefined hypothalamic region of interest for statistical small volume correction. Findings of the more recent studies present a multifaceted image of structural changes in cluster headache: the most common finding is grey matter volume changes in areas unspecifically involved in pain processing and modulation of aversive stimuli, among these the thalamus, insular and cingulate cortex, cerebellum, temporal lobe, hippocampus, and frontal cortex [8–10]. Naegel et al. showed these changes to be dynamic and depending on the disease and pain state regarding their direction, location, and extent [8].
None of the studies were able to replicate the posterior hypothalamic volume changes. One possible reason could be the differences in the software used for analysis: SPM (http://www.fil.ion.ucl.ac.uk/spm) as well as the VBM toolbox for SPM have been gradually updated with huge impact on the normalization and segmentation procedures which crucially influence results in a relatively small area as the hypothalamus. However, very recently, Arkink et al. found increased grey matter values in the anterior part of the hypothalamus in chronic cluster headache patients as compared to healthy controls [11]. This VBM analysis was amended by a volume comparison of the manually segmented anterior hypothalamus leading to the finding of increased anterior hypothalamic volume in chronic as well as episodic cluster headache. Taken together, very early and very recent VBM analyses suggest some structural alterations within the hypothalamus in cluster headache patients, whereas other VBM studies in cluster headache found more unspecific changes in general pain processing areas.
7.2.2 Diffusion Tensor Imaging
The evolution of the magnetic resonance imaging (MRI) techniques has provided an extraordinary tool to characterize the microstructural organization of biological tissue in vivo: diffusion tensor imaging (DTI) [12]. DTI maps the magnitude and directionality of the water molecules diffusion by means of the diffusion tensor model. There are four major parameters that can be computed from the diffusion tensor in each voxel: mean diffusivity (MD), fractional anisotropy (FA), radial diffusivity (RD), and axial diffusivity (AD). Based on these parameters, it is possible to detect microstructural alterations of the white matter and to understand whether the investigated structures present de-myelination or dys-myelination, although some doubts still persist about the exact interpretation of these measures [13, 14]. Remarkably, the principal direction of the diffusion tensor can be used to perform tractography, a technique that allows revealing the anatomical connectivity of the brain [15].
Despite the putative importance of the study of white matter microstructural alterations in the cluster headache pathophysiology, these investigations are few and, due to the puzzling results, not conclusive. In particular, in one of the first DTI studies, Absinta et al. [9], using a 3T MRI scanner, published a convincing evidence of the absence of white matter alterations in a convenient sample of episodic cluster headache patients during the “out-of-bout” condition. However, subsequent investigations, all conducted with a 1.5T MRI scanner and mainly in small samples of patients, showed the widespread presence of microstructural alterations of the white matter in episodic cluster headache. The first study [16] of these series showed significant FA changes in frontal and subcortical areas (amygdala, hippocampus, thalamus, and basal ganglia) and in the brainstem in a small sample of episodic cluster headache individuals, mainly in “out-of-bout” condition. The authors speculated that these white matter microstructural alterations indicated pain processing abnormalities, suggesting a possible key role in the cluster headache pathophysiology of the alterations of the descending pain inhibitory pathways. Remarkably, they interpreted the alterations observed in the brainstem as abnormalities of the medial lemniscus and of the nucleus tractus trigemini, involved in the modulations of the trigemino-sensory pathways. Interestingly, the alterations observed in the upper brainstem were linked to lesions of the sympathetic pathway. Altogether these results support the well-recognized involvement of the sympathetic and trigeminal systems in the cluster headache pathophysiology, but also a role of the pain processing pathways, suggested in several neuroimaging studies [17–19].
Along the same lines, the work of Szabó et al. [20] reported widespread alterations of the white matter across all the brain areas (i.e., in the frontal, parietal, temporal and occipital lobes) in patients with episodic cluster headache. More recently, Király et al. [21] investigated white matter microstructural alterations of the subcortical structures in two different groups of patients with left or right episodic cluster headache. Interestingly, the data from these patients were not merged in a unique sample, due to the observed differences in diffusivity parameters between the left and right hemisphere. In line with the hypothesis of the involvement of the subcortical structures in pain processing, they found evidence of microstructural alterations in the right amygdala, caudate, and pallidum. Chou et al. [22] showed white matter alterations in a group of episodic cluster headache patients during “in-bout” and “out-of-bout” periods. Using tract-based spatial statistic (TBSS), they found microstructural alterations in frontal (medial prefrontal gyrus, in subgyral area of the frontal lobe), limbic (hippocampus/amygdala, insula), and cerebellar areas. These areas play an important role in the processing of the cognitive and affective dimensions of the painful experience; clearly, this result provides further support for the mass of neuroimaging data showing structural and functional alterations in the regions involved in pain processing [3, 16, 23, 24]. Notably, the observed alterations were present in both “in-bout” and “out-of-bout” conditions, suggesting possible stable white matter abnormalities. Very remarkably, the authors observed direct anatomical connections between these altered white matter areas and the ipsilateral hypothalamus. This important result shows, again, a key role of the hypothalamus in the cluster headache pathophysiology. In the light of the negative findings of the work of Absinta et al. [9] conducted with a 3T MRI scanner in a relatively large sample of patients and the different results obtained by other studies conducted with a 1.5T MRI scanner [20–22, 25], future investigations are needed to identify global microstructural white matter alterations in the cluster headache pathophysiology.
Remarkably, some studies were dedicated to the investigations of the anatomical circuits at the basis of successful hypothalamic deep brain stimulation (DBS). Although DBS of the hypothalamic region is successful in the treatment of more than 60% of patients implanted for drug-refractory cluster headache [26], the stimulated anatomical and functional networks at the basis of this efficacy are not well understood. To identify the cerebral networks associated with the DBS targets in chronic cluster headache, Clelland et al. [27] used DTI. Two important results were observed: (1) the tips of the electrodes for DBS were located in the midbrain tegmentum, near the third ventricle, and posterior to the hypothalamus, as previously suggested [28, 29]; (2) the DBS targets project to three main regions: the ipsilateral hypothalamus, reticular formation, and cerebellum. The observed anatomical projections from the DBS target to the ipsilateral hypothalamus are an important proof of concept that links the neuroimaging data, showing the activity in midbrain [30] and hypothalamic regions [24] during the attacks, with clinical, neuroendocrinological, and animal findings providing converging evidence of the hypothalamic involvement in cluster headache pathophysiology [26, 31]. Importantly, a direct pathway between the hypothalamus and cerebellum was evidenced in a previous DTI study [32]; this fits well with the observed abnormal functional connectivity between the hypothalamus and cerebellum [33, 34], and with the observation that the cerebellum and the hypothalamus/midbrain tegmentum are activated during the attacks [24, 30]. The cerebellum was suggested to be part of the pain processing network playing an important role in the nociceptive modulation [35]. Remarkably, the observed data are very consistent with studies evidencing projections from the DBS electrode target to the cerebellum and the reticular nucleus [36, 37].
7.3 Functional Imaging
7.3.1 Single-Photon Emission Tomography and Positron Emission Tomography
In functional neuroimaging, fluorodeoxyglucose-positron emission tomography (FDG-PET) is often used to measure brain metabolism. By using methods of statistical parametric mapping, groups of subjects can be compared regarding the distribution of areas with heightened glucose metabolism. Areas with such a heightened metabolism are usually interpreted as being activated in the respective group or under a respective condition. It is thus possible to identify and localize brain activations typical for a certain group of patients or for a certain condition within one group of patients (e.g., cluster headache patients inside of an attack). While FDG-PET is a widely used method in neuroimaging as an unspecific marker of brain activity, there are a lot of specific ligand-PET variants, in which radioactively marked ligands are used to measure, e.g., receptor density within certain parts of the brain [38]. Single-photon emission tomography (SPECT) on the other hand is a method that in scientific neuroimaging in the headache field has become gradually less influential. Reasons might include the poor spatial resolution and the fact that it has never really been used in voxel-based analyses in the headache field [39].
There are currently only a few SPECT studies in cluster headache available, all of which have been conducted prior to the broad establishment of voxel-based analyses. Results are mostly contradictory: whereas some studies did not find any differences in mean cerebral blood flow (CBF) when comparing the acute attack state with the state outside of attacks [40–42], other studies have found a heterogeneous pattern (increases in some patients and decreases or no changes in others during acute cluster headache attacks) [43, 44] or an increased CBF during acute attacks [45]. There is only one SPECT study to date that has not focused on overall CBF changes during attacks but has used a case-control design to compare “out-of-bout” cluster headache patients with healthy controls [46]. CBF was lower in the contralateral primary somatosensory cortex and motor cortex as well as in the thalamus. All in all, while providing some early attempts on capturing brain activity changes in cluster headache, SPECT studies have as yet not provided much insight into cluster headache pathophysiology.
Regarding other trigeminal autonomic cephalalgias, evidence from SPECT imaging is limited to two case reports: In paroxysmal hemicrania, hypoperfusion was detected bilaterally in the frontoparietal region between attacks with complete normalization of rCBF within attacks [47], whereas in two SUNCT patients, perfusion was normal during attacks [48].
Positron emission tomography (PET) on the other hand is a still widely used functional imaging method that had its main significance in the early functional studies in cluster headache. The possibility of performing voxel-based analyses in combination with PET allowed for a distinct attribution of changes in cerebral blood flow to certain areas of the brain and brainstem. Back in 1996, Hsieh et al. investigated a group of four episodic cluster headache patients during induced attacks and found increased regional cerebral blood flow in various pain processing areas such as the anterior cingulate cortex, insular region, and operculum, indicating the expected but unspecific involvement of those areas in cluster headache pain processing [49]. These brain regions however are widely involved in pain processing and not specific for cluster headache. Judging from the clinical appearance with a clear circadian and circannual rhythmicity of attacks and bouts as well as a clear autonomic involvement, the hypothalamus has long been hypothesized to be crucially involved in the pathophysiology of cluster headache. Activation within this region was thus the first finding to be seen as specific for cluster headache attacks in a PET study of nine episodic cluster headache patients during nitroglycerin-triggered attacks: a small area close to the posterior hypothalamic grey matter was strongly activated during these attacks than outside of attacks [30]. This activation was present neither in mild headaches following nitroglycerin administration nor in experimentally induced pain. This led to the conclusion of this activation being indeed not solely an epiphenomenon of severe pain during cluster attacks but really cluster attack specific—a finding of tremendous importance as it was the first with a specific link to cluster headache pathophysiology. Other PET studies have been able to replicate this finding in a spontaneous acute cluster attack in one chronic cluster patient [50] and also provided evidence for reduced availability of opioidergic receptors within the hypothalamic area depending on the disease duration: the longer the disease duration, the less receptor binding was observed [38]. Additionally, hypermetabolism in many cortical and subcortical pain processing areas could be observed in cluster headache patients during “in-bout” periods but between attacks when compared to “out-of-bout” periods [51]. Interestingly, when comparing all cluster headache patients regardless of the bout status with all healthy controls, many of these areas showed hypometabolism. This undermines the hypothesis that chronic pain conditions may functionally affect pain processing areas with the cluster-specific alterations of functional increases during “in-bout” periods. PET in combination with voxel-based analyses has thus contributed essentially to our current understanding of cluster headaches and has even paved the way for specific new treatment options: stimulation of the posterior hypothalamic grey area is a treatment option in otherwise intractable cluster headache, which makes this a great example of translational medicine.
Another advantage of PET as an imaging method as compared to functional MRI is that it operates without a strong magnetic field while providing a reasonable spatial resolution, which makes it an apt tool to investigate effects and treatment mechanisms of neurostimulation therapies: this has to date been done for occipital nerve stimulation in drug-resistant chronic cluster headache [52]. After 6–30 months of stimulation, hypermetabolism within several areas of the pain matrix including the anterior cingulate cortex, the midbrain, and pons normalized, while there was still heightened activity within the hypothalamus. This might suggest a symptomatic rather than a curing effect of occipital nerve stimulation in cluster headache. To date, there are no further PET studies on neurostimulation devices in cluster headache, although this method might be ideal to study the effects of the relatively new and effective sphenopalatine ganglion stimulation.
Regarding other trigeminal autonomic cephalalgias, similar activations of the posterior hypothalamus could be demonstrated. During the acute untreated stage of paroxysmal hemicrania, there was stronger activation of the posterior hypothalamus and midbrain contralaterally to the pain site. A similar pattern could be observed for hemicrania continua with significant activation of the posterior hypothalamic grey area and the dorsal rostral pons [53]. Noteworthy, while posterior hypothalamic activation in cluster headache usually occurred ipsilaterally to the pain site, in paroxysmal hemicrania and hemicrania continua it was detected on the contralateral site.
7.3.2 Resting State Functional Magnetic Resonance Imaging
During the resting state (RS) condition, a poorly defined state in which an individual is not actively engaged in cognitive or sensory-motor tasks, the brain shows an extraordinary highly structured intrinsic dynamic activity. fMRI during RS is able to capture the low-frequency (<0.1 Hz) large-scale spatial patterns of this ongoing activity, mapping the spontaneous blood oxygen level-dependent signal covariations in the temporal domain between distant brain regions [54, 55]. The first report that RS-fMRI signal fluctuations are highly structured dates back to 1995 with the seminal work of Biswal and colleagues [54]. In this study, the authors showed that low-frequency RS-fMRI signal fluctuations in the sensory-motor regions present a high degree of correlation in the time domain. The authors argued that this temporal coherence observed between distant areas was an epiphenomenon of the functional connectivity between brain areas. Subsequent studies confirmed that several different cortical and subcortical networks present high temporal coherence of RS-fMRI signal fluctuations. This pattern of correlated activity, defined as “functional connectivity” [54, 56], seems to have its underpinning in the anatomical connectivity of the brain [57–60]: strong evidence came from studies on corpus callosum agenesis [61], and callosotomy [62, 63], neuropathological conditions that abolish, particularly in the acute state, the interhemispheric functional connectivity. However, a seminal study investigating both the functional connectivity (using RS-fMRI) and the anatomical connectivity (using diffusion tensor imaging) showed that the functional connectivity is not completely explained by the structural connectivity; indeed functional connectivity also exists between distant brain regions with no direct anatomical pathways [56]. Based on the observation that every single functional network comprises regions that are typically co-activated during the execution of a cognitive task, it was hypothesized that the task-related activity is mirrored in this intrinsic and dynamic spontaneous process [64]. At the moment, we have no clear explanations for this pervasive phenomenon [64]; however, it was speculated that this low-frequency ongoing activity might organize and coordinate neuronal activity [65] or, in a Bayesian perspective, that it may represent a dynamic prediction of the brain regions that will be involved together in the execution of tasks [66].
7.3.2.1 The Main Resting State Functional Magnetic Resonance Imaging Networks
The analyses of the spatiotemporal coherence of low-frequency fluctuations during RS-fMRI acquisitions reveal several functional networks characterized by distinct temporal coherence features [67]. These functional networks are supposed to underlie cognitive, motor, and sensory processing [68–70] and are detected mainly by means of independent component analyses (ICA) [71, 72], the seed-based approach, and the hierarchical clustering [73]. The most investigated functional circuit is the default mode network: the seminal fMRI work of Greicius et al. [74] showed that the medial prefrontal cortex, the posterior cingulate/precuneus, and the lateral parietal cortex form a very robust functional network, which increases its activity during rest and decreases its activity during the execution of tasks. This particular pattern of activity led to hypothesize that the brain presents a baseline functional state [75] whose activity is suppressed or reduced when the subject is engaged in the goal-directed behavior, although there are still some debates [76]. Beyond the default mode network, other very consistent neural functional networks, each one characterized by specific BOLD signal time-courses [71, 77], were identified. These networks comprise primary sensory networks, such as the visual [71, 78]; auditory [55] and the sensorimotor network [67]; and networks mediating several other cognitive functions, such as the temporoparietal network, the executive control network, and the salience network, particularly important in the cluster headache pathophysiology. In addition, hippocampus [79], thalamus [80], cerebellum [81], basal ganglia [81], and hypothalamic [82] networks were also identified.
7.3.3 The Main RS-fMRI Networks in Cluster Headache
Summary of the studies investigating cerebral functional connectivity in cluster headache pathophysiology using resting state functional magnetic resonance imaging (RS-fMRI) with independent component analyses (ICA)
Resting state—independent component analyses | ||||
---|---|---|---|---|
Authors | Participants | Identified networks | Between-groups differences | Other analyses |
Rocca et al. [17] | 13 episodic CH “out-of-bout” patients (8 with right-sided, 5 with left-sided attacks) | 1. Visual networks 2. Auditory network 3. Sensorimotor network 4. Frontoparietal-temporal areas 5. Frontoparietal areas L & R 6. Default mode network | CH vs. CTRL: 1. Sensorimotor network: decreased RS-FC in primary sensorimotor cortex, supplementary motor area, and anterior cingulate cortex 2. Primary visual network: decreased RS-FC in V1 | Inverse correlations between RS-FC and disease duration in: 1. Sensorimotor network (in left primary sensory-motor cortex) 2. Primary visual network (in left V1) |
Faragò et al. [33] | 17 episodic CH “out-of-bout” patients (with right-sided attacks after flipping MRI images) | 1. Visual networks 2. Auditory network 3. Sensorimotor network 4. Salience network 5. Ipsilateral and contralateral attentional network 6. Default mode network 7. Cerebellar networks | CH vs. CTRL: 1. Ipsilateral attentional network: increased RS-FC in superior frontal gyrus and middle frontal gyrus 2. Increased RS-FC in ipsilateral/contralateral cerebellar network | Inverse correlations between RS-FC and cumulative headache days in controlateral attentional network (in frontal pole) |
Chou et al. [27] | 17 episodic CH “in-bout” and “out-of-bout” (with right-sided attacks after flipping MRI images) | 1. Visual networks 2. Sensorimotor network 3. Default mode network 4. Salience network 5. Frontal attentional network 6. Dorsal attentional network 7. Parietal, cerebellar, temporal, and subcortical networks | CH vs. CTRL: RS-FC differences in several networks, in particular in DMN (in left precuneus) and in the salience network (L and R and L insula); CH “in-bout” vs. CH “out-of bout”: RS-FC differences in frontal network (R inferior frontal gyrus) and L attentional network (L postcentral gyrus) | Inverse correlations between RS-FC and disease duration in the frontal attentional network (R cingulate gyrus) during “in-bout” condition |
In the past, the investigations of neurological disorders have greatly benefited from localization-based approaches; however, the relatively recent advances in acquisition techniques, in data analyses, and in the theoretical frameworks opened new venues for the investigation of the brain activity more focused on the complexity and interactions between cerebral regions. Along these lines, RS-fMRI, investigating large-scale brain networks in the low-frequency domain, offered new and successful perspectives in various neurological and psychiatric diseases, such as Alzheimer’s disease, depression, and schizophrenia [83–88]. More importantly, alterations in the low-frequency coherence of specific networks were showed to have diagnostic and prognostic value for specific neurological diseases [89, 90]. This suggests that the more consistent RS-fMRI networks, such as the default mode network, might be sensitive biomarkers of the pathological dynamic organization of the brain.
What is the role of the investigations of the functional connectivity of the RS-fMRI networks in revealing the neuropathological bases of the cluster headache?
Cluster headache is characterized by extremely severe unilateral head pain and ipsilateral cranial-facial autonomic symptoms [91]. Clinical, neuroendocrinological, and animal findings [26, 31] together with the already mentioned neuroimaging studies of May et al. [30, 92] strongly suggest the ipsilateral (to the head side of attack) posterior hypothalamus as the generator of cluster headache attacks. These findings led to the pioneering successful treatment of refractory chronic cluster headache with hypothalamic deep brain stimulation (DBS) [93]. As the electrode tip is in fact usually located posterior to the hypothalamus in the diencephalon–mesencephalic junction, where it possibly stimulates several fasciculi and regions [94] and DBS is also successful when stimulating the ventral tegmental area [95] and the posterior wall of the third ventricle [96], the hypothalamus could have a modulatory role on some functional networks, possibly comprising the hypothalamo-trigeminal pathway [26, 97], pointing clearly to the possible presence of a dysfunctional network, normalized or modulated by DBS [26].
In line with this very important hypothesis, the study of RS-fMRI functional connectivity is very promising in the investigation of the neuropathological bases of the cluster headache condition. In this framework, since 2010, neuroimaging studies have begun to shed lights on the presence of several abnormal functional networks in the cluster headache condition: in particular, the hypothalamic network, the salience network, and the default mode network might play a key role in the cluster headache pathophysiology.
7.3.3.1 The Salience Network
The salience network is one of the most interesting functional RS-fMRI circuits in regard to cluster headache pathophysiology. The seminal work of Seeley et al. [98] showed that the areas typically involved during the execution of a variety of demanding fMRI tasks and comprising the dorsal anterior cingulate cortex, the frontoinsular cortex, the dorsolateral prefrontal cortex, and the lateral parietal cortex are dissociable into two functional circuits when appreciated with RS-fMRI: the salience network and the executive control network. The key nodes of the salience network comprise the dorsal anterior cingulate cortex and the orbital frontoinsular cortex, with projections to subcortical structures such as the thalamus, hypothalamus, and ventral tegmental area/substantia nigra. Previous works have shown that the dorsal anterior cingulate cortex and the frontoinsular cortex represent salient stimuli, such as hunger [99] and pain [100], and respond to emotional pain, such as during social rejection; it was therefore hypothesized that these areas are the neural substrate of the interoceptive feedbacks [101]. In agreement, Seeley et al. [98] proposed that the salience network identifies relevant homeostatic stimuli, by integrating sensory information with visceral and autonomic functions, supporting a capital role of this network in pain processing. Notably, the identified salience network comprises brain regions involved in the central processing of the autonomic functions [102]. Indeed, the anterior cingulate cortex, the insular cortex, the amygdala, and the hypothalamus were shown to be key components of the autonomic function network. It is important to note that the parasympathetic and the sympathetic system present divergent central processing pathways: the parasympathetic system maps onto areas of the default mode network, while the sympathetic system maps onto areas of the salience and the executive network [102]. The possible involvement of the salience network in the cluster headache pathophysiology is well suggested by its clinical features and neuroimaging investigations. The typical clinical features of this condition (severe head pain and cranial-facial autonomic symptoms) directly call in cause areas involved in pain and in central autonomic processing; neuroimaging investigations provided convincing evidence of the involvement of the hypothalamus, the anterior cingulate cortex, and the insular cortex, areas belonging to the salience network, during spontaneous and induced cluster headache attacks [18, 23, 24, 50, 103]. Similarly, RS-fMRI studies also reported abnormal functional connectivity between these same cortical regions (i.e., anterior cingulate cortex and insular cortex) and the hypothalamus in “out-of-bout” conditions [17] and during CH attacks [104]. Based on these pieces of evidence, Qiu et al. [105] directly investigated the functional connectivity of the salience network in a relatively large sample of episodic cluster headache patients during the “in-bout” condition but outside the attacks. The results of this study suggest that episodic cluster headache patients present, in comparison to healthy individuals, a decreased functional connectivity between regions of the salience network and the bilateral hypothalamus, independent from the site of recurrent attacks. The authors suggested that the presence of a defective functional connectivity within the salience network might indicate an abnormal pain control, with possible dysregulation of the antinociceptive pathways, leading to the generation of the cluster headache attacks. Functional alterations of the salience network were replicated in a more recent study [19] showing a decreased functional connectivity in the insular cortex within this network in cluster headache patients during “in-bout” and “out-of bout” conditions. This last observation seems to indicate that the functional alterations in the salience network are relatively stable and not related to the shift from “in-bout” to “out-of bout” condition and vice versa.
7.3.3.2 The Default Mode Network
The default mode network comprises the ventral medial prefrontal cortex, the dorsomedial prefrontal cortex, the posterior cingulate cortex, the precuneus, and the lateral parietal cortex [76]. The entorhinal cortex is frequently described as a structure belonging to this functional network. According to Raichle [76], the default mode network integrates the sensory-visceromotor processing (occurring in the ventral medial prefrontal cortex), with the self-referential activity (occurring in the medial prefrontal cortex) and the recalling of the previous experience (occurring in the precuneus/parietal cortex and in the hippocampus). Importantly in the context of the cluster headache, as we have noted above, the central processing of the parasympathetic activity occurs in the default mode network [102]. Given the above characteristics, it is not unexpected that default mode network presents abnormal functional connectivity in chronic pain conditions [106–108] and in several neuropsychiatric [77] and neurodegenerative [74] diseases. Notably, the dysfunctional connectivity of default mode network in the context of chronic pain processing seems to mediate pain rumination [109]. Based on these observations, it is plausible that the cluster headache, as recurrent (chronic) and severe pain, may induce alterations of the default mode network functional connectivity. Rocca et al. [17], investigating the intrinsic functional connectivity in episodic cluster headache patients during the out-of-bout condition, reported no alterations in the default mode network. This lack of evidence was possibly related to the relatively small sample size investigated. Indeed, in a larger dataset of cluster headache patients, Chou et al. [19] observed functional alterations of the default mode network (in left precuneus) in both the “in-bout” and “out-of-bout” conditions with no differences between the two. As for the salience network, this work suggests that the default mode network is dysfunctional in episodic cluster headache patients; however, this dysfunction is not affected differently by the “in-bout” and “out-of-bout” conditions, indicating relatively stable functional alterations in this network.
7.3.3.3 Hypothalamic RS-fMRI Functional Connectivity
Summary of the studies investigating hypothalamic functional connectivity in cluster headache pathophysiology using resting state functional magnetic resonance imaging (RS-fMRI) with seed-based analyses (seed in left or right hypothalamus, or * in hypothalamus ipsilateral to the pain or controlateral to the pain)
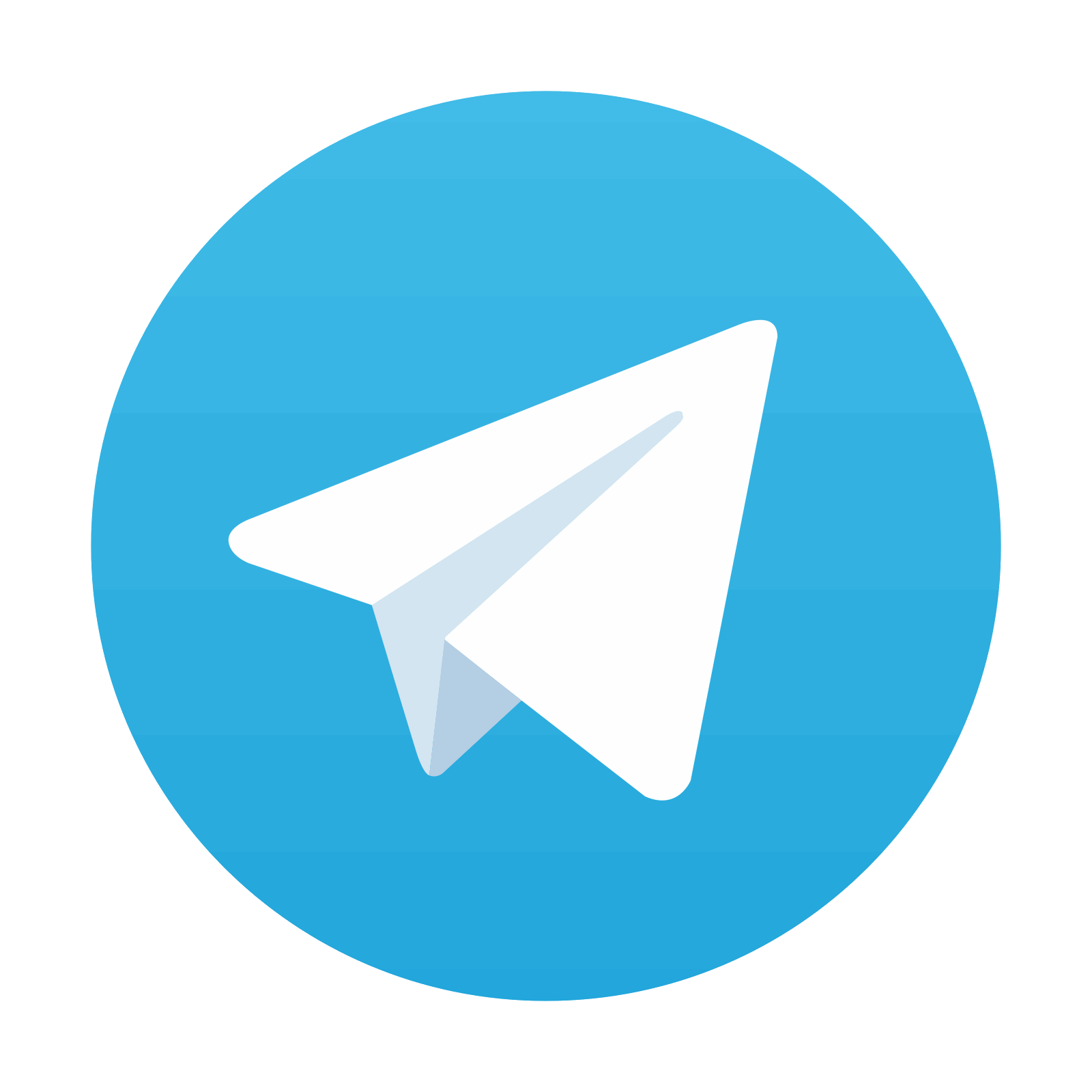
Stay updated, free articles. Join our Telegram channel

Full access? Get Clinical Tree
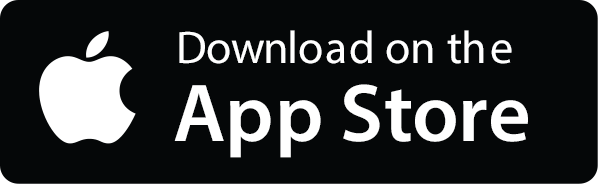
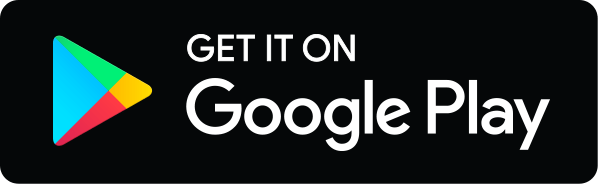
