Inflammation and Immunity
Up to this point, we have discussed a number of mechanisms that may explain how the mentally ill brain is different from the normal brain. The immune system is increasingly being viewed as a possible culprit. The body’s reaction to foreign invasion (or mistaken foreign invasion) and the resulting inflammatory response may contribute to the development of some psychiatric disorders.
For a long time, there have been indications of subtle links between mental illness and the immune system. The first antidepressants were developed in the 1950s when it was noted that the antitubercular medication iproniazid had a positive effect on mood. When treated with this medication, even some terminally ill patients became more cheerful, physically active, and hopeful. Ultimately this antibiotic was refined and transformed into the first effective antidepressant: the monoamine oxidase inhibitors.
In 1929 the Swiss psychiatrist Tramer reported an increase in schizophrenic births during the months from December to May. Tramer noted that children born during the winter/spring months were more likely to develop schizophrenia as adults. It is postulated that the seasonal fluctuation is influenced by exposure to infections during the perinatal period, which are more common during the winter months—more on this topic later in the chapter.
Perhaps the oldest link between infection and psychiatric disorders lies with syphilis. This microbe was the cause of about 20% of the hospitalizations for mental illness at the beginning of the last century. In 1910 it was Paul Ehrlich’s “magic bullet,” compound 606, that became the first effective medication for syphilis—the beginning of psychopharmacology. The microbe that causes syphilis, Treponema pallidum, provides a good example of the immune response and the problems this response causes.
THE IMMUNE RESPONSE
The body’s immune system is poised to recognize and attack foreign invaders—anything that is nonself: splinters, bacteria, Fox News, etc. The attack is conducted by a confusing array of cells and proteins—some of which stand guard at all times, while others remain dormant until the alarm is sounded. All the components interact with each other through a litany of signals to rally the defenses when a microscopic terrorist is identified. Likewise the defenses must be turned off when the coast is clear. For the purposes of this book, we can simplify the immune response down to four major components:
Cellular—white blood cells (leukocytes)
Humoral—antibodies
Complement—little torpedoes
Cytokines—the molecular signals
All four of these components come into play when the syphilitic bacteria T. pallidum invades the body.
Syphilis
Syphilis is a sexually transmitted disease caused by the corkscrew shaped bacteria T. pallidum (Figure 9.1). When a contagious individual has sex with an uninfected partner, there is about a 30% chance the disease will be transferred. The body’s first line of defense against infection is the skin. T. pallidum spirochete penetrates the dermal barrier by twisting through microabrasion.
The body’s next line of defense is the complement system (Figure 9.2A). The complement system is made up of numerous proteins circulating in the blood poised to coalesce into little torpedoes. When activated by foreign proteins, such as those found on bacteria, the complement molecules lock on to the bacteria. As more and more of these
proteins accumulate on the bacteria, the complement can literally drill a hole in the cell wall. This allows fluid to pore in and the bacteria explodes. The trick is to limit the attack to the foreign invaders—to avoid attacking host cells.
proteins accumulate on the bacteria, the complement can literally drill a hole in the cell wall. This allows fluid to pore in and the bacteria explodes. The trick is to limit the attack to the foreign invaders—to avoid attacking host cells.
Also standing ready to fend off foreign invaders are a variety of white blood cells (leukocytes). Two of these, the macrophage and the neutrophil, are frontline defenders. The macrophage patrols the perimeter of the skin and mucus membranes cleaning up debris and searching for bad guys. (They also are one of the cells that will present foreign antigens to T cells to start the production of antibodies.) Neutrophils circulate in the blood ready to come to the aid of defenseless tissue. They aggressively attack T. pallidum but generally also make a mess of the healthy tissue. The initial physical sign of syphilis—the chancre that develops on the genitalia at the site of the infection—is in large part due to neutrophils. Pus is predominantly dead neutrophils—suicide bombers at the cellular level.
Syphilis Continued
After the first phases of syphilis, most people enter a latency phase—usually within 6 months. During this phase, they do not have signs or symptoms of the illness and are not contagious. However, microbes remain hidden within the body and laboratory studies are still positive. Remarkably, the T. pallidum can quietly live undetected in the host for years. How is this possible?
The best explanation for the stealth capabilities of T. pallidum is the relative inertness of the outer membrane. The proteins that protrude through the phospholipid bilayer cell membrane wall (called integral proteins) are few and far between in T. pallidum. It is these proteins that killer T cells and antibodies grab when they attack the bacteria. In comparison, T. pallidum has only 1% of the integral proteins compared with Escherichia coli. So, this bacteria can remain hidden, under the radar so to speak, and not be recognized as different—like little terrorist sleeper cells.
If left untreated, approximately one-third of the people infected with syphilis will cure themselves, another third will remain in the latency phase until they die, and the last third will develop tertiary syphilis—the most serious phase of the infection. Of particular interest for us is neurosyphilis. Neurosyphilis includes inflammation of the meninges, cerebral vasculature, or neural tissue of the brain and/or spinal cord. Although animal studies have shown that T. pallidum can find their way to the central nervous system (CNS) within 18 hours after the initial infection, the serious manifestations of neurosyphilis do not develop for 10 to 50 years.
The important point is this: once the immune system catches up with syphilis in the CNS, it is the inflammatory response—not the bacteria per se—that damages the brain. T. pallidum does not produce a toxin nor directly attacks the cells of the nervous system. It is the overexuberant cellular response to T. pallidum that blocks the vascular support and kills the neurons. The military calls this collateral damage. The incessant neural loss once the immune system is activated leads to personality changes, psychosis, and dementia as well as paralysis, seizures, and death.
TREATMENT COCAINE VACCINE
Cocaine dependence is a major health problem. There is no Food and Drug Administration (FDA)-approved pharmacotherapy. One research group has developed a vaccine that stimulates the immune system to produce anticocaine antibodies. These antibodies can sequester circulating cocaine and prevent the substance from entering the brain and quelling any euphoria. Early studies have found reduced cocaine intake for addicts whose body produced sufficient antibodies. Future research is focused on eliciting a stronger and more sustained immune response in more patients.
Inflammation
The inflammatory response is the body’s overall reaction to foreign invasion. The signs are swelling, redness, heat, and pain. The mechanisms that cause the inflammation are engorgement of the blood vessels, movement of fluid into the tissue, and, above all, attraction of leukocytes to the infected area. The signals that start the inflammatory process and coordinate the response are the cytokines. (More on the cytokines later in the chapter.)
Adaptive Immunity
A large part of the success of the mammalian defense system results from the capacity of the system to proliferate and attack specific and unique molecular features of the invader. This adaptive immunity commences when pieces of the destroyed bacteria are taken to the lymph nodes and presented to T cells—essentially shopped around to find a good match (Figure 9.2B). When a close match is found, the T cells multiply and head off to attack that specific remnant of the invader, but not before activating the B cells. The B cells with a similar match will start producing antibodies. Antibodies are the “Y”-shaped proteins that can lock on to the specific foreign molecules of the bacteria, virus, or infected cell. Antibodies attached to a foreign cell serve as a signal to other leukocytes, essentially saying that this cell is ready for demolition (Figure 9.2C).
The important points to grasp are the varied mechanisms the body uses to defend against foreign invasion and the need for numerous checks and balances to keep the response under control, that is, to avoid damaging the host cells. As with any battle, “civilian casualties” are unavoidable. War, even at the microscopic level, is a dirty business.
IMMUNITY IN THE BRAIN
The immune response inside the brain is less aggressive than the response normally seen in the rest of the body. Constraining the response is essential because the rejuvenating capacity of the brain is limited and the effects of collateral damage from inflammation are more serious. White blood cells are rarely seen in the CNS. But the brain is not defenseless. Although once thought to be “immune
privileged”—that is, able to tolerate the presence of an antigen without eliciting an inflammatory response—the brain is now recognized to have a unique but muted immune response to invaders.
privileged”—that is, able to tolerate the presence of an antigen without eliciting an inflammatory response—the brain is now recognized to have a unique but muted immune response to invaders.
The brain has three layers of protection against foreign invasion.
The blood-brain barrier—physical barrier to isolate the brain.
Microglia—the macrophage of the brain.
Leukocytes infiltration reserved for serious damage.
The distinguishing feature of the CNS immune system is the microglia. The microglia, although considered a nerve cell, actually originate from a different cell line. While neurons, oligodendrocytes, and astrocytes develop from cells of neuroectodermal origin, the microglia come from mesenchymal stem cells (bone, cartilage, and blood cells). Microglia move in and take up residence among the neural cells very early during embryonic development.
The microglia are most closely related to the macrophage and are the central component of the brain’s innate immune system. Like the macrophage, they are dormant until activated, can migrate to the battle zone, and attempt to gobble up whatever should not be there. The microglia respond to chemical signals of danger, emit pro-inflammatory cytokines, and can function as antigen presenting cells—similar to the macrophage. In addition, microglia can also release cytotoxic substances such as hydrogen peroxide to damage the invader. Unfortunately, the hydrogen peroxide also kills neurons.
Neural Prostheses
The protagonist of the 1970s television series The Six-Million Dollar Man was a NASA astronaut whose injured body was rebuilt with mechanical parts. Oh, if only it was this easy. Forty years later, real researchers are still struggling with the basics. A device that could restore simple movements for people with spinal cord injuries would be of great value to those confined to a wheelchair. While still very much in the experimental stages, the goal is to capture the individual’s neural signals generated in the motor cortex and turn them into useful movements (Figure 9.3).
Electrodes implanted directly into the gray matter of the motor cortex are an intrusive element needed to determine the person’s intentions. The electrode captures the electrical activity as the neurons generate action potentials and the signals are sent to a computer. The computer—after substantial training—decodes the signals and moves the external device—in this case a mechanical arm. Problems develop as the brain’s immune system produces a sustained reaction against the foreign body electrode.
Indwelling Electrodes
The gray matter of the cortex is dense with blood vessels. Invariably as the electrodes are pushed into the brain, the vasculature is ruptured. This breaches the blood-brain barrier and allows the entry of inflammatory proteins as well as macrophages, neutrophils, and T cells. In short, the wound healing process is initiated and neurons—the ones the electrode needs to record—can be damaged or destroyed. While the bloodborne inflammatory response is the most serious, microglia and astrocytes become activated regardless of the vascular damage (Figure 9.4).
Microglia, in their resting state, look like stars with elaborate branching structure. When they sense trouble, they multiply and morph into rounder structures. They also increase their production of cytokines and growth factor proteins. In this activated state, they engulf and digest the cellular debris generated by the injury—acting like their macrophage cousins from the periphery.
Astrocytes usually support the neurons and maintain the integrity of the blood-brain barrier. In response to injury they too become activated. Their main function in response to trouble is to isolate the problem. They produce and release a fibrous substance that plays an essential role in forming a physical barrier around the injury. This barrier is commonly called the glial scar. This is a problem for the whole contraption, since the scar impedes contact between neurons and the electrode.
Glial Scar
Reactive gliosis, the formation of the glial scar, helps to isolate the injury, prevent overwhelming inflammation, and limit cellular degradation. The importance of gliosis was established in mice whose astrocytes were depleted. Small stab wounds in their spinal cords resulted in greater blood-brain barrier disruption, larger leukocyte infiltration, increased neural loss, and more motor deficits than comparable mice with normal astrocytes.
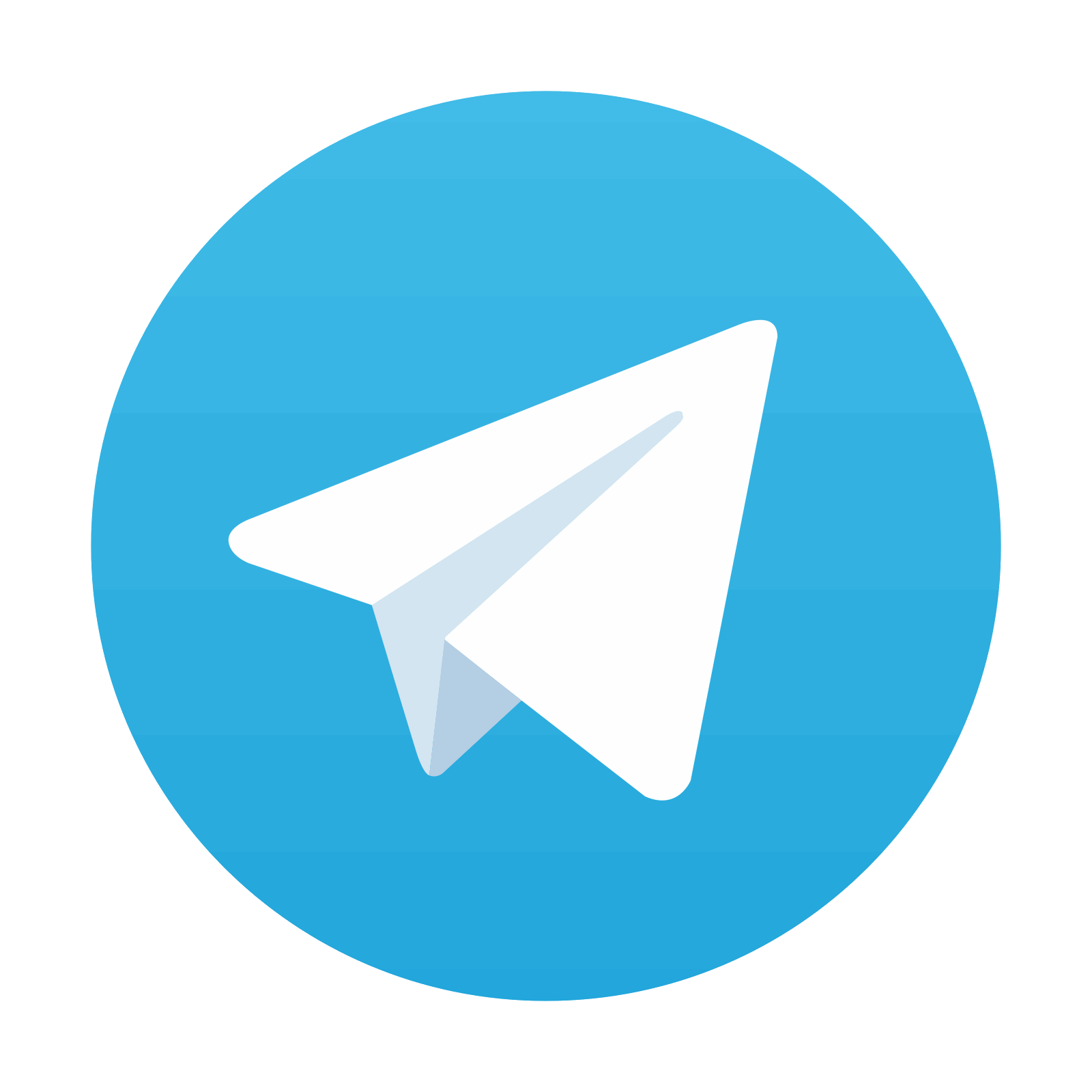
Stay updated, free articles. Join our Telegram channel

Full access? Get Clinical Tree
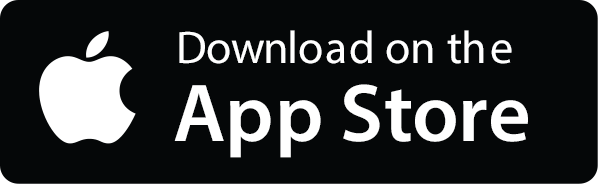
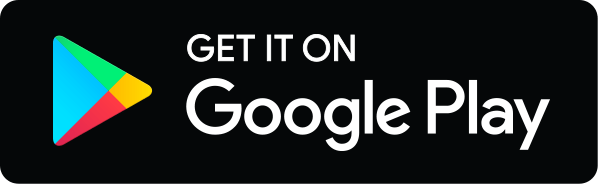
